Abstract
Chemical utilization of CO2 is one of the fundamental strategies to synthesize value-added chemicals and reduce the CO2 emission. N-formyl-1,2,3,4-tetrahydroisoquinoline derivatives (FTHIQs) are important chemicals for the synthesis of medical intermediates and alkaloids. N-formylation of isoquinoline derivatives (IQs) with CO2 is an ideal protocol to synthesize the FTHIQs and utilize the CO2 source. In the present work, we prepared a heterogeneous Ru/ZIF-8 catalyst with a Ru loading of 2 wt% by a simple impregnation method and assessed its performances in the N-formylation of IQs and CO2 with H2. The Ru/ZIF-8 catalyst offered high IQ conversion of 98% and FTHIQ selectivity of 93% in the reaction under relatively mild conditions (150 °C, 2 MPa CO2, and 6 MPa H2). The catalyst possessed good universality and reusability, showing a potential in wide application of N-formylation of highly unsaturated nitrogen-containing isoquinolines.
1. Introduction
CO2 is a greenhouse gas, and its high concentration in the atmosphere causes many climate problems [Citation1–3]. Conversion of CO2 into value-added chemicals represents a green way to utilize this waste C1 source and further approach net-zero carbon emission. Great efforts and significant progresses have been devoted into this issue especially in the last decade. Particularly, diversified important chemicals such as hydrocarbons, olefins, alcohols, acids, and amides have been efficiently synthesized [Citation4–12]. Among these products, amides are one kind of important fine chemicals and pharmaceutical intermediates [Citation13]. To efficiently synthesize amides is highly important and has caught much attentions.
N-formylation reaction can convert amines to amides especially formamides, which has been widely studied in both academia and industry. By using different C1 sources, the N-formylation reaction could be achieved. In addition to CO2, other C1 sources are also applied in the reaction. For example, by using HCOOH as C1 and hydrogen sources, Ma et al. used a KCC-1@Co-N-C-800 catalyst for the N-formylation of isoquinolines to N-formyl-1,2,3,4-tetrahydrogenisoquinolines (FTHIQ) reached 71.8% of conversion and 99.9% of selectivity, respectively [Citation14]. An inorganic-ligand loaded Fe catalyst also showed high activity for the reaction of amine with formic acid to formamides [Citation15]. CH3OH could be also used as C1 source for the reaction. In Han and coworkers’ work, a (NH4)3[CrMo6O18(OH)6] catalyst could catalyse the N-formylation of amines with methanol in the presence of H2O2 [Citation16]. (CH2O)n could be used as C1 source and converted into formamides over the catalysts like Au/Al2O3 with high yields [Citation17]. However, among these C1 sources, CO2 possesses the properties of abundance, low price, and low toxicity, and is an ideal C1 source for the reaction.
Generally, N-formylation of amine with CO2 is one of the sustainable ways to generate amides because the reaction not only provides different classes of amides products, but also consumes the waste CO2 source. In fact, lots of catalysts have been developed to achieve the reaction, for example, the homogeneous catalysts involving Ru [Citation18,Citation19], Pd [Citation20], Co [Citation21], Fe [Citation22], and the heterogeneous catalysts containing Pd/Al2O3-NR-RD (NR and RD mean nano-rod and reductive deposition) [Citation23], Pd/Mg-Al LDH [Citation24], Pd/PAL (PAL means natural palygorskite) [Citation20], and other supported Pd-based [Citation25] or Pd-Au bimetallic catalysts [Citation26], were established with outstanding performances. However, most of the developed catalysts mainly focused on the reaction of the saturated amines as the substrates. The N-formylation of unsaturated amines, especially for isoquinoline derivatives, is still challenging.
N-formyl-1,2,3,4-tetrahydrogenisoquinolines (FTHIQs) are important amides in organic synthesis and pharmaceutical industry [Citation27]. Indeed, N-formylation of amines with CO2 and other C1 sources have drawn extensive attention for the direct transformation of amine to formylamines [Citation28–32]. For instance, He and co-workers developed the direct N-formylation of isoquinolines (IQs) with CO2 and H2 over a heterogeneous Co catalyst combined with ZnCl2 at 170 °C [Citation27]. In the catalytic system, the homogeneous ZnCl2 was needed, and this was unfavourable for the separation of products from the reaction.
Thus, to develop a totally heterogeneous catalyst in N-formylation of isoquinolines with CO2 and H2 is highly important. Great efforts have been made to develop transition-metal-based heterogeneous catalysts. Herein, we prepared a simple Ru/ZIF-8 catalyst for the N-formylation of IQs and CO2 with H2 to FTHIQs. Especially, the Ru/ZIF-8 catalyst with Ru loading of 2 wt% could achieve the reaction with the conversion of IQ and the selectivity to FTHIQ approaching 99% and 93%, respectively under 150 °C, CO2 2 MPa, and H2 6 MPa. Besides, the N-formylation of IQs was found to be proceeded via the coupling of selective hydrogenation of IQs and N-formylation of N-tetrahydrogenisoquinolines with CO2 and H2. CO2 was proposed to directly participate the reaction but not via an intermediate of CO, CH3OH, (HCHO)n, or HCOOH, etc. Additionally, the catalyst possesses acceptable universality in the reaction, and could be reused at least for four times without obvious loss in activity.
2. Experimental section
2.1. Materials and methods
IQs (99%), indole (98.0%), phenylacetonitrile (99%), and 3-methylisoquinoline (95%) were obtained from Macklin. Zn(OAc)2·6H2O (99.0%) was afforded by Tianjin Kermel Chemical Reagent Co., Ltd. RuCl3 (99.0%), 1,2,3,4-tetrahydroisoquinoline (THIQs, 98.0%), and 5-methoxyisoquinoline (97.0%) were provided by Aladdin. 2-Methylimidazole (99%) was purchased from J&K Chemical Ltd. N2 (99.999%), CO2 (99.995%), and H2 (99.99%) were purchased from Xi’an Teda Cryogenic Equipment Co., Ltd. All other chemicals were obtained from commercial companies and used without further purification.
2.2. Preparation of ZIF-8
Typically, Zn(OAc)2·2H2O (2.195 g, 10 mmol) and 2-methylimidazole (8.200 g, 100 mmol) were dissolved in 15 mL of ethanol under stirring, which was then transferred into a 25 mL Teflon-lined stainless steel autoclave. Subsequently, the autoclave was placed in an oven and heated to 120 °C and maintained for 24 h. After that, the autoclave was rapidly cooled to 0 °C in an ice bath. A light yellow-colored solid was obtained after washing by CH3OH and H2O for three times.
2.3. Preparation of the Ru/ZIF-8 catalyst
The Ru/ZIF-8 catalyst was prepared by an impregnation method, and the procedures are given in . RuCl3·3H2O (25 mg, 0.1 mmol) was dissolved in 2 mL of ethanol under stirring to form a homogeneous solvent, which was then slowly dropped into 1.0 g above prepared ZIF-8 support. After 3 h, the ethanol solvent was removed at 50 °C and the catalyst was further dried at 150 °C overnight under vacuum. The obtained catalyst was denoted as the Ru/ZIF-8 catalyst. Other catalysts with different Ru loadings, loaded metals (Pd, Rh, Co, Cu, etc.), and supports (SiO2, TiO2, Al2O3, MgO, etc.) were synthesized by the same procedures.
2.4. Catalyst characterization
The metal loadings were analysed by ICP-OES. The TEM images were studied on a JEM-2100 electron microscope with an acceleration voltage of 200 kV. The XRD tests were performed on a Rigaku D/max 2500 equipped with a nickel filtered Cu-Kα (λ = 0.154 nm) radiation and operated at 40 kV and 20 mA. The XPS spectra were studied using an ESCALab 220I-XL electron spectrometer from VG Scientific using 300 W Al Kα radiation with a hemispherical energy analyser. Raman spectra of the prepared catalysts were recorded on a Laser microscopic Raman imaging spectrometer (Thermo Fisher, USA) equipped with a 532 nm Ne laser and a high-grade Leica microscope. The textural and structural properties of the catalysts were tested by N2-adsorption/desorption measurements, which were carried out on an ASAP 2460 (Micromeritics).
2.5. N-Formylation of isoquinoline derivatives with CO2 and H2
The N-formylation reaction was carried out in 16 mL of stainless autoclave with a Teflon inner container [Citation33]. In a typical reaction, isoquinoline (0.5 mmol), catalyst 25 mg, and THF (1 mL) were added into the reactor, which was then sealed and flushed with 1 MPa H2 to remove the air for three times. Afterwards, the autoclave was charged with 2 MPa CO2 and 6 MPa H2 and heated to 150 °C and stirred for 15 h. Upon reaction completion, the autoclave was cooled to room temperature and vented slowly. In addition, the solid catalyst was separated and the liquid was analysed by GC (GC9720, Zhejiang Fuli Analytical Instruments Co., Ltd., China) equipped with an HP-5 capillary column (30 m × 0.32 mm × 0.25 μm) by using toluene as an internal standard and GC-MS (Agilent 6890 N-5975) with an HP-5MS capillary column (30 m × 0.32 mm × 0.25 μm).
2.6. The reusability of the catalyst and the hot filtration experiments
Upon reaction completion, the solid Ru/ZIF-8 catalyst was separated and washed by 3 mL of THF for 5 times, as well as dried in vacuum at 50 °C, which was then used in the next run. The details for the hot filtration experiment were the same as the typical procedures mentioned above except that the catalyst was separated after 9 h of the reaction. The liquid continued to be reacted in the absence of a catalyst for other 6 h. After the reaction, the liquid was analysed by GC to discover whether the FTHIQ has increased.
3. Results and discussion
3.1. Catalyst characterization
The prepared catalysts were characterized by various technologies. Initially, the morphology of the catalyst was investigated by TEM, and the results are given in . The fresh catalyst showed a morphology of ZIF-8 NPs with the size in the ranges of 30-60 nm (). The particles with a mean size of 5.2 nm appeared in the ZIF-8 NPs were indexed to ZnO (101) for the lattice spacing of 0.247 nm (), showing the ZnO nanoparticles are formed on the ZIF-8 supports. It should be mentioned that ZnO could be in-situ formed when zinc carbonate or znic acetate as the metal precursors for the preparation of ZIF-8. Additionally, part of 2-methylimidazole ligands coordinate with Zn2+ to form ZIF-8, while the unreacted 2-methylimidazole ligands acted as catalysts for the formation of ZnO nanoparticles [Citation34]. The used catalyst was also investigated by the TEM tests, and the images show that it is aggregated (). Besides, the HRTEM images show the presence of nanoparticles with the lattice spacing of 0.203 nm (), which could be assigned to the (101) plane of Ru0. The results indicated that the Ru species were reduced to Ru0 after used 5 times.
Figure 2. TEM and HRTEM of the fresh Ru/ZIF-8 catalysts (a, b, and c) and the catalyst used after 5 times (d, e, and f).
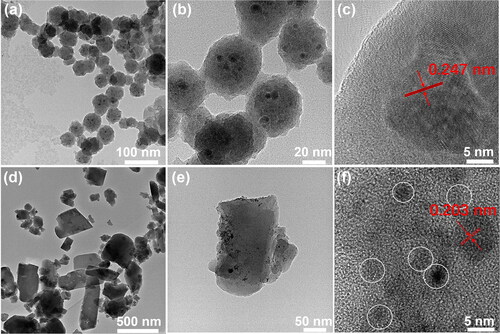
The textural structures of the ZIF-8 support and the Ru/ZIF-8 catalyst were characterized by N2-adsorption/desorption isotherms, and the results are given in . The specific surface area and the pore volume of the ZIF-8 are 1687 m2/g and 0.82 cm3/g, and these values for the Ru/ZIF-8 catalyst decreased to 1187 m2/g and 0.60 cm3/g, respectively. The high N2 adsorption amounts at low P/P0 ranges close to zero indicated that the ZIF-8 support possesses abundant micro porous, and these pores still exist in the Ru/ZIF-8 catalysts. The abundant micro pores on the Ru/ZIF-8 catalyst are beneficial for the mass transformation in the direct N-formylation. The incorporation of Ru species into the pores could account for the decrease in BET surface areas of the Ru/ZIF-8 catalyst. The other catalysts showed low BET surface areas, for example, the Ru/SiO2, Ru/TiO2, and Ru/Al2O3 catalysts exhibited 34.6, 162.2, and 204.1 m2/g, respectively.
Figure 3. N2 adsorption/desorption isotherms of the ZIF-8 support, the 2 wt% Ru/ZIF-8, 2 wt% Ru/SiO2, 2 wt% Ru/TiO2, and 2 wt% Ru/Al2O3 catalysts.
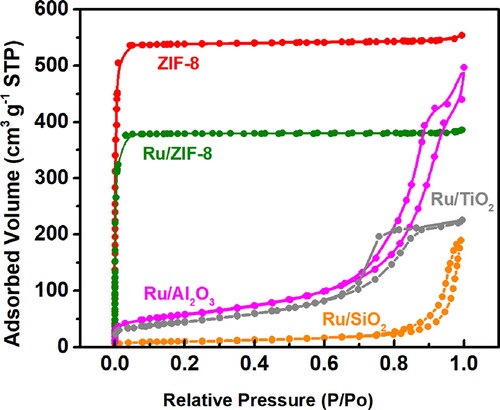
Raman spectra of the ZIF-8, the fresh and the used Ru/ZIF-8 catalysts were investigated, with the results given in . The peaks at 182 cm−1 and 282 cm−1 for the ZIF-8 could be assigned to the Zn-N bonds, and the other peaks at 678, 1143, 1160 and 1489 cm−1 with high intensities are contributed to the imidazolium ring, C–N stretching, and C–H stretching, respectively [Citation35]. After loading of Ru species, the Raman spectra of the fresh catalyst are the same as that of the ZIF-8, indicating that upon impregnation of Ru species, the structure of the ZIF-8 was not changed. The used catalyst shows a relatively low intensity, but the Raman shifts are the same as those of the ZIF-8 and the fresh catalyst.
Figure 4. Raman spectra of the ZIF-8, the fresh 2 wt% Ru/ZIF-8, and the catalyst after used 5 times, respectively.
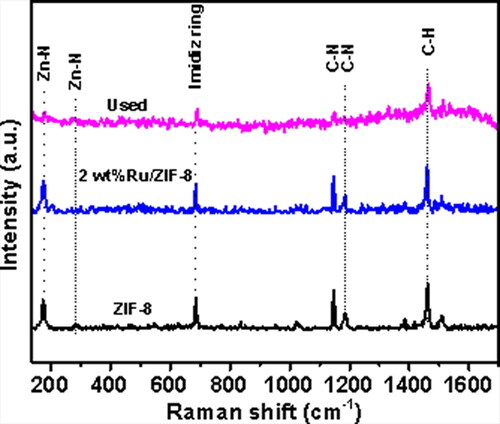
To detect the bulk phase information of the prepared samples, the XRD patterns were studied, and the results are given in . The ZIF-8 support showed the peaks at 7.3, 10.2, 12.7, and 18.5° assigned to the (011), (002), (112), and (222) planes of ZIF-8, respectively, implying a body-centred cubic crystal lattice of the space group I—43 m [Citation36]. The XRD pattern of the fresh Ru/ZIF-8 catalyst was the same as that of the support, and no peaks corresponding to Ru species were found for the low loadings. The XRD pattern of the used catalyst shows a similar profile to the fresh catalyst, and does not show any Ru0 species, either.
Figure 5. XRD patterns of the ZIF-8, the 1 wt% and 2 wt% Ru/ZIF-8 catalysts, and the catalyst after used 5 times, respectively.
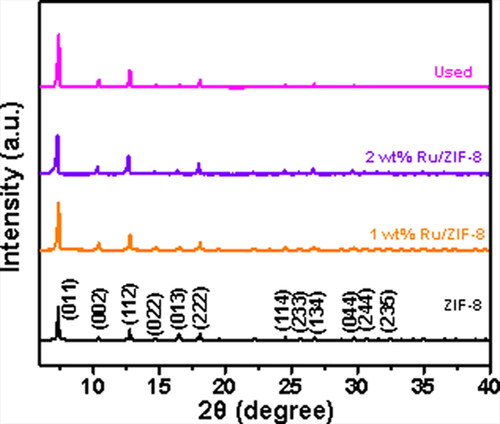
The catalyst was also characterized by FT-IR, and the results are shown in . The peaks at 2936, 1601, 1145, 998, and 421 cm−1 could be assigned to the absorption peaks of C-H, C = N, C-N, and Zn-N bonds in imidazole, respectively [Citation37]. The FT-IR spectra of the Ru/ZIF-8, the fresh 1 wt% Ru/ZIF-8, the 2 wt% Ru/ZIF-8, and the used catalyst are mainly consistent with the characteristic curve of the ZIF-8, indicating that the catalyst, especially the ZIF-8 support is not destroyed during its preparation and reaction processes, which is consistent with the XRD results.
3.2. Catalytic performances
As mentioned above, the catalytic activity of the catalyst in the N-formylation reaction of isoquinoline with CO2 and H2 was investigated, and the results are shown in . No product was detected in the absence of a catalyst, confirming the reaction could not occur spontaneously (, entry 1). When a single ZIF-8 was used as the catalyst, the reaction could not be proceeded, either (, entry 2). However, the Ru/ZIF-8 catalyst with 2.0 wt% Ru loading showed a very high acticity for the reaciton, offering 99% conversion of IQ and 94% selectivity to FTHIQ. Only a small amount of THIQ was detected (5%), indicating the catalyst has a proper hydrogenation capacity, so as to avoid the occurrence of over-hydrogenation reaction (, entry 3). For comparison, diverse precious metals (Pd, Rh) and precious metal-free catalysts (Co, Cu) supported on the ZIF-8 were investigated for the titled reaction (, entries 4-7). Compared with precious metal-fee catalysts such as Co and Cu, noble metal catalysts have higher conversions of IQ. However, all the compared catalysts were less active in N-formylation. We also stuided the catalytic performances of Ru supported on other supports, such as SiO2, TiO2, Al2O3, and MgO. However, all these samples offered a low activity for the synthesis of FTHIQ, indicating that they are less active for the N-formylation. The activity order for the Ru/SiO2, Ru/TiO2, and Ru/Al2O3 catalysts was inconsistent with the order of their BET surface areas, indicating that the mass transfer was propably not the key reason for the different catalytic performances. The performances among the Ru/ZIF-8 catalyst and Ru supported on others supports confirmed that there is an interplay between the Ru metal and the ZIF-8 support.
Table 1. Screening of catalysts in N-formylation of IQ and CO2 with H2.a
To further exam the Ru role in the reaction, the effect of Ru loadings was also studied. The 0.5 wt%Ru/ZIF-8 catalyst offered a low conversion, while the Ru/ZIF-8 catalysts with higher Ru loadings including 1 wt% and 1.5 wt% showed high activities in hydrogenation of IQ, but low activities in the N-formation. The results indicated that the N-formalytion of IQ involves two steps, i.e. the hydrogenation step and N-formylation step. The active Ru component not only affects the hydrogenation activity, but also has a great influence on the N-formylation via the interplay between Ru and ZIF-8 support.
By using the 2 wt% Ru/ZIF-8 catalyst, we studied the effects of reaction conditions on catalytic performances of N-formylation of isoquinoline with CO2 and H2, and the results are given in . The reaction temperature affected the catalytic performance remarkably, and the target FTHIQ was less yielded at 120 °C. Increasing the reaction temperature could enhance the catalytic activity, and the highest yield was obtained at 160 °C (). The effect of reaction pressure (PCO2/PH2) was also investigated by setting CO2 pressure at 2 MPa. A low (4 MPa) or high pressures of H2 (>8 MPa) were not beneficial for the reaction, and the best performances were proceeded under 6 MPa H2, which was probably because the low PH2 can not meet the demand for the hydrogenation of the highly unsaturated isoquinoline, while the too high pressure of H2 may cause over-hydrogenation reactions, such as hydrogenation of THIQ, hydrogenation of FTHIQ, etc (). Besides, the effect of reaction time on the catalytic performances was investigated (). The THIQ and FTHIQ could be produced at the first 6 h but with low yields. With prolonging the time to 9 h, the yields of THIQ and FTHIQ increased remarkably. However, further prolonging the time caused the decrease in the yield of THIQ but an increase in the yield of FTHIQ, confirming that FTHIQ is obtained from THIQ. The effect of the agitation speed on the catalytic performances is shown in , the catalytic performances could be achieved under 200 rpm. Hence, the subsequent studies were carried out under 200 rpm.
Figure 7. Effects of temperature (a), pressures of CO2 and H2 (b), the reaction time (c), and the agitation speed (d) on the catalytic performances of N-formylation of isoquinoline and CO2 with H2 over the Ru/ZIF-8 catalyst. Reaction conditions: catalyst 25 mg, THF 1 mL, and isoquinoline 0.5 mmol.
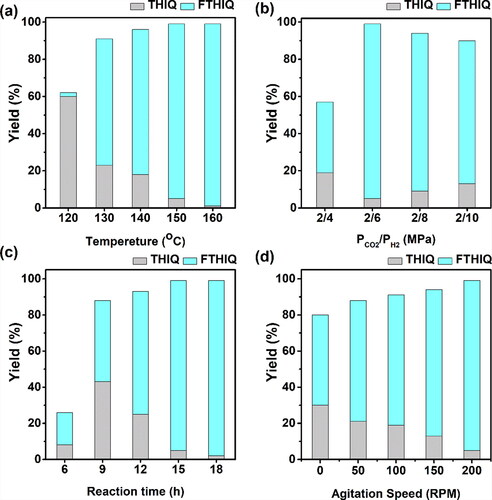
As mentioned above, the reaction was proceeded via a cascade way, i.e. the coupling hydrogenation and N-formylation reactions. To further confirm this, we carried out some control experiments in Scheme 1. The reaction could not be proceeded in the absence of H2, indicating that hydrogenation step was the trigger for the whole reaction and H2 is the real hydrogen source. IQ could be hydrogenated in the absence of CO2, which produced THIQ with a yield of greater than 99% but no FTHIQ was detected in the reaction, confirming that the formyl group of FTHIQ is derived from CO2. The results of control experiments indicated that the reaction was really proceeded in a cascade way. Besides, it was also confirmed that CO2 and H2 are the true C1 and hydrogen sources for the reaction.
Scheme 1. Control experiments of hydrogenation of IQ and N-formylation of THIQ. Reaction conditions are the same as those of in , entry 3.
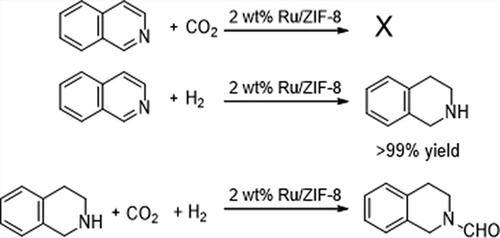
We studied the titled reaction by using diverse common C1 sources including CH3OH, (HCHO)n, and HCOOH [Citation38], and the result are given in . No product was detected using CH3OH, showing that it is not the intermediate for the reaction. Worth noting that unlike the reported homogeneous Co(ClO4)2·6H2O/PP3/K3PO4 catalyst [Citation39], the present catalyst was not acid-resistant, thus HCOOH was probably not the intermediate. When (HCHO)n and CO were assessed, only 21% and 26% yields of FTHIQ were obtained. Taking consideration of that CO was not detected, the HCHO was much easily reduced to CH3OH under the Ru-based catalyst, and the obtained low yields, we speculated that the intermediates were not HCHO or CO.
Figure 8. Effects of diverse C1 source on the catalytic performances (a), the reusability for the catalyst (b), and the hot filtration experiments (c), respectively. Reaction conditions are similar to those of , entry 3.
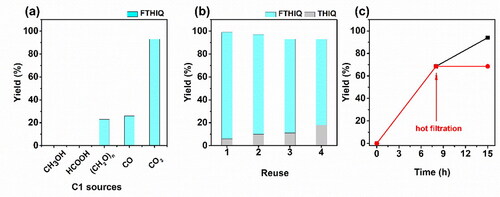
CO2 could be efficiently converted to formyl group, confirming that CO2 shows different catalytic processes compared with the other tested C1 sources. In other words, CO2 could directly participate in the N-formylation of isoquinoline, which was similar to the previous report [Citation27]. Typically, the Ru/ZIF-8 catalyst was initially reduced to Ruδ+/ZIF-8 and Ru0/ZIF-8, which contribute to N-formylation of THIQ and hydrogentaion of IQ, respectively. Then the Ru0/ZIF-8 catalyst could achieve the hydrogenation of IQ to THIQ, which could be reacted with CO2 over the Ruδ+/ZIF-8 catalyst to FTHIQ. During the catalytic processes, CO2 and THIQ were adsorbed on the catalyst surface and coupled and hydrogenated to formyl group over the catalyst.
The reusability of the Ru/ZIF-8 catalyst was examined, with the results given in . The catalyst could be reused at least for four times without obvious loss in activity. The Ru leaching amounts in each run were less than 1% of the feeding amount, which was tested by ICP-OES. The results indicated that the present catalyst has good stability and reusability in the titled reaction. Additionally, we observed the Ru/ZIF-8 catalyst showed an increasing yield of THIQ and a declining yield of FTHIQ. It is probably because the Ru0 species were formed during the reaction, which was confirmed by the TEM tests. The Ru0 species were less active for the N-formylation reaction but beneficial for the hydrogenation reaction [Citation40].
The hot filtration experiments showed the yield of FTHIQ did not increase after removing the catalyst, showed the catalyst proceeded via a true heterogeneous way.
Using the established Ru/ZIF-8 catalyst, we studied the universality for the present strategy, and the results are given in . The oxidized isoquinoline could be efficiently converted into FTHIQ with a 93% yield (, entry 1). The substituted isoquinoline derivatives such as 5-methoxyisoquinoline, 6-methylisoquinoline, and 5-chloroisoquinoline could be converted into corresponding products with high or acceptable yields higher than 70% (, entries 2-4). 3-Methylisoquinoline, however, could only offer 32% yield to the target product for the steric hindrance from the methyl group adjacent N atom (, entry 5). Interestingly, the catalyst could achieve the N-formylation of quinoline with 71% yield, which is much difficult than that of IQ for its lower basicity (, entry 6). No target product was detected using pyrrole as the substrate (, entry 7). The 2-phenylacetonitrile could not yield the N-formylated product, but generate N-methyl-2-phenylethanamine with a 65% yield (, entry 8). The nitrobenzene could not be reacted under the given conditions, either. The results indicated that the present catalytic system can catalyze the tested isoquinoline derivatives, but cannot achieve in pyrrole, 2-phenylacetonitrile, and nitrobenzene.
Table 2. The scope of the substrate in N-formylation using CO2 with H2 over the Ru/ZIF-8 catalyst.a
4. Conclusions
In the present work, we established a supported Ru catalyst, i.e. the Ru/ZIF-8 catalyst in N-formylation of isoquinoline and its derivatives with CO2 and H2. The catalyst showed excellent performances both in hydrogenation of isoquinoline and N-formylation of 1,2,3,4-tetrahydroisoquinoline, and thus it could achieve the one-pot N-formylation of isoquinoline. The developed Ru/ZIF-8 could catalyze the N-formylation of isoquinoline derivatives, and possessed good stability and reusability in the reaction. The present work not only provides an efficient catalyst for hydrogenation of highly unsaturated isoquinoline derivatives, but also affords a straightforward way to use CO2 resource and synthesize the important N-formyl-1,2,3,4-tetrahydroisoquinoline derivatives.
Acknowledgments
The authors gratefully acknowledge the financial supports from National Natural Science Foundation of China (21978160, 22078182, 21803028, and 21908139), the Key Industrial Innovation Project of Shaanxi Provincial Science and Technology Department (2019ZDLGY06-04), and the Key Project of Education Department of Shaanxi Province (21JY005).
Disclosure statement
No potential conflict of interest was reported by the authors.
Data availability statement
All data generated or analysed during this study are included in this submitted article. The raw data shall be made available upon request to the corresponding author.
References
- He M, Sun Y, Han B. Green carbon science: Scientific basis for integrating carbon resource processing, utilization, and recycling. Angew Chem Int Ed. 2013;52:9620–9633.
- Gao W, Liang S, Wang R, et al. Industrial carbon dioxide capture and utilization: state of the art and future challenges. Chem Soc Rev. 2020;49(23):8584–8686.
- Grim RG, Huang Z, Guarnieri MT, et al. Transforming the carbon economy: challenges and opportunities in the convergence of low-cost electricity and reductive CO2 utilization. Energy Environ Sci. 2020;13(2):472–494.
- Zhang Y, Li Y-M, Tan Q, et al. Facile synthesis of two-dimensional copper terephthalate for efficient electrocatalytic CO2 reduction to ethylene. J Exp Nanosci. 2021;16:247–255.
- Yuan Q, Li Y-Y, Yu P-P, et al. Reaction mechanism on Ni-C2-NS single-atom catalysis for the efficient CO2 reduction reaction. J Exp Nanosci. 2021; 16:256–265.
- Eoghan PD, Enrico A, Laurie C, et al. Polyethyleneimine functionalised nanocarbons for the efficient adsorption of carbon dioxide with a low temperature of regeneration. J Exp Nanosci. 2015;10:746–768.
- Chu SL, Yan X-P, Choi C, et al. Stabilization of Cu+ by tuning CuO-CeO2 interface for selective electrochemical CO2 reduction to ethylene. Green Chem. 2020;22(19):6540–6546.
- Hudson R, Graaf RD, Rodin MS, et al. CO2 reduction driven by a pH gradient. Proc Natl Acad Sci U S A. 2020;117(37):22873–22879.
- Liu Y, Fan X, Nayak A, et al. Steering CO2 electroreduction toward ethanol production by a surface-bound Ru polypyridyl carbene catalyst on N-doped porous carbon. Proc Natl Acad Sci USA. 2019;116(52):26353–26358.
- Jiang Y, Choi C, Hong S, et al. Single sites for CO2 reduction. Cell Rep Phy Sci. 2021;2(3):100356.
- Zhang M, Choi C, Huo R, et al. Reduced graphene oxides with engineered defects enable efficient electrochemical reduction of dinitrogen to ammonia in wide pH range. Nano Energ. 2020;68:104323.
- He Z, Cui M, Qian Q, et al. Synthesis of liquid fuel via direct hydrogenation of CO2. Proc Natl Acad Sci U S A. 2019;116(26):12654–12659.
- Seavill PW, Wilden JD. The preparation and applications of amides using electrosynthesis. Green Chem. 2020;22(22):7737–7759.
- Xu D, Zhao H, Dong Z, et al. Catalytically active Co-Nx species stabilized on nitrogen-doped porous carbon for efficient hydrogenation and dehydrogenation of N-heteroarenes. ChemCatChem. 2020;12(17):4406–4415.10.
- Wu Z, Zhai Y, Zhao W, et al. An efficient way for the N-formylation of amines by inorganic-ligand supported iron catalyst. Green Chem. 2020;22(3):737–741.
- Kaziev GZ, Oreshkin AV, Holguin Quinones S, et al. Synthesis and characterization of amminecopper hexamolybdometalates. Russ J Inorg Chem. 2006;51(11):1710–1715.
- Ke Z, Zhang Y, Cui X, et al. Supported nano-gold-catalyzed N-formylation of amines with paraformaldehyde in water under ambient conditions. Green Chem. 2016;18(3):808–816.
- Wang G, Jiang M, Ji G, et al. Bifunctional heterogeneous Ru/POP catalyst embedded with alkali for the N-formylation of amine and CO2. ACS Sustainable Chem Eng. 2020;8(14):5576–5583.
- Zhang L, Han Z, Zhao X, et al. Highly efficient ruthenium‐catalyzed N‐formylation of amines with H2 and CO2. Angew Chem Int Ed Engl. 2015;54(21):6186–6189.
- Dai X, Wang B, Wang A, et al. Amine formylation with CO2 and H2 catalyzed by heterogeneous Pd/PAL catalyst. Chin J Catal. 2019;40:1141–1146.
- Daw P, Chakraborty S, Leitus G, et al. Selective N-formylation of amines with H2 and CO2 catalyzed by cobalt pincer complexes. ACS Catal. 2017;7(4):2500–2504.
- Jayarathne U, Hazari N, Bernskoetter WH. Selective iron-catalyzed N-formylation of amines using dihydrogen and carbon dioxide. ACS Catal. 2018;8(2):1338–1345.
- Cui X, Zhang Y, Deng Y, et al. Amine formylation via carbon dioxide recycling catalyzed by a simple and efficient heterogeneous palladium catalyst. Chem Commun (Camb). 2014;50(2):189–191.
- Wang Y, Chen B, Liu S, et al. Methanol promoted palladium-catalyzed amine formylation with CO2 and H2 by the formation of HCOOCH3. ChemCatChem. 2018;10(22):5124–5127.
- Luo X, Zhang H, Ke Z, et al. N-doped carbon supported Pd catalysts for N-formylation of amines with CO2/H2. Sci China Chem. 2018;61(6):725–731.
- Ju P, Chen J, Chen A, et al. N-formylation of amines with CO2 and H2 using Pd–Au bimetallic catalysts supported on polyaniline-functionalized carbon nanotubes. ACS Sustainable Chem Eng. 2017;5(3):2516–2528.
- He Z, Liu H, Qian Q, et al. Heterogeneous cobalt-catalyzed direct N-formylation of isoquinolines with CO2 and H2. ChemCatChem. 2017;9(11):1947–1952.
- Zhang Y, Dai X, Wang H, et al. Catalytic synthesis of formamides with carbon dioxide and amines. Acta Phys Chim Sin. 2018;34(8):845–857.
- Hosseini-Sarvari M, Sharghi H. ZnO as a new catalyst for N-formylation of amines under solvent-free conditions. J Org Chem. 2006;71(17):6652–6654.
- Han Y, Wu Z, Wei Z, et al. N-formylation of amines using methanol as a potential formyl carrier by a reusable chromium catalyst. Commun Chem. 2019; 2:15.
- Bobbink FD, Das S, Dyson PJ. N-formylation and N-methylation of amines using metal-free N-heterocyclic carbene catalysts and CO2 as carbon source. Nat Protoc. 2017;12:417–428.
- Zhang Y, Wang J, Zhu H, et al. N-formylation of amines with CO2 and H2 by using NHC-Iridium coordination assemblies as solid molecular catalysts. Chem Asian J. 2018;13:3018–3021.
- He Z, Liu H, Qian Q, et al. N-methylation of quinolines with CO2 and H2 catalyzed by Ru-triphos complexes. Sci China Chem. 2017;60(7):927–933.
- Zhu M, Venna SR, Jasinski JB, et al. Room-temperature synthesis of ZIF-8: the coexistence of ZnO nanoneedles. Chem Mater. 2011;23(16):3590–3592.
- Tanaka S, Fujita K, Miyake Y, et al. Adsorption and diffusion phenomena in crystal size engineered ZIF-8 MOF. J Phys Chem C. 2015;119(51):28430–28439.
- Schejn A, Aboulaich A, Balan L, et al. Cu2+-doped zeolitic imidazolate frameworks (ZIF-8): efficient and stable catalysts for cycloadditions and condensation reactions. Catal Sci Technol. 2015;5(3):1829–1839.
- Liu Y, Zhang J, Tan X. High performance of PIM-1/ZIF-8 composite membranes for O2/N2 separation. ACS Omega. 2019;4:16572–16577.
- Li Y, Sorribes I, Yan T, et al. Selective methylation of amines with carbon dioxide and H2. Angew Chem Int Ed. 2013;52(46):12156–12160.
- Liu Z-H, Yang Z-Z, Ke Z-G, et al. Ethanol-mediated N-formylation of amines with CO2/H2 over cobalt catalysts. New J Chem. 2018;42(16):13933–13937.
- Fang J, Wang Z-Q, Wei X, et al. N-Formylation of amines with carbon dioxide and hydrogen catalyzed by ionic Liquid-Assisted Ru complexes. ACS Sustainable Chem Eng. 2021;9(39):13256–13267.