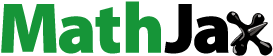
Abstract
For non-small cell lung cancer (NSCLC) treatment, a BCS class II drug, Gefitinib, was widely used. Due to poor bioavailability, uncontrollable drug release, Gefitinib witnessed side effects. To circumvent such associated problems, optimized Gefitinib encapsulated polycaprolactone (PCL) nanoparticles with three different molecular weights of PCL (average Mn∼10,000, Mn∼45,000 & Mn∼80,000) were developed using Box–Behnken design while understanding the influence of critical process parameters of the nanoparticles. For morphological characterizations, SEM, TEM, AFM were used. Hemocompatibility, platelet aggregation, and erythrocyte membrane integrity tests were used to test nanoparticles for biocompatibility; excellent biocompatibility was reported during these tests. The in-vitro drug release studies confirmed that Gefitinib-PCL10,000NPs, Gefitinib-PCL45,000NPs, and Gefitinib-PCL80,000 NPs, show significant initial burst effects, and later nanoparticles possessed zero-order kinetics. The genotoxicity of PCL nanoparticles was assessed by cytokinesis-block micronucleus (CBMN) assay, indicating DNA damage in NCI-H460 cell and micronuclei and nuclear buds’ formation. Further, reactive oxygen species studies, MTT cytotoxicity assays at 24 & 48 h, stability, in-vitro cellular uptake of optimized fluorescent Gefitinib PCL80,000NPs, and apoptosis studies were also carried out. As a result, investigating stable Gefitinib-loaded poly-caprolactone (PCL) nanoparticles could open up new research avenues, potentially lowering side effects and improving Gefitinib's profile in the treatment of NSCLC.
1. Introduction
Gefitinib, a BCS class II drug used to treat non-small cell lung cancer (NSCLC) [Citation1–5]. It works as both an EGFR inhibitor and a tyrosine kinase inhibitor (TKIs) [Citation6]. According to new research, Gefitinib has a lower solubility and a slower onset of action in the gastric environment at pH 4–6. Gefitinib was found to have a log-P value of 4.15, indicating that it has a higher hydrophobic property [Citation7]. Furthermore, hepatic elevations of alanine aminotransferase, anorexia, stomatitis, vomiting, diarrhea, and nausea were reported to occur with the 250 mg marketed Gefitinib tablets, which had only 44% bioavailability [Citation8]. Moreover, despite their lack of understanding of new polymers, the effects of critical attributes and critical process parameters, and the basic criteria for polymeric nanoformulations such as smaller particle sizes, entrapment efficacy percentage, and polydispersity index, modern scientists are turning to polymeric nanotechnological approaches to tackle these issues [Citation9, Citation10]. As a result, one of the goals of this study is to create a Gefitinib control release formulation that uses a quality by design approach, which could have rapid onset of action, increased drug solubility profile, and better drug entrapment efficacy [Citation11].
According to Navya Sree Kola Srinivas [Citation12], Gefitinib, a tyrosine kinase receptor, can be encapsulated using Eudragit® RL100; Gefitinib polymeric nanoparticles can be made using homogenization ultrasonication techniques. Gefitinib polymeric nanoparticles were found to be nontoxic in cytotoxicity tests on Vero cell lines [Citation13]. Gefitinib's relative bioavailability increased up to 1.812-fold when encapsulated with Eudragit® RL100, according to bioavailability studies [Citation12]. The combination of Gefitinib and GSK461364A, according to Praveena Velpurisiva et al. (2019), could have synergistic effects and improve bioavailability. Anti-glioblastoma activities were tested against U87-MG using a combination of poly (lactic-co-glycolic) acid and polyethylene glycol (PLGA-PEG) nanoparticles. The results of this experiment suggest that encapsulating GSK461364A and higher concentrations of Gefitinib in nanoparticles results in synergistic glioma cell killing. One thing is clear from these two studies: Gefitinib polymeric nanoparticles have superior anticancer activity [Citation14, Citation15]. Controlled polymeric nanoparticles of Gefitinib may have a greater concentration of drug at the site of action than traditional delivery [Citation16]. Furthermore, free Gefitinib is hindered by efflux mechanisms and multidrug resistance, compared to the controlled polymeric nano-drug delivery system, resulting in a poor binding site for Gefitinib in cancer cells [Citation14, Citation17, Citation18]. It is feasible to improve permeability and drug retention in tumour tissues using Gefitinib polymeric nanoparticles with regulated polymeric nanoparticles. Researchers are constantly working on chitosan [Citation19–25], poly(lactic-co-glycolic acid) (PLGA) [Citation26, Citation27], and polysarcosine (pSar) [Citation28] to improve control release characteristics of polymeric nanoparticles. Polycaprolactone (PCL) [Citation29, Citation30], a semi-crystalline biodegradable and biocompatible polyester was the subject of very little research in recent times. The PCL has the unique ability to prolong mean residence time, which aids tumor targeting [Citation31]. PCL degrades more slowly than other biodegradable polyesters; this property can be used to deliver drugs to target tissues in a controlled manner over time [Citation32, Citation33]. As per Qianqian [Citation34], co-delivery of Paclitaxel and IR780 for drug-resistant ovarian cancer using poly-ε-caprolactone nanoparticles (PCLNPS) has promising new strategies for cancer therapy [Citation34, Citation35]. To make PCLNPs more target-specific, luteinizing hormone-releasing hormone receptors (LHRH) were grafted covalently onto the surface of PCLNPs, which enhances the cellular internalization and selective targeting in ovarian tumor cells. In another research, Oxaliplatin-loaded PCL polymeric nanoparticles, according to Ozgur Esim et al. (2020), could be a potential treatment for non-small cell lung cancer (NSCLC) [Citation36]. However, the optimized batch could only provide 1.45 ± 0.13% drug loading and 29.17 ± 0.74% drug entrapment efficacy with moderate cellular uptake and cytotoxicity, according to their findings. As a result, there are significant opportunities to improve PCL's drug loading capacity. One of the main goals of this study is to improve the Gefitinib delivery profile through PCL, which has excellent anticancer properties and cellular internalization [Citation37]. PCL drug delivery is well-known for delivering drugs to specific organs via injection or ingestion. When PCL encapsulated Gefitinib delivery is proposed, it is critical to consider the established relationship between critical process parameters such as homogenization speed (rpm), PVA concentration (%), and PCL concentration (%) and critical quality attributes such as particle size (nm), PDI, zeta potential (mV), and entrapment efficacy (%). To quantify the relationship between the dependent and independent variables in this experiment, the Box-Behnken design model established [Citation38]. The inclosing an experimental design aids in the identification of the combined effects of independent variables on the various characteristic profiles of PCL-loaded Gefitinib nanoparticles [Citation39]. The semicrystalline aliphatic polyester called polycaprolactone (PCL) was the best choice for controlled delivery of Gefitinib because of its superior biocompatibility, slow degradation rates, very low glass transition temperature, and biodegradable properties [Citation40].In this experiment, measuring particle size and zeta potential was crucial; the smaller the particle size, the lower the reticuloendothelial opsonization [Citation41], and the better the drug's plasma level stability. Higher anionic zeta potential required for greater formulation stability, as it can improve drug encapsulation efficiency and maximise drug content in the target tissues [Citation42]. Apart from particle size, zeta potential, drug loading, percentage drug entrapment measurement, polymeric nanoparticles were subjected to characterizations using a scanning electron microscope (SEM), transmission electron microscopy (TEM), atomic force microscopy (AFM). Further, hemocompatibility, platelet aggregation, and erythrocyte membrane integrity tests were performed to ensure that the prepared formulations were biocompatible.
In this experiment, prepared Gefitinib PCL10,000NPs, Gefitinib PCL45,000NPs, and Gefitinib PCL80,000NPs were evaluated for their efficacy in non-small lung cancer using the NCI-H460 cell lines. Furthermore, MTT cytotoxicity assay at 24th and 48th hour, cellular uptake, cytokinesis-block micronucleus (CBMN) assay, reactive oxygen species studies, in-vitro cellular uptake of optimized fluorescent Gefitinib PCL80,000NPs, the process of programmed cell death analysis or apoptosis studies were also performed. As a result, studying Gefitinib-loaded poly-ε-caprolactone (PCL) nanoparticles could open up new avenues of research, potentially reducing side effects and improving Gefitinib's pharmacokinetic profile in the treatment of non-small cell lung cancer (NSCLC) [Citation43, Citation44]. However, to fully grasp this research at the molecular level, in vivo imaging and xenograft in an animal model are required.
2. Materials and methods
2.1. Materials
Gefitinib was a gift sample from Neon Laboratories Limited, Mumbai, India. Polycaprolactone (average Mn∼10,000, Mn∼45,000 & Mn∼80,000) was purchased from Sigma-Aldrich, Bengaluru, India. The methanol, dichloromethane, polyvinyl alcohol was purchased from Sisco Research Laboratories Pvt. Ltd. Navi Mumbai, India. Nonoxynol-9(Polyoxyethylene glycol alkylphenol ether), LDH assay kit/lactate dehydrogenase assay kit (Colorimetric) (ab102526), Annexin V-FITC apoptosis staining/detection Kit (ab14085) was a gift sample from Abcam, USA. Triton X-100 was purchased from Merck Specialities Pvt. Ltd, Mumbai, India. Lishman’s stain solution was purchased from Avntor Performance Materials India Ltd., Thane, India. NCI-H460 cells were procured from Radiant Research Services Pvt Ltd., Bengaluru, India. MTT (3-(4,5-Dimethylthiazol-2-yl)-2,5-Diphenyltetrazolium Bromide) reagent was purchased from Thermo Fisher Scientific, Mumbai, India. All other chemicals were of analytical grade.
2.2. Preparation of Gefitinib loaded nanoparticles
Gefitinib-loaded nanoparticles were prepared with minimal alterations employing the emulsion solvent evaporation technique [Citation45]. In the solvent evaporation technique, the drug is dissolved, disseminated, or emulsified in an organic polymer solution before emulsifying into an external aqueous or oil phase. This method is the most reliable and widely used for making lab-scale polymeric nanoparticles. Coumarin-6 encapsulated poly-ε-caprolactone (PCL), poly(lactic acid) (PLA), and poly(lactide-co-glycolide) (PLGA) nanoparticles were prepared for potential biomedical applications using the spontaneous emulsification solvent evaporation (SESE) method, according to Marta Szczch et al. (2020) [Citation46]. The findings of this study show that the SESE technique can improve drug stability and integrity within polymeric nanoparticles.
In the current study, three different molecular weights of polycaprolactone (PCL) were used in this experiment (PCL-average Mn∼10,000, PCL average Mn∼45,000, PCL ∼ average Mn∼80,000). Initially, 20 mg of PCL were dissolved in 10 mL acetone-free methanol and stirred for 30 min. To create a pale white colour mixture, 5 mg of Gefitinib (Average Mn446.9 Da, BCS-II; Log P: 4, highly lipophilic) was progressively added into this polymeric solution while stirring. The aqueous phase was made by adding 2–3 drops of Nonoxynol-9(Polyoxyethylene glycol alkylphenol ether) to 10 mL double distilled water. The Gefitinib PCL polymeric solution was gradually added to the water phase containing Nonoxynol-9. The solution mixture was stirred for 15 min at various homogenizing speeds using a T 25 digital ULTRA-TURRAX® homogenizer of Germany. The foamed and milky initial emulsion was then homogenized for 15 min in 15 mL of PVA solution. The methanol in the resulting mixture was allowed to evaporate at room temperature before being purified three times using an Eppendorf Vacufuge plus at 12,500 RPM for 2 h at 4 °C until pale white particles were produced in the micropipette bottom. Before lyophilization, the particles were treated with a 2.5% trehalose (cryoprotectants) solution using Millrock Technology Inc's REVO lyophilizer to maintain and enhance the stability of polymeric nanoparticles. Trehalose (cryoprotectants) solution was utilized to conduct appropriate lyophilization [Citation47]. The polymeric cryoprotectant solution was stirred at 750 rpm for 15 min using a Bio-Gene Magnetic Stirrer before being kept for 48 h in a 4-type T thermocouple product sensor. The Lyophilization technique began after 48 h. 230 V/1 ph/60 Hz/30A was utilized for lyophilization, and the temperature was controlled between −53 °C and 85 °C for 24 h with a vacuum pressure of around 100 mTorr [Citation48]. Free-flowing nanoparticles were succeeded.
2.3. Experimental design
Critical process parameters (homogenization speed(A)) and critical material attributes (PVA Concentration(B), PCL Concentration(C)) were studied using an experimental methodology to better understand the impacts of independent variables on critical quality characteristics (Particle size, PDI, Zeta Potential, Entrapment efficacy). The whole design was constrained for 17 runs using Design Expert (Stat-Ease; version 11.0) software and the Box-Behnken design of response surface methodology (RSM) model. shows three levels of independent variables (1, 0, and + 1) and critical process parameters, and critical material attributes that impact nanoparticle outcome. Independent factors impacting essential quality characteristics were evaluated using polynomial equations and 3-dimensional surface plots.
Table 1. Critical process parameters which influence various critical quality attributes.
According to Pradipta [Citation49], the initial Plackett-Burman design was used to develop telmisartan-loaded polymer (PLGA) nanoparticles, and subsequent formulations were designed using the Box-Behnken design (BBD) [Citation49]. Critical quality attributes included particle size, encapsulation efficiency, and zeta potential. The successful execution of the quality by design approach could correlate the significant parameters that influence the formulations both directly and indirectly.
The research findings suggest that the inception of the Box-Behnken design of response surface methodology (RSM) model in the current study would be a key component in developing Gefitinib loaded PCL nanoparticles.
2.4. Characterization of PLC-polymeric nanoparticles
2.4.1. Particle size, PDI, zeta potential & morphology
Mean particle size and size distribution were measured by Delsa Nano C photon correlation spectroscopy (PCS) (Beckman Coulter, USA) [Citation50]. At 25 °C and 7421 cps light intensity, the particle size was estimated while keeping samples in a polystyrene cuvette. From the z-average diameter, polydispersity index (PDI) was measured. DelsaNano C zeta potential analyser (Beckman Coulter, USA) estimated zeta potential in the presence of dual laser light. During zeta potential measurement, 0.9 mL of 10-time diluted polymeric nanoparticles solutions were kept in the sample holder while maintaining 160° scattering angle. The velocity of the particles was converted into zeta potential using the Helmholtz-Smoluchowski equation. For morphological understanding, the scanning electron microscope (SEM) (Zeiss, Jena, Germany) was first performed. While performing SEM, 100 µL of polymeric solutions were diluted with 2 mL of distilled water, and minute polymeric solutions were placed into a cover slip and dried at room temperature. Using sputter coater, the dried film was coated with gold in higher vacuum conditions, and the coated film was scanned under SEM maintaining a resolution of 0.4 nm @ 30 kV, 1.2 nm @ 1 kV. To understand better the magnifications and internal morphology of the prepared polymeric nanoparticles Transmission Electron Microscopy (TEM) was performed (Hitachi 7500, Japan). Prior to performing TEM studies, nanoparticles were carbon-coated and placed in a copper grid utilizing 1% Phosphotungstic acid as the native stain. Anatomic force microscope (AFM) (AFM5300E, Hitachi, Japan) was used under semi-contact mode and high-vacuum conditions to understand the mechanical properties and the external morphology of polymeric nanoparticles. In semi conduct mode, the AFM prob could move in two directions. One drop of nanoparticles suspensions was placed in the microscopic slide and was kept in room temperature until a film was formed. The 3D images were developed, and subsequently, average roughness average height was calculated.
Atractylodin-loaded PLGA nanoparticles were developed by Nada [Citation51] for cholangiocarcinoma (bile duct cancer). Particle diameter, PDI, and zeta potential, as well as transmission electron microscopy, are being used to characterize the nanoparticles. After 24 h of exposure, Atractylodin-loaded PLGA nanoparticles were tested for cytotoxicity against the CCA, CL-6 cell line [Citation51].
According to the above-mentioned research protocol, particle size, PDI, zeta potential, and morphological analysis are all key components of any polymeric nanoparticle analysis. As a result, current research on Gefitinib-loaded PCL nanoparticles necessitates a thorough characterization profile before this product can be developed.
2.4.2. Determination of encapsulation efficiency
The encapsulation efficiency of Gefitinib in polycaprolactone (PCL) encapsulated nanoparticles was determined by the reverse-phase HPLC method. In this operation, Agilent 1120 Compact LC HPLC system with gradient pump (4 MPa or 40barr) was utilized. 10 mg of lyophilized Gefitinib encapsulated PLC nanoparticles were dissolved into 10 mL of mobile phase (0.1% Trifluoroacetic acid: Methanol at 35:65 ratio) and filtered using 0.45 µm nylon filter paper (PALL) [Citation52]. 10 µL filtrate of nanoparticles solutions were injected into HPLC, maintaining C18 5 µm 250 × 4.6 mm (Agilent) as stationary phase. The amount of Gefitinib was detected using 1260 Infinity II variable wavelength detector at 246 nm. The HPLC method for Gefitinib was validated, and the linearity range of different standard concentrations was determined. The concentration range was maintained at 5–30 µg/mL, having a determination coefficient of R2 = 0.9988. The detection, quantification limit, theoretical plates, and trailing factor were 1.473 µg/mL, 4.412 µg/mL, 3776, and 1.21881, respectively. The Gefitinib encapsulation efficiency and the drug loading percentage was calculated using the following formula:
The results were replicated thrice, and their mean values ± SD were reported.
2.4.3. In vitro Gefitinib release
In-vitro drug release studies were performed in the presence of pH 7.4 and pH 5.4 phosphate buffer solution. 5 mg of lyophilized Gefitinib loaded Polycaprolactone (PLC) nanoparticles poured in pH 7.4 and pH 5.4 phosphate buffer solution (20 mL each) and further ultra-sonicated in centrifugation tubes. From the resultant suspension, 2 mL was withdrawn and wrapped in a 12–14 kDa dialysis tubing cellulose membrane (Millipore Sigma, Bangalore, India). The filled dialysis tube was tied in two side and hatched in 30 mL pH 7.4, and 30 mL pH 5.4 phosphate buffer solution and stirred continuously at 100 rpm at 37 °C. At specific time intervals from the released media, 1 mL of samples were collected and replaced with fresh buffer solution. Using RP-HPLC, the released Gefitinib was analyzed at 246 nm. The In vitro release of free 5 mg Gefitinib in the same conditions was included as a control. The release kinetics were assessed after drug release data were fitted into Higuchi, first order and zero order kinetics. The best kinetic model was selected by comparing with obtained correlation coefficients.
According to Souvik [Citation53], when Gefitinib (G) and Simvastatin (S) are combined to form an inclusion complex with β-cyclodextrin, they can have excellent in-vitro drug release profiles. The dissolution medium for the in-vitro drug release profile was pH 7.2 phosphate buffer. After 480 min or 8 h, the inclusion complex of Gefitinib (G) and Simvastatin (S) shows an 85.96% drug release. The findings suggest that proper encapsulation of Gefitinib is required to maintain its formulation's release pattern [Citation53].
2.4.4. Stability of nanoparticles
As per ICH Q1A(R2) guideline the stability of nanoparticles was determined by estimating particle size, zeta potential, drug entrapment efficacy and formulation physical appearance for 6 months at 5°C ± 3 °C storage conditions [Citation54].
2.4.5. Haemolysis study
Haemolysis study could support understanding the behavior of Gefitinib; Gefitinib loaded polycaprolactone (PLC) nanoparticles and placebo after ingested into blood capillaries [Citation55]. Therefore, to investigate the quality standard and biocompatibility of the formulation, haemolysis study is required. Blood was obtained from the nearest blood bank to conduct haemolysis test. The blood samples (2.5 mL) were centrifuged for 15 min at 1345xg at room temperature. The graduated centrifuge tubes used in this experiment were previously sterile. Micropipettes were used to scrape the plasma coating during centrifugation. The developed erythrocyte pellets in the bottom of the graduated centrifuge tubes were diluted and suspended further using normal saline solution. At room temperature, the suspended part was centrifuged against 1345xg for 15 min. Centrifugation was used three times to finish the washing operation. The erythrocyte suspension was further diluted with 10 mL saline water, and then 10, 50, and 100 µg/mL of Gefitinib, Gefitinib loaded polycaprolactone (PLC) nanoparticles and placebo combined with 2 mL of erythrocyte suspension in sterile tubes separately. In positive controlled, which fully haemolyzed erythrocytes, was rendered by dissolving 1% triton X-100 in erythrocyte solutions. The negative control test, in which erythrocytes are not haemolyzed, was made by suspending erythrocytes in regular saline solution in previously sterile Eppendorf tubes. The samples were incubated for at least 15 min at 37 °C. Nearly 200 µL samples were removed at specific pre-determined time intervals, i.e. 0.5, 1, 2, 4, 5, 6, 7, 8, 9, and 10 hr, thus centrifuging at 1345xg for 15 min. A 100 µL supernatant was incubated for 45 min at room temperature to achieve adequate hemoglobin to oxyhaemoglobin conversion. At 580 nm, the absorbance was measured spectrophotometrically. The following formula is used to calculate the proportion of haemolysis:
where A sample is the absorbance of the test sample containing the drug loaded nanoparticles. A spontaneous control is the absorbance of erythrocytes that are previously incubated with saline water. On the other hand, A positive control was the absorbance of the supernatant of erythrocytes which was exposed with 1% Triton X-100 solution made up of normal saline. The experiment was performed in triplicate, and the data were expressed in mean ± SD (n = 3).
It is critical to understand the impact of nanoparticles on red blood cells, according to G Barshtein et al. [Citation56]. Polystyrene nanoparticles (PS-NP) were tested for hemolysis in their study. The findings suggest that the presence of protein in the hemolytic medium may affect hemolytic activity [Citation56].
2.4.6. Platelet aggregation studies
In their research, Erica [Citation57] emphasised the importance of the platelet aggregation factor. In vitro platelet aggregation may be induced by polystyrene latex nanoparticles (PLNPs), which may increase cardiovascular risk [Citation57]. The researchers also wanted to know how surface engineering of nanoparticles in the 50–100 nm range [amine-modified (aPLNP) and carboxyl-modified (cPLNP)] affected platelet aggregometry. Their research also found that NPs may increase the risk of platelet-driven events by inducing or enhancing platelet aggregation through mechanisms dictated by their unique nano-size and surface chemistry combination. As a result, according to the current study, determining the in vitro platelet aggregation factor is critical in order to determine the integrity and impact of Gefitinib PCL nanoparticles on blood.
Platelet aggregation experiment was performed using a haematological counter (Biolinx Lab systems Private Limited, India) [Citation58]. Before platelet counting, 2 mL of blood was incubated with different Gefitinib concentrations for 3 h at 37 °C, i.e. 10, 50, and 100 µg/mL. After incubation, the required volume of sample was diluted with standard saline water, and additional samples were examined using a triplicate haematological counter (mean SD; n = 3). It is important to do a detailed study of platelet aggregation, which can be performed with an optical microscope. In this experiment, heparinized blood samples were treated with various samples. After treatment with the samples, peripheral blood smears were mounted on a glass slide and air-dried for 5 min. With the aid of Leishman's dye, the blood smears were pigmented for 10 min. The stain was then washed with purified water and covered with a glass cover. The staining essence of platelets was examined using an optical microscope, and high-resolution images were captured.
2.4.7. Evaluation of erythrocyte membrane integrity
Lactate dehydrogenase (LDH) is an enzyme that is produced by erythrocytes and can be determined photometrically with the LDH assay kit/Lactate Dehydrogenase Assay Kit (Colorimetric) (ab102526) [Citation59]. The erythrocyte suspension was prepared according to the protocol previously discussed on haemolysis. 1 mL of erythrocyte suspension was used to administer the Gefitinib, Gefitinib loaded polycaprolactone (PCL) nanoparticles and placebo. The positive control sample (100% hemolyzed erythrocytes) was made by diluting the erythrocyte suspension with 1% Triton-X-100, while the negative control sample was made by diluting the erythrocyte suspension with normal saline solution. To allow LDH natural preparation, 150/UL Lactate dehydrogenase (LDH) was incubated with erythrocyte suspension at 37 °C. During specific time periods, such as 2 h, 4 h, and 8 h from suspension, 400 µL samples were extracted and independently centrifuged at 1345xg for 20 min. LDH was detected at 500 nm after the supernatant was prepared with a ready-to-use LDH solution. The sum of LDH was calculated using the formula below.
A sample stands for Gefitinib, Gefitinib loaded polycaprolactone (PLC) nanoparticles incubated erythrocytes absorbance, A negative control indicates erythrocytes absorbance of sample, which was pre-treated with normal saline solution. The A standard indicates the absorbance of erythrocytes sustention, which were pre-treated with 150/UL Lactate dehydrogenase (LDH) enzyme. The experiment was performed in triplicate, and the date is expressed as mean ± SD (n = 3)
The survival rate of RBC cells after being injected with nanoparticles depends on the erythrocyte membrane integrity test. Yuanyuan [Citation60] developed erythrocyte membrane-encased poly(d,l-lactide-co-glycolide) (PLGA) nanoplatforms for cancer immunotherapy [Citation61, Citation62]. In vitro cell uptake was said to be improved with these formulations [Citation60].
2.4.8. Cytotoxicity assay
The MTT [(3-(4, 5-dimethylthiazol-2-yl)-2, 5-diphenyl tetrazolium bromide)] assay was used to determine cellular metabolic activity, cytotoxicity, and cell viability. For the first time, Mosmann T et al. discussed the MTT assay, a colorimetric assay system [Citation63].MTT assay helps to identify cell growth inhibitions of NCI-H460 [ATCC HTB-177, Manassas, VA, USA] cells when treated with free Gefitinib and Gefitinib loaded polycaprolactone (PCL) nanoparticles. Basically, MTT is a pale-yellow substrate that is cleaved by living cells to yield a dark blue formazan product [Citation64]. The amount of MTT cleaved is thus directly proportional to the number of viable cells present, which can be determined using colorimetric method. For NCI-H460 cell growth, a continuous flow of 5% CO2 was maintained. The Dulbecco’s Modified Eagle’s Medium (DMEM) had been utilized for cell growth. The NCI-H460 cells were seeded on 96 well plates, and cell viability was determined after incubating NCI-H460 cells with varying concentration of free Gefitinib and Gefitinib loaded polycaprolactone (PCL) nanoparticles for 24th and 48th hours.
2.4.9. Cellular uptake of nanoparticles
The 12-well plates were seeded with NCI-H460 cells at a density of 5 × 104 per well. The cells were attached for 24 hr. The medium in each well plate was replaced with 1 mL of freshly prepared Gefitinib loaded polycaprolactone (PCL) nanoparticles suspension, and plates were further incubated at specific temperature for 1 to 4 hrs. After incubation, cells were washed three times with chilled pH 7.4 phosphate buffer solution to remove un-internalized nanoparticles. Further, cells were dissociated from wells using a proteolytic enzyme called trypsin. Further, 100 µL of NCI-H460 cells lysates were withdrawn and cells were extracted using 1 mL methanol. As per previously described RP-HPLC method, Gefitinib was analysed from the obtained solution [Citation65].
2.4.10. Apoptosis induced by Gefitinib loaded polycaprolactone (PCL) nanoparticles
The Annexin V test was used to determine if Gefitinib-loaded polycaprolactone (PCL) nanoparticles could cause apoptosis or not. NCI-H460 cells were seeded in a flask, and further flasks were incubated with Gefitinib loaded polycaprolactone (PCL) nanoparticles for 24 h at 37 °C. The cell supernatant was washed with pH 7.4 phosphate buffer solution three times. Annexin V-FITC was used to incubate washed cells for another 20 min, maintaining a dark environment at room temperature. Using ZE5 cell flow cytometer analyzer (Bio-Rad, USA), the samples were analyzed [Citation66, Citation67].
2.4.11. Cell–growth curve
A total of 20,000 NCI-H460 cells/cm2 were seeded in 6 Multiwell Plates with a diameter of 35 mm to achieve 90% confluence. Furthermore, cells were counted using an RS PRO USB Digital Microscope (Noida, India) after being diluted in a 1:10 ratio with trypan blue dye. The experiment was carried out for 4, 24, and 48 h at 5, 25, and 75 µg/mL concentrations of individual Gefitinib-PCL10,000 NPs, Gefitinib-PCL45,000 NPs, Gefitinib-PCL80,000 NPs, and measurements were taken at each time interval. Each experiment was repeated three times, with the mean and standard divisions recorded.
2.4.12. Detection of reactive oxygen species (ROS)
The reactive oxygen species (ROS) concentration was determined using the total reactive oxygen species (ROS) Assay Kit 520 nm (Invitrogen TM, Thermo Fisher Scientific, USA). NCI-H460 cells were seeded at a density of 10,000 cells/cm2 in 96 well plates. After the 24th hour, the cells were stained with Thermo Fisher Scientific's ROS stain 1X (catalog number: 88-5930-74) and incubated for 60 min at 37 °C before being resuspended in dimethyl sulfoxide (DMSO) medium for further incubation. After incubation, the resultant medium was removed, and an aliquot concentration of DMEM medium with hydrogen peroxide was added to the control group and the rest parts of the 96 well plates, adhere to cells were treated with Gefitinib-PCL10,000 NPs, Gefitinib-PCL45,000 NPs, Gefitinib-PCL80,000 NPs solution at 5 µg/mL, 25 µg/mL, and 75 µg/mL at 0, 15, 30, 45, 60, and 24 h, plates were read using a BioTek Synergy H1 Hybrid Multi-Mode Reader (Agilent Scientific Instruments, USA). Each experimental condition was replicated three times, and the fluorescence data were measured up to the 24th hour.
2.4.13. In-vitro Cytokinesis-Block micronucleus (CBMN) assay
As per the Guidelines for Multinational Enterprises (OECD), the Cytokinesis-Block Micronucleus (CBMN) assay was performed with minor modifications. The CBMN is one of the most reliable assays for genotoxic carcinogens. It is often observed due to carcinogenic anomalies within the cells, one of the daughter nuclei could not be able to conceive the right chromosome, due to which a small nucleus formed near the parent nucleus, which was eventually called a micronucleus. Such abnormal micronucleus leads to genotoxicity and chromosomal instability. Therefore, the cells could be a potential biomarker of chromosomal damage. The CBMN assay detects clastogen and antigens, which causes DNA damage and so chromosomal deformation. During this experiment, NCI-H460 cells were seeded into the four 6 well plates with 2.5 × 105 cells/well. Cells were treated with 5 µg/mL, 25 µg/mL, and 75 µg/mL solutions of individual Gefitinib-PCL10,000 NPs, Gefitinib-PCL45,000 NPs, Gefitinib-PCL80,000 NPs respectively and incubated with Cytochalasin B for 1.5–2.0 cell cycles. As a positive control sample, Gefitinib was used.
Further, cells were transferred to 15 mm centrifuge tubes for Micronucleus harvest. All the centrifuge tubes transfer to a microcentrifuge and centrifuge for 5 min at 154 RCF. After centrifugation, supernatants were discarded, and cell pellets were obtained. Cells were further washed with a 6.8pH phosphate buffer solution to remove any excess medium and unwanted cells. Cells were treated with 5 mL of 0.56% KCL solution/sample and further centrifuged. Discard the supernatant, add 5 mL of fixative I (Methanol, acetic acid, and NaCl), and incubate for 5 min and again centrifuge. Once again, supernatants were discarded and 5 mL fixative (1 parts of acetic acid and 5 parts of methanol) were added to the cells. The resuspended cells were incubated for 10 min and centrifuged at for 5 min at 154 RCF. The cell suspensions were further incubated at 4 °C for 24 h. For making slides for automated scoring of micronucleus, cells were prepared after adding 25 µL of fixative I into 75 µL of cell suspension and put directly into silds. For nuclear standing for micronuclear analysis, a fluorescent stain called DAPI (4′,6-diamidino-2-phenylindole) was added across the length of pre-dried cell mount slides. The complete staining of DAPI mounted cells was covered with coverslips and a metamorph semi-automated microscopy system; cells were scored using 10 magnifications under the DAPI filter. Cells containing micronuclei (MN) were recorded, and cytokinesis block proliferation index (CBPI) was estimated for each slide The frequency of mononuclear cells was calculated using the following formula.
Moreover, total cells and nuclear buds (NBUDs) were determined to estimate genotoxicity. 1000 binucleated cells are equal to 1000 micronuclei. A total of 1000 binucleated cells are referred to as Nuclear Buds utilized for this experiment. The experiment was conducted three times (n = 3; mean ± SD)
2.5. Statistical analysis
Graphpad Prism version 9.1.2 and Sigma Plot 14.0 was used to analyze in vitro data. Findings of analysis of variance (ANOVA) and student t-test helps to demonstrate statistical differences (p < 0.05). The experiment was performed in triplicate, and the date is expressed as mean ± SD (n = 3).
3. Results and discussion
3.1. Factorial design
During the emulsification solvent evaporation method, stable droplets containing polycaprolactone (PLC) polymeric solution were formed in the inner phase and the aqueous phase on the outer surface. During evaporation, the organic phase was removed from the solvent mixture. The factors responsible for adequate drug loading capacity were minimum particle size, narrow particle size distributions, appropriate zeta potential. As per Shah Pranav et al. (2021) research, 32 factorial design was used to prepare PLGA based Clonazepam nanoparticles [Citation68]. The amount of PLGA and Poloxamer 188 concentration was considered as two independent factors, and entrapment efficacy (%), particle size (nm), and cumulative drug release (%) at 24th hour were considered as dependent factors. In this investigation, homogenization speed (rpm), PVA concentration (%), PCL concentration (%) was considered as independent variables which influence various critical quality attributes like particle size (nm), PDI, zeta potential (mV), entrapment efficacy (%). The quadratic models were calculated for independent variables. The variables having p-value >0.05 within the model were eliminated and significant values were identified.
3.2. Effect of independent variables on Gefitinib loaded polycaprolactone (PCL) nanoparticles
3.2.1. Particle size
The average particle size of all 17 batches of Gefitinib loaded polycaprolactone (PCL) nanoparticles were shown in . Based on the alteration of independent variables like, homogenization speed (rpm), PVA concentration (%), PCL concentration (%), the mean particle size varies from 190.76 ± 15.38 nm (F17) to 256.21 ± 15.67 nm (F1). The responses of particle size in multiple regression quadratic model in the presence of two independent variables generate quadratic polynomial equation. indicates the impacts of each factor and their interactions on the size of the nanoparticles; those formulations that had p < 0.05 were statistically significant. indicates that changes in process variables had a more significant impact on particle size. As per Ozgur Esim et al. (2020) research findings [Citation69], in the presence of 0.3% PVA, >1000 nm oxaliplatin loaded nanoparticles were developed. However, in this research, in the presence of 0.5 to 1.5% PVA and 10,000 to 15,000 rpm homogenization speed, >300 nm Gefitinib loaded polycaprolactone (PLC) nanoparticles was developed; indicating the evolution of best process optimization. Higher PVA concentration with higher homogenization speed decreases the particle size. The presence of PVA in nanoparticles solutions plays a pivotal role in the emulsification process and formulation stabilization during the solvent evaporation method. According to Antje [Citation70], a 0.25% w/v PVA concentration in Polycaprolactone nanoparticles increased the nanoparticles' stability, allowing the formation of 150 to 300 nm particles with PDI <0.3. The absence of PVA, on the other hand, destabilizes the particles [Citation70].
Table 2. Effect of critical process parameters on critical quality attributes of F1–F17 batches of polymeric nanoparticles.
Table 3. Parameters of the response’s surfaces obtained from Box–Behnken design.
In the current research, lower concentration of PVA and homogenization speed results in nanoparticles aggregation, larger particle size and instability. However, increased concentration of PVA (1.5%) ensure higher viscosity on the outer phase of the emulsion resulting in higher particle size. As a result, increasing PVA concentrations from 1% to 1.5% has less effect on particle size, while increasing PVA concentrations from 0.5% to 1% had significantly decreased the particle size. The PCL concentration (1% to 3%) had significant effects (p > 0.05) on particle size. Increased concentration of PCL could increase the particle size of the nanoparticles. Due to the presence of higher polymer concentration in dispersion phase, poor dispersibility can be observed into aqueous solution. Adejumoke Lara [Citation71] research findings are almost cognitive with current research. Their study showed that increasing the concentration of Polycaprolactone (PCL) within nanoparticles (0.6–10% w/w in acetone) increased particle size from 190 nm to 350 nm [Citation71].
The higher viscosity results in resistance to shear forces, which ultimately generates higher droplet size of the particles. Higher homogenization speed results in fast dispersion of the nanoparticles with advanced energy coefficient. This phenomenon decreases particle size. From it can be understood that higher concentration of PCL and PVA with higher homogenization speed could decrease the particle size. The data retrieved from the experiments were subjected to multiple regression analysis and fitted into polynomial quadratic equation No. 1:
(1)
(1)
Figure 1. Response surface plots for Gefitinib loaded PCL NPs, homogenization speed, PVA concentration, and PCL concentrations on (a) particle size, (b) PDI, (c) zeta potential and (d) encapsulation efficiency (%) (e) overlay plot of design space and optimum batch (f) process optimisation by desirability approach.
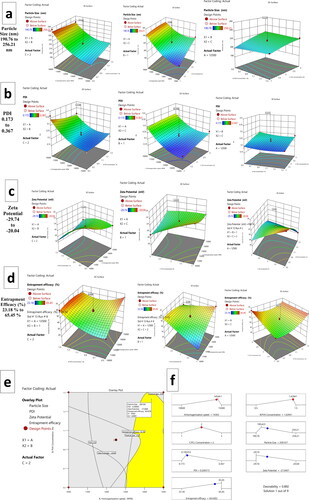
indicates model co-efficient for particle size. The term A, C & A2 was found to be significant (p < 0.05)
3.2.2. Polydispersity index
To know about the physical stability of the nanoparticles, polydispersity index (PDI) is an essential parameter.
Pentamidine-loaded nanoparticles and phosphatidylcholine liposomes were compared by Geofrey [Citation72]. The goal of this study was to see if these two formulations could cross the blood-brain barrier in vitro. When the PDI of these two formulations was measured, it was discovered that freeze-dried pentamidine loaded liposomes had a PDI greater than 0.6. Non-lyophilized pentamidine loaded PCL NPs, on the other hand, showed particle size uniformity with a low PDI (0.25 ± 0.15) and lower aggregation [Citation72].
It is advisable to have PDI <0.5 for narrow mean particular distribution of the nanoparticles. However, for homogeneous particles distribution PDI < 0.2 is most preferable. In this study all the 17 formulations have PDI ranged from 0.173 ± 0.016 (F17) to 0.367 ± 0.002 (F1) (). Almost all the formulations have had PDI in acceptable range. From it was understood that in the presence of increased homogenization speed lower PDI was obtained. A rise in PVA concentration could reduce PDI. The effect of variables on Polydispersity index was mentioned in , where it was understood that Homogenization (A) speed had significant effects on PDI (p < 0.05). The data retrieved from the PDI experiment were subjected to multiple regression analysis and fitted into polynomial quadratic equation No. 2(2)
(2)
3.2.3. Zeta potential
Higher negative zeta potential ensuring stability profile of the formulation. As per M. Mady et al. [Citation73] the negative zeta potential of Fluorouracil-encapsulated poly(lactic-co-glycolic acid) and polycaprolactone nanoparticles were −17.47 ± 1.88 mV and −14.22 ± 0.47 mV, respectively. However, after coating with chitosan, the nanoparticles have a positive zeta potential of 15.21 ± 1.72 mV and 12.08 ± 1.87 mV, indicating that the chitosan is having cationic nature. Furthermore, the anionic nature of the Fluorouracil encapsulated polycaprolactone nanoparticles would justify the current experiment's anionic zeta potential results mentioned in . The zeta potential value ranged from −20.76 ± 2.17 mV to −29.74 ± 4.42 mV respectively. From it can be confirmed that all the variables are having significant effects on zeta potential (p > 0.05). The zeta potential of Indomethacin loaded Polycaprolactone nanoparticles produced by Waisudin [Citation74] ranges from −19 to −13 mV at pH 5 and 1 mM NaCl. Under three temperatures of 4 °C, 25 °C, and 40 °C, the produced nanoparticle dispersions were found to be stable for 30 days. These findings suggest that Polycaprolecton nanoparticles could be stable when the zeta potential is projected below −20 mV.
From it can be confirmed that increased concentration of PCL (1–3%) could increase negative zeta potential. This is because polycaprolactone (PCL) is basically an anionic polymer. Nevertheless, it was also understood that increased concentration of PVA increased negative zeta potential. The data retrieved from the zeta potential experiment were subjected to multiple regression analysis and fitted into polynomial quadratic equation No. 3.
(3)
(3)
3.2.4. Encapsulation efficiency
From it can be understood that homogenization speed (A), PVA concentration (B) and PCL concentration (C) have significant effects (p < 0.05) on encapsulation efficiency. From it can be recognised that increasing PVA and PCL concentration would have synergistic effects on encapsulation efficiency. In the presence of higher PVA and PLC concentration, the encapsulation efficiency of the formulation decreases when homogenization speed decreases; this phenomenon might be due to the higher particulate formation within nanoparticles in low homogenization speed. The polynomial quadratic equation No. 4 can be written as:
(4)
(4)
3.3. Determination of optimal formulation
Using analysis of variance (ANOVA), Gefitinib loaded polycaprolactone (PCL) nanoparticles were analysed and using F-test all the parameters were evaluated. The yellow colour area of the overly plot in denotes design space. The input factors responsible for generating predicted variables in design space were shown in . reflects the desirable ranges for each response (input and outcome variables). The overall desirability of the design was found to be 0. 892.The optimized nanoparticles should have minimum particle size, minimum PDI, higher encapsulation efficiency and higher anionic zeta potential. The design has been incepted as per permselective criteria. The relationship between predicted values and actual responses from the optimised batch was shown in .
Table 4. The experimental and predicted values of the optimum Gefitinib loaded polycaprolactone (PLC) nanoparticles.
3.4. Characterization of formulations
To check the effects of different molecular weight of polymer on in-vitro characteristics of Gefitinib loaded polycaprolactone (PCL) nanoparticles, the optimized nano formulation was further prepared using polycaprolactone (PCL) with three different molecular weights Viz., polycaprolactone (PCL) with average 10,000 Da molecular weight (Gefitinib-PCL10,000NPs), polycaprolactone (PCL) with average 45,000 Da molecular weight (Gefitinib-PCL45,000NPs) and polycaprolactone (PCL) with average 80,000 Da molecular weight (Gefitinib-PCL80,000NPs). From it can be postulate that, due to the increase in molecular weight of PCL, viscosity of the formulation increased and therefore higher particle size was obtained. At specified homogenization speed and lower molecular weight of PCL, the resultant emulsion droplets were modest, thus the mean particle size became smaller. A strange phenomenon was observed when molecular weight of PCL increased from 45,000 Da to 80,000 Da. The particle size, encapsulation efficiency and drug loading capacity of the nanoparticles drastically decreased from 286.38 ± 9.27 nm to 246.16 ± 4.62 nm, 68.23 ± 8.32% to 64.51 ± 4.84%, and 4.27 ± 4.11% to 3.94 ± 3.11% (p < 0.05). Due to increased molecular weight of polycaprolactone (PCL), the physicochemical behaviour of the drug changes and therefore it might be possible to have considerable interaction between hydrophobic polymer chain and the drug moiety. Moreover, increased molecular weight of PCL could decrease the diffusion rate of the solvent in external aqueous phase, resulting in lower encapsulation efficacy of the drug. Ozgur Esim et al. (2020) reported similar outcomes when they prepared oxaliplatin loaded PCL nanoparticles using emulsification solvent evaporation method [Citation36].
Table 5. Gefitinib loaded polycaprolactone (PLC) nanoparticles prepared with different Molecular weight of Polycaprolactone (PCL).
The size and shape of the prepared polymeric nanoparticles were found to be spherical and monodispersed. The particle size determined by SEM had a good corelation with particle size obtained from Delsa Nano C photon correlation spectroscopy (PCS). The particles' anionic nature, as a consequence of their negative zeta potential, aids in particle separation, which was clearly apparent in . TEM images () of different molecular weights of PCL showing polymeric nanocomposites with a good spherical form, indicating a good correlation with SEM results. Polycaprolactone (PCL) nanoparticles of three different molecular weights were evaluated using atomic force microscopy (AFM) for skewness (0.238, 0.298, 0.270), average roughness (3.023 nm, 4.167 nm, 3.924), and kurtosis (0.785, 0.812, 0.793). Pretilachlor-loaded polycaprolactone nanocapsules were prepared by Marjan [Citation75]. The nanoparticles particles had a narrow distribution size without aggregation, according to AFM analysis. The results of Gefitinib-loaded PCL nanoparticles were validated by such research findings [Citation75]. Furthermore, the spherical and uniform nature of the three nanoparticles (Gefitinib-PCL10,000NPs, Gefitinib-PCL45,000NPs, Gefitinib-PCL80,000NPs) confirmed by AFM results ().
Figure 2. (a–c) Scanning electron microscopeic (SEM) images of Gefitinib-PCL10,000NPs, Gefitinib-PCL45,000NPs and Gefitinib-PCL80,000NPs respectively. (d–f) Transmission electron microscopy (TEM) images of Gefitinib-PCL10,000NPs, Gefitinib-PCL45,000NPs and Gefitinib-PCL80,000NPs respectively. (g–i) Atomic force microscopy (AFM) images of Gefitinib-PCL10,000NPs, Gefitinib-PCL45,000NPs and Gefitinib-PCL80,000NPs respectively. (j) Nanoparticles suspension of Gefitinib-PCL10,000NPs, Gefitinib-PCL45,000NPs and Gefitinib-PCL80,000NPs. (k) Percentage cumulative drug delivery release from Gefitinib, Gefitinib-PCL10,000NPs, Gefitinib-PCL45,000NPs and Gefitinib-PCL80,000NPs at pH 5.4 and pH 7.4 respectively. (l) Percentage cumulative drug delivery release from Gefitinib at pH 5.4 and pH 7.4.
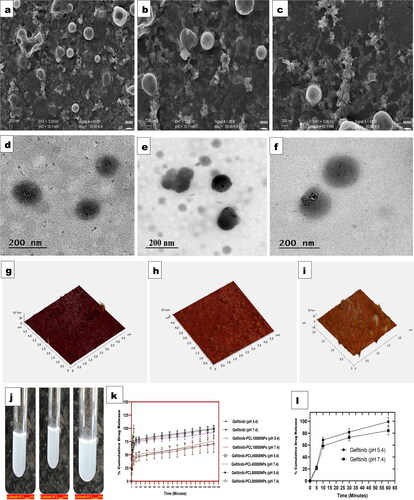
depicts three different kinds of Gefitinib-loaded polycaprolactone (PCL) nanoparticle suspensions.
3.4.1. In vitro drug release studies
In vitro experiments revealed that altering the molecular weight of PCL affects drug release from polymeric nanoparticles. The word molecular weight refers to the length of a polymer's chain and provides information on the polymer's hydrophilicity and lipophilicity. Longer chain lengths enhance the polymer's lipophilicity, resulting in a slower degradation rate (). The release rate and kinetics of the nanoparticles can be alter using different molecular weights of PCL. The presence of free drug molecules adhered to the surface of nanoparticles caused early burst effects in all three formulations within 120 min of initial drug release. According to Mohammed Shahab et al. (2020), biphasic early burst effects within 2 h were seen in chitosan-polycaprolactone nano formulation formulations [Citation76], followed by a sustained released profile. The study also estimates an initial release of 38.56 ± 4.62% % Dorzolamide (DRZ) during the first 2 h of the drug release. According to Nagalakshmi Kamaraj et al. (2017), 12-didehydroandrographolide loaded polycaprolactone nanoparticles exhibit a biphasic drug release pattern, with 20% drug release observed at 24 h, 50% drug release at 192 h, and a sharp decline in drug release at 264 h. Due to the immediate dissolution and release of the drug from the outer surface of the nanoparticles, the prepared polycaprolactone nanoparticles exhibit initial burst effects.
In the present study, it was discovered that early drug release was controlled by diffusion in the polymer metrix, while later drug release was governed by degradation of the polymer metrix and Gefitinib (drug) diffusion within the metrix. Polycaprolactone with three distinct molecular weights (varying from 10,000 to 80,000 Da) to a nanoparticles composite may substantially reduce Gefitinib release. In 2160 min or 36 h, Gefitinib-PCL10,000NPs, Gefitinib-PCL45,000NPs & Gefitinib-PCL80,000NPs shows 71.08 ± 20.04%, 76.22 ± 11.13% & 70.31 ± 9.34% release of total Gefitinib in pH 7.4 phosphate buffer solution, however at 36 h Gefitinib-PCL10,000NPs, Gefitinib-PCL45,000NPs & Gefitinib-PCL80,000NPs shows 92.78 ± 5.56%, 98.78 ± 5.24% and 99.78 ± 3.19% release of total Gefitinib in pH 5.4 phosphate buffer solution. In addition, the cumulative release of Gefitinib from Gefitinib-PCL10,000NPs, Gefitinib-PCL45,000NPs & Gefitinib-PCL80,000NPs was significantly higher at pH 5.4 (p < 0.01) compared with pH 7.4, implying that Gefitinib release in tumour tissue and endosomal conditions could be more efficient and controlled. According to earlier research, biodegradable polymers with a lower molecular weight, utilised in nanoparticles degrade quickly. In addition, as the molecular weight of the polymer increases, so does its lipophilicity, restricting the drug's release profile. Furthermore, bigger nanoparticle particle sizes restrict the penetration of buffer solution into the surface area of the nanoparticles, resulting in delayed drug release. The drug release date fitted into first-order, zero-order, and Higuchi equations to determine release kinetics. The results show that Gefitinib release from Polycaprolactone followed the Higuchi model, and that drug release regression coefficients were better than zero and first order kinetics ().
Table 6. Different mathematical models' correlation coefficients and the release rate constant (k) for Gefitinib from PCL nanoparticles.
3.4.2. Stability of Gefitinib loaded polycaprolactone (PCL) nanoparticles
Stability is a major issue when it comes to nanoparticles, since it has a direct impact on the therapeutic index of the product. As per , it can be confirmed that the particle size and PDI of the Gefitinib loaded polycaprolactone (PCL) nanoparticles were 202.67 ± 6.27 nm and 0.210 ± 0.021 for Gefitinib-PCL10,000 NPs, 286.38 ± 9.27 nm and 0.196 ± 0.034 for Gefitinib-PCL45,000 NPs, 246.16 ± 4.62 nm and 0.205 ± 0.022 for Gefitinib-PCL80,000 NPs. The prepared nanoparticles were having stability for 30 days with no significant changes (p > 0.05).
3.4.3. Hemocompatibility of Gefitinib loaded polycaprolactone (PCL) nanoparticles
To understand more about the effect of Gefitinib loaded polycaprolactone (PCL) nanoparticles on red blood cells, hemocompatibility or haemolysis experiments were performed. According to Qian [Citation77], the amphiphilic block copolymer methoxy polyethyleneglycol-polycaprolactone (mPEG-PCL) can be evaluated for hemocompatibility because it has potential biomedical applications [Citation77]. Due to the obvious inhibition of the arachidonic acid pathway, higher concentrations of mPEG-PCL may cause blood clotting and platelet aggregation. The importance of a hemocompatibility test for injectable methotrexate-loaded polycaprolactone microspheres (MTX-PCL-MPs) was highlighted by Mukesh [Citation78]. RBCs were treated with various concentrations of MTX-PCL MPs (0.5 mg/mL, 1.0 mg/mL, 2.5 mg/mL, 0.5 mg/mL) in this study. Because it had no effect on the morphology of RBCs with developed MTX-PCL-MPs, the results suggest that polycaprolactone is hemocompatible. As a result, they can be used as a localized injectable system for in vivo drug delivery.
One thing is clear from the above-mentioned experimental interpretation: PCL is hemocompatible; however, higher concentrations of PCL can cause hemolysis.
shows erythrocyte solutions treated with 1%triton X-100 as the positive control, untreated erythrocyte solutions as the negative control, and Gefitinib, placebo, and Gefitinib loaded polycaprolactone (PCL) nanoparticles as the treatment. All three polymeric nanoparticles, i.e. Gefitinib-PCL10,000NPs, Gefitinib-PCL45,000NPs, and Gefitinib-PCL80,000NPs showed >0.5% haemolysis and were determined to be safe for RBC membrane integrity, as shown in . There was less haemolysis reported in Gefitinib loaded polycaprolactone (PCL) nanoparticles, due to good Gefitinib encapsulation within the nanoparticles, which protects red blood cells from Gefitinib-induced haemolysis. The haemolysis experiments have shown that i.v. injection of Gefitinib loaded polycaprolactone (PCL) has a favourable effect on erythrocytes. As a result, there's a good chance that malondialdehyde (MDA) levels and carbonyl group content would drop in blood. As a result, when administering intravenously, all three Gefitinib-loaded polycaprolactone (PCL) nanoparticles would may prevent erythrocyte degradation and decrease oxidative stress.
Figure 3. (a). Indicates the visual compirison of humna blood after incorporation of Gefitinib, Gefitinib loaded PCL nanoparticles, placebo in human blood, in the presence of positive and negative control. (b–d). Indicates, Haemolysis (%) profile of Gefitinib, Placebo, Gefitinib PCL10,000NPs, Gefitinib PCL45,000NPs, Gefitinib PCL80,000NPs after individual 100 µg/mL treatment. (e). The number of platelets counts after treatment with Phosphate buffer solution, Gefitinib, Placebo, Gefitinib PCL10,000NPs, Gefitinib PCL45,000NPs, Gefitinib PCL80,000NPs at different concentrations (10, 50 and 100 µg/mL) as per Bonferroni’s multiple comparisons test (values are represented as mean ± SD (n = 3)), *** indicates high statistical significance (p < 0.001) and # indicates insignificance (p = 0.245) as compared to the different concentrations of Gefitinib all the formulations were compared against with treated controlled group (Gefitinib treatment). (f) Light microscopy images of platelets with different treatments at prescribed concentration. (g–i) Amount of LDH release after treating with different concentration (10 µg/mL,50 µg/mL,100µg/mL) of PBS, Gefitinib, placebo, Gefitinib PCL10,000NPs, Gefitinib PCL45,000NPs, Gefitinib and PCL80,000NPs against Triton X 100 at 8th hour.
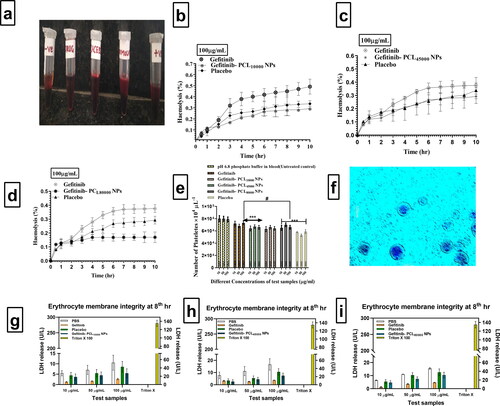
3.4.4. Platelet aggregation study results
After receiving nanoparticles via intravenous (i.v.) route, patients may suffer myocardial infarction, ischemia, or stroke; these issues may occur as a result of platelet aggregation, which leads to thrombus formation in the blood. As a consequence, platelet aggregation must be evaluated following i.v. administration of Gefitinib, placebo, and Gefitinib loaded polycaprolactone (PCL) nanoparticles, i.e. Gefitinib-PCL10,000NPs, Gefitinib-PCL45,000NPs, and Gefitinib-PCL80,000NPs, respectively.
According to Mona Lisa Simionatto Gomesa et al. (2019), cilostazol encapsulated poly(-caprolactone)-poly(ethylene glycol) blend nanocapsules inhibited platelet aggregation more effectively than free cilostazol (p < 0.001) after 24 h than free cilostazol. This could be due to the drug's controlled release rate, which keeps platelet aggregation at bay for longer [Citation79]. According to the above-mentioned research interpretation, PCL could control drug release, lowering the risk of platelet aggregation. Platelets were quantified using a haematological counter after being incubated for 3 h at 37 °C with Gefitinib, placebo, and three Gefitinib-loaded polycaprolactone (PCL) nanoparticles at 10, 50, and 100 g/mL concentrations. The pH 6.4 phosphate buffer was used as a stand-alone control group throughout the research. Gefitinib did not exhibit any significant changes in platelet count when compared to control pH 6.4 phosphate buffer (PBS) solution at any concentration, indicating that Gefitinib did not cause platelet aggregation (). The platelet count in the Placebo group was substantially lower (p < 0.005) than in the Gefitinib, Gefitinib-PCL10,000NPs, Gefitinib-PCL45,000NPs, and Gefitinib-PCL80,000NPs groups. However, when 100 µg/mL Gefitinib and Gefitinib-PCL10,000NPs, Gefitinib-PCL45,000NPs, and Gefitinib-PCL80,000NP are compared to placebo, platelet aggregation is more significant, indicating a non-significant (p > 0.05) relationship. The absence of Gefitinib, which may affect platelet aggregation, resulted in lower platelet aggregation for placebo. shows light microscopy image of Leishman's-stained whole blood samples following treatment with Gefitinib-PCL 80,000 NP at a dose of 100 µg/mL.
3.4.5. Erythrocyte membrane integrity
In this study, white blood cells and plasma were removed from the blood using centrifugation, and only erythrocytes suspended in normal saline solution were used. Measuring Lactate dehydrogenase (LDH) enzyme concentrations allowed researchers to assess membrane integrity. An abnormality in the membrane integrity of erythrocytes causes an increase in LDH release. In , the amount of LDH released after treating 1 mL of erythrocyte suspension with Phosphate buffer solution, Gefitinib, Gefitinib PCL10,000NPs, Gefitinib PCL45,000NPs, Gefitinib PCL80,000NPs (equivalent to 10, 50, and 100 g/mL of Gefitinib), and Placebo nanoparticles equal to the volume of three different molecular weights of polycaprolactone (PCL) nanoparticles of Gefitinib was mentioned respectively. Surprisingly, after 8 h of incubation, placebo formulations showed no significant increase in LDH in comparison to phosphate buffer treated samples. At such concentrations of test samples, the optioned results indicate that erythrocytes are unaffected. Triton X 100, on the other side, displays extremely high LDH release, indicating annihilation of the total erythrocyte. As a result, Gefitinib PCL10,000NPs, Gefitinib PCL45,000NPs, and Gefitinib PCL80,000NPs would not affect the integrity of erythrocyte membranes, and thus prepared Gefitinib loaded nanoparticles would be safe for intravenous administration in terms of erythrocyte safety.
3.4.6. Cell–growth curve
The growth curves of NCI-H460 cell lines in different polymeric nanoformulation (Gefitinib PCL10,000NPs, Gefitinib PCL45,000NPs, and Gefitinib PCL80,000NPs) concentrations (5, 25, and 75 µg/mL) are shown in . The coating of higher molecular weight polycaprolactone (PCL) over Gefitinib nanoparticles has serious antagonistic effects on NCI-H460 cell growth, as shown in . This phenomenon could be explained by polycaprolactone (PCL) and Gefitinib ability to target overexpressed Interleukin-22 receptor of non-small cell lung cancer (NSCLC) could conceal cancer prognosis. The decline of cells grown at higher concentration (75 µg/mL) and higher molecular weight of polycaprolactone (PCL) conjugated nanoparticles (GefitinibPCL80,000NPs) could be due to nanoparticle aggregation on the cell surface.
Figure 4. Growth curve in the NCI-H460 cells was determined by Trypan Blue (counting dye method). The effects of Gefitinib PCL10,000NPs, Gefitinib PCL45,000NPs, and Gefitinib PCL80,000NPs exposure were evaluated at 4, 24, and 48 h at 5, 25, and 75 µg/mL concentrations and compared to the control cells. Microscopic images of NCI-H460 cell growth with Gefitinib PCL10,000NPs (a), Gefitinib PCL45,000NPs (b), Gefitinib PCL80,000NPs (c) at 75 µg/mL, as well as their cell growth curve at all concentrations. NCI-H460 cell growth was suppressed when 75 µg/mL of Gefitinib PCL80,000NPs were added to the cells; indicating superior anticancer effects of Gefitinib PCL80,000NPs.
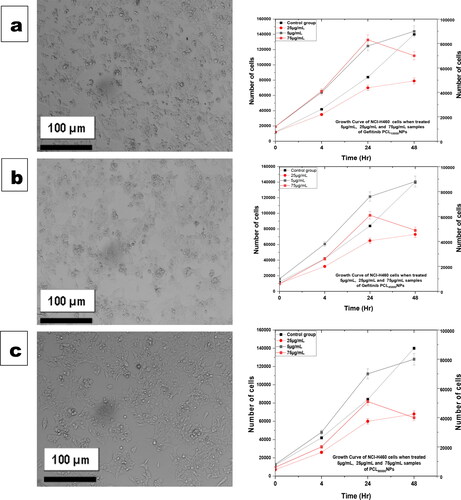
When treated with 75 µg/mL of Gefitinib PCL10,000NPs, Gefitinib PCL45,000NPs, and Gefitinib PCL80,000NPs, NCI-H460 cell growth was reported to have 70,000, 49,000, and 40,000 cells, respectively, in comparison to 140,000 cells of controlled NCI-H460. This indicates that the cell growth was gradually inhibited, with 50%, 35%, and 28.57% inhibition, respectively.
On the contrary, it was discovered that higher concentrations and a longer incubation time could enhance nanoparticle interactions in NCI-H460 cells, with a possible reduction in cell growth.
3.4.7. Cytotoxicity assay
The MTT assay was used to assess the effects of Gefitinib and Gefitinib encapsulated polymeric nanoparticles on cell viability for 24th and 48th hours in NCI-H460 cells. Mohammad M. Badrana et al. (2018) prepared Docetaxel-loaded chitosan-coated PLGA/PCL nanoparticles, and an MTT assay was used to assess cytotoxicity in HT-29 colon cancer cells after 48 h of incubation [Citation80]. The findings suggest that Docetaxel and Docetaxel-loaded chitosan-coated PLGA/PCL nanoparticles have moderate cytotoxicity (11–14%). When HT-29 cells were given Docetaxel-loaded chitosan-coated PLGA/PCL nanoparticles, they died significantly after 48 h. Bio fabricated polycaprolactone nanoparticles transparent membrane for corneal endothelial tissue engineering was prepared by Tahereh [Citation81]. The cytotoxicity of such scaffolds was investigated using the MTT assay [Citation81].
From the MTT assay, one can easily understand that concentration-dependent influences on cell viability can be witnessed. A slight change in cell viability can be seen when drug contraction ranges from 0.25 µM to 5 µM () (p > 0.05); however, concentration above 5 µM shows a significant decrease in cell viability (p > 0.05). Cytotoxicity effects of all the three polymeric nanoparticles, i.e. Gefitinib PCL10,000NPs, Gefitinib PCL45,000NPs, and Gefitinib PCL80,000NPs, increase with the increase of dose when treated for 24th hrs in NCI-H460 cell lines. The cytotoxicity effects of free Gefitinib, placebo nanoparticles, and Gefitinib encapsulated three nanoparticles on NCI-H460 cell lines were evaluated at 24th hrs and 48th hrs. In , the cell viability and expressed NCI-H460 cell after Gefitinib encapsulated three nanoparticles were highlighted. It was observed that all three formulations had better in-vitro anticancer effects. However, Gefitinib PCL80,000NPs shown significant inhalation of NCI-H460 cells after 48th hours of treatment (). This might be due to the formation of strong electrostatic interactions between negative glycocalyx of PCL on the cell membrane. These effects led to increased Gefitinib PCL80,000NPs cell membrane interactions, which facilitates anticancer activity against NCI-H460 cells. From the 24th hr study, it can be assumed that in-vitro anticancer activity of all the formulations shows substantial progression with an increase of drug delivery. At the end of the 48th hour, the NCI-H460 cell viability with Gefitinib and Gefitinib encapsulated three polymeric nanoparticles was found to be 38.67%, 6.78% (Gefitinib PCL10,000NPs), 4.78% (Gefitinib PCL45,000NPs) & 2.78% (Gefitinib PCL80,000NPs), respectively. The findings are strongly suggesting significant cytotoxicity (p < 0.05) of all concentrations of nanoparticles as compared to free-Gefitinib (Positive control) (). The overall increase in cytotoxicity (p < 0.05) may be due to nanoparticle interactions with overexpressed melanoma growth stimulatory activity factor (MGSA) on NCI-H460 cells, which results in increased cytotoxicity. The Gefitinib PCL80,000NPs would release Gefitinib more steadily due to the higher viscosity of PCL80,000, resulting in significant (p < 0.05) cytotoxicity effects within this formulation.
Figure 5. Cell viability studies of free Gefitinib, Placebo, Gefitinib PCL10,000NPs, Gefitinib PCL45,000NPs, Gefitinib PCL80,000NPs, on NCI-H460 cell lines using MTT assay at 24th hours (A–C) & at 48th hours (D–F) (mean ± SD; n = 3, *p ≤ 0.05, **p ≤ 0.01, ***p ≤ 0.001). The NCI-H460 cell behaviour was also can be seen after incorporating Gefitinib PCL10,000NPs, Gefitinib PCL45,000NPs, Gefitinib PCL80,000NPs at 24th and 48th hours.
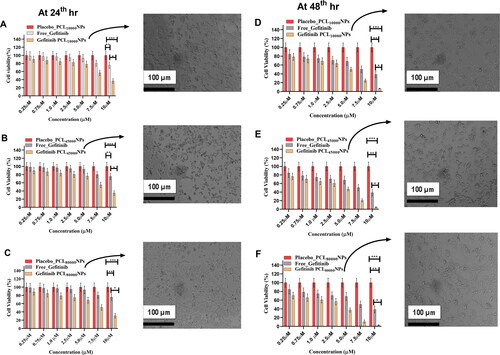
3.4.8. Cellular uptake
A linear drug uptake can be witnessed using passive diffusion within the nanoparticles. From 1st hour to 6th hour, cellular uptake of Gefitinib and three Gefitinib encapsulated nanoparticles was found to be linear (p < 0.05). The cellular NCI-H460 cell uptake depends on the type of nanoparticles administration. From , it is evident that during the 4th to 6th hours, cellular uptake of Gefitinib PCL10,000NPs, Gefitinib PCL45,000NPs, and Gefitinib PCL80,000NPs was found to be significantly higher than free Gefitinib (p < 0.05). However, the particle size, zeta potential, and viscosity of the polymers also can be pivotal for Agilent cellular uptake. The altered molecular weight of the PCL wound not creates a significant difference in cellular uptake at 4th to 6th hours (p < 0.05). Although the decrease in mean particle size with the decreased molecular weight of the polymers was evident in this research, cellular uptake of Gefitinib did not provide significant similarities with existing research. This phenomenon might be due to the vivid polydispersity index of the formulations.
Figure 6. (A) Cellular uptake and (B). cell death analysis of free Gefitinib and Gefitinib, Gefitinib PCL10,000NPs, Gefitinib PCL45,000NPs, Gefitinib PCL80,000NPs (*significantly different from free Gefitinib (p < 0.05)). CLSM images of cellular internalization of Gefitinib PCL80,000NPs into NCI-H460 cell at 2nd (C) and 6th (D) hours of incubation, respectively.
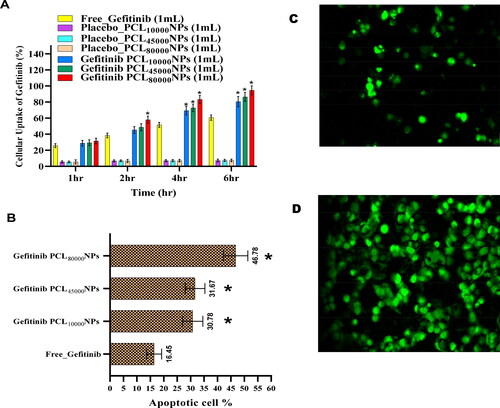
According to Dhwani Jhalaa et al. (2018), Polycaprolactone-chitosan nanofibrous can influence cellular uptake and cytotoxicity of nanoclusters. Biomimetic properties can be found in nanofibrous sets [Citation82]. Their findings show that nanoparticles' surface charge, size, and shape affect the cell cycle, extracellular matrix, and cellular internalization. Various endocytic pathways, cell cycle stage, pinocytosis, and macro-pinocytosis are also involved in proper internalization. According to Batoul [Citation83], Sulfated alginate/polycaprolactone double-emulsion nanoparticles have cellular uptake and wound healing capacity in immortalized primary human adult epidermal cells (HaCaT) [Citation83].
According to these two research findings, because of its ability to mobilize endocytic pathways, cell cycle stage, and pinocytosis process, polycaprolactone (PCL) has good cell internalization and cellular uptake properties. As a result, Gefitinib encapsulated PCL nanoparticles are the best candidates for improving NCI-H460 cell uptake.
3.4.9. In-vitro cellular uptake of fluorescent Gefitinib PCL80,000NPs and cell death analysis
The cellular uptake of free Gefitinib, placeboPCL10,000NPs, placebo PCL45,000NPs, placebo PCL80,000NPs Gefitinib PCL10,000NPs, Gefitinib PCL45,000NPs, and Gefitinib PCL80,000NPs on NCI-H460 cell lines were represented in . The increased cellular uptake of Gefitinib-loaded nanoparticles in NCI-H460 cell lines could be due to the endocytosis process mediated by the CD44 receptor. It's possible that passive Gefitinib transport into the cytoplasm resulted in efflux out by P-glycoprotein (P-gp) pumps, resulting in Gefitinib uptake being limited compared to the three Gefitinib loaded nanoparticles. When Gefitinib nanoparticles are coated with polycaprolactone (PCL), the stealth property of the nanoparticles may be enhanced, and as a result, P-gp may fail to recognize them, resulting in higher uptake of three Gefitinib PCL nanoparticles in NCI-H460 cells, compared to placebo nanoparticles. Before performing confocal laser scanning microscopy (CLSM) (ECLIPSE Ti2, Nikon Corporation, Seongbuk, Japan) to check in-vitro cellular uptake of the nanoparticles, NCI-H460 cells were seeded at a density of 25,000 cells/well and 250 µL of fluorescein diacetate scaffold warm Gefitinib PCL80,000NPs was added into NCI-H460 cells and incubated for 2nd and 6th hours. Using phosphate buffer solution Gefitinib PCL80,000NPs were removed and fixed by 75% v/v ethanol and measured under CLSM. indicates cellular internalization of Gefitinib PCL80,000NPs into cells at 2nd and 6th hours of incubation, respectively. At 6th hour the cellular florescent intensity increases, implying distribution of Gefitinib PCL80,000NPs into the cell cytoplasm by moving forward in time.
When NCI-H460 cells were treated with Gefitinib, phosphatidylserine (PS) translocation was observed. The presence of Annexin V may speed up the apoptotic process, making it easier to identify cell activity from the outer cell surface to the inner plasma membrane. The percentage of apoptosis within the cells was altered when the cells were treated with free Gefitinib, Gefitinib PCL10,000NPs, Gefitinib PCL45,000NPs, and Gefitinib PCL80,000NPs. It was concluded from that the percentage of apoptotic cells incubated with free Gefitinib was 16.45 ± 2.78%. When the NCI-H460 cells were incubated with Gefitinib PCL10,000NPs, Gefitinib PCL45,000NPs, and Gefitinib PCL80,000NPs, the number of apoptotic cells increased to 30.78 ± 3.78%, 31.67 ± 3.67%, and 46.78 ± 4.56%, respectively. When compared to free Gefitinib (p < 0.05), the results clearly showed that Gefitinib encapsulated PCL NPs can greatly increase cell apoptosis. Pro-apoptotic and anti-apoptotic proteins control the modulation of signaling pathways within cells. Gefitinib inhibits tyrosine kinase receptors like epidermal growth factors in a specific manner. As a result, Gefitinib appears to be a successful drug for lung cancer targeted therapy.
The synergistic Cisplatin-Tetradrine combination can be used as an antitumor agent, according to Fangcen [Citation84]. Such drug combinations were encapsulated in the form of poly(ethylene glycol)-polycaprolactone (PEG-PCL) nanoparticles [Citation84]. A fluorescence microscope confirmed that when both hydrophilic and hydrophobic drugs are encapsulated with PCL, the cellular uptake is increased. The PCL encapsulated drug can inhibit tumor metabolic activities with few side effects, and hence formulations may improve Anti-cancer properties. In another experiment, Paclitaxel (PTX) was encapsulated with Folic acid-Pluronic-PCL nanoparticles, according to Yan Chun [Citation85], fluorescence techniques revealed that the formulations had increased cellular uptake and cytotoxicity. The In-vitro Cellular uptake results of Gefitinib-loaded PCL nanoparticles correlated with recently published studies. Therefore, Gefitinib-loaded PCL nanoparticles can be considered the best candidate for cancer targeting [Citation85].
3.4.10. Detection of reactive oxygen species (ROS)
Increased levels of reactive oxygen species (ROS) in the intracellular environment can hasten cell cycle arrest, which leads to apoptosis. As a result, it's crucial to look for ROS in NCI-H460 cells that have been seeded with various concentrations of polymeric nanoparticles. The current experiment was designed to detect possible ROS production in NCI-H460 cells at concentrations of Gefitinib encapsulated nanoparticles solution of 5 µg/mL, 25 µg/mL, and 75 µg/mL, i.e. Gefitinib PCL10,000NPs, Gefitinib PCL45,000NPs, and Gefitinib PCL80,000NPs. In comparison to the negative control group, 5 µg/mL Gefitinib PCL80,000NPs treatment causes significant ROS production in NCI-H460 cells at 5 min (p < 0.0005) and 60 min (p < 0.005). However, the higher the concentration of all polymeric nanoparticles, the lower the production of reactive oxygen species (ROS) (). At the 24th hour, no significant variations in ROS production were observed in any of the formulations. This confirms that prepared nanoparticles can reduce ROS, due to all three Gefitinib PCL-NPs having good cellular internalization and arrest of cellular apoptosis. However, when compared to the other two polymeric nanoparticles, Gefitinib PCL80,000NPs were found to have a low relative fluorescence. This indicates that Gefitinib PCL80,000NPs nanoparticles reduce free ROS production in NCI-H460. As a result, Gefitinib PCL80,000NPs may be the best candidate for treating non-small cell lung cancer (NSCLC).
Figure 7. Detection of reactive oxygen species (ROS) in NCI-H460 cells: Following exposure at 0, 15, 30, 45, 60 min, and after 24 h at 5, 25, and 75 µg/mL concentrations, the effects of Gefitinib PCL10,000NPs (a), Gefitinib PCL45,000NPs (b), Gefitinib PCL80,000NPs (c) on ROS production were evaluated. *p < 0.05; **p < 0.005; ***p < 0.0005; error bars indicate standard error of the mean. After 24th hr it was witnessed that Gefitinib PCL80,000NPs (c) generates limited relative fluorescence; indicating less amount of ROS production in NCI-H460 cells after Gefitinib PCL80,000NPs treatment. In NCI-H460 cells treated with Gefitinib PCL10,000NPs, Gefitinib PCL45,000NPs, Gefitinib PCL80,000NPs, the expression of micronuclei (BNMN), cytokinesis block proliferation index (CBPI), and Nuclear Bud (NBUDs) was measured. After exposure to Gefitinib PCL10,000NPs, Gefitinib PCL45,000NPs, Gefitinib PCL80,000NPs, the CBPI (Cytokinesis Block Proliferation Index) (d), Micronuclei (e), and Nuclear Buds (f) were measured at 5, 25, and 75 µg/mL concentrations. CBPI, ‘Cytokinesis Block Proliferation Index’ Micronuclei, and Nuclear Buds, were measured after exposure to all three PCL nanoparticles of Gefitinib, at 5, 25, and 75 g/mL concentrations. 1000 binucleated cells are equal to 1000 micronuclei. A total of 1000 binucleated cells are referred to as Nuclear Buds. As a positive control, Gefitinib (5 g/mL) was used. *p < 0.05; **p < 0.005; ***p < 0.0005; error bars indicate standard error of the mean. (g) CBMN assay in NCI-H460 cells: DNA damage after Gefitinib PCL80,000NPs treatment cell with micronuclei, and nuclear bud cell with micronuclei (mentioned in black colour arrow) at magnification 400×.
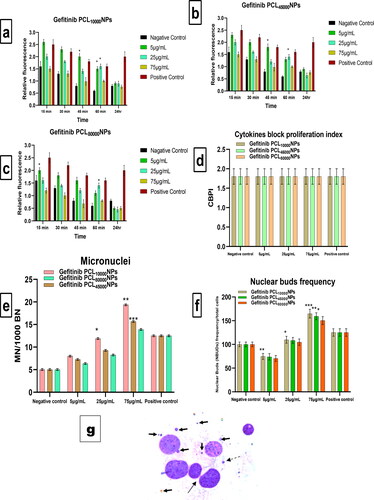
3.4.11. In-vitro Cytokinesis-Block micronucleus (CBMN) assay
The cytokinesis block proliferation index (CBPI) must be determined in order to assess cellular proliferation progression. As a result, the results of the micronucleus test are required. When CBPI was obtained from cells incubated with 5 µg/mL, 25 µg/mL, and 75 µg/mL solutions of individual Gefitinib PCL10,000NPs, Gefitinib PCL45,000NPs, and Gefitinib PCL80,000NPs, respectively, compared to controlled conditions, nonsignificant effects were observed. When treated with different concentrations of polymeric nanoparticles, however, a significant dose-dependent increase in micronucleus was observed. Analysis of nuclear buds (NBUDs), which progenitor as nucleoplasmic material, was also feasible. The results also indicated a significant decrease in all PCL nanoparticles at 5 µg/mL compared to the control group, as well as a 70% increase at 75 µg/mL compared to the control group (). After 5 µg/mL treatment of Gefitinib PCL80,000NPs in NCI-H460 cells, the damage to DNA in the form of micronuclei and NBUDs was clearly visible in .
4. Conclusion
This research work's findings clearly emphasize the formation of Gefitinib-loaded PCL nanoparticles, which have superior anticancer activities against NCI-H460 cell lines. The Box-Behnken design was introduced to establish a relationship between dependent and independent variables and to establish optimum formulation. The PVA concentration (%) and homogenization speed (RPM) have a significant impact on particle size, PDI, and zeta potential (mV). However, particle size increase with the molecular weight increment of PCL from 10,000 to 45,000 Da. The significant burst release of the three Gefitinib-loaded PCL nanoparticles was observed within 120 min. The presence of the free drug on the nanoparticles' outer surface causes this phenomenon. The higher molecular weight of PCL could increase viscosity. Therefore, Gefitinib PCL80,000NPs could have decreased the release rate of the Gefitinib. All three formulations of Gefitinib PCL10,000NPs, Gefitinib PCL45,000NPs, and Gefitinib PCL80,000NPs have excellent cytotoxicity at 24th and 48th hours. The results also suggest that Gefitinib-loaded PCL nanoparticles have significant cytotoxicity against NCI-H460 cell lines. Furthermore, PCL nanoparticles enhance the cellular uptake and apoptosis of Gefitinib. As a result, three PCL polymeric nanoformulations of Gefitinib may have a good control release profile and represent the most promising clinical application for non-small cell lung cancer therapy (NSCLC).
Authors’ contribution statement
Sankha Bhattacharya: Conceptualization; Supervision; Validation; Visualization; Writing—original draft; Writing—review & editing.
Acknowledgements
I'd like to thank Dr. R.S. Gaud, Director, SVKM's Pharma Institutions, SVKM's NMIMS Deemed-to-be University, Maharashtra, India, for providing excellent research facilities and unwavering support throughout this project. DIYA LAB, Mumbai, Maharashtra 400708, India, has also helped me with my studies in scanning electron microscopy (SEM), transmission electron microscopy (TEM), and atomic force microscopy (AFM). Deshpande Laboratories (DL) Pvt. Ltd., Bhopal, India, is also gratefully acknowledged for commercial support for in-vitro toxicity studies. I'd like to thank Bharathidasan University, Tiruchirappalli, Tamil Nadu, India, for allowing me to study Confocal Laser Scanning Microscope (CLSM)
Disclosure statement
There is no conflict of interest reported.
Data availability statement
The data that support the findings of this study are available from the corresponding author, [Sankha Bhattacharya], upon reasonable request.
References
- Löke DR, Helderman RFCPA, Franken NAP, et al. Simulating drug penetration during hyperthermic intraperitoneal chemotherapy. Drug Deliv. 2021;28(1):145–161.
- Manevich Y, Reyes L, Britten CD, et al. Redox signaling and bioenergetics influence lung cancer cell line sensitivity to the isoflavone ME-344. J Pharmacol Exp Ther. 2016;358(2):199–208.
- Mohammadzadeh-Asl S, Aghanejad A, Yekta R, et al. Kinetic and thermodynamic insights into interaction of erlotinib with epidermal growth factor receptor: surface plasmon resonance and molecular docking approaches. Int J Biol Macromol. 2020;163:954–958.
- Passaro A, Mok T, Peters S, et al. Recent advances on the role of EGFR tyrosine kinase inhibitors in the management of NSCLC with uncommon, non exon 20 insertions, EGFR mutations. J Thorac Oncol. 2021;16(5):764–773.
- Qiu WL, Tseng AJ, Hsu HY, et al. Fucoidan increased the sensitivity to Gefitinib in lung cancer cells correlates with reduction of TGFβ-mediated slug expression. Int J Biol Macromol. 2020;153:796–805.
- Ito K, Murotani K, Kubo A, et al. Propensity score analysis of overall survival between first- and second-generation EGFR-TKIs using real-world data. Cancer Sci. 2020;111(10):3705–3713.
- Liu S, Li Q, Li G, et al. The mechanism of m(6)a methyltransferase METTL3-mediated autophagy in reversing Gefitinib resistance in NSCLC cells by β-elemene. Cell Death Dis. 2020;11(11):969.
- Zhang T, Wang R, Li M, et al. Comparative study of intratracheal and oral Gefitinib for the treatment of primary lung cancer. Eur J Pharm Sci. 2020a;149:105352.
- Garizo AR, Castro F, Martins C, et al. p28-functionalized PLGA nanoparticles loaded with Gefitinib reduce tumor burden and metastases formation on lung cancer. J Control Release. 2021;337:329–342.
- Joshi U, Filipczak N, Khan MM, et al. Hypoxia-sensitive micellar nanoparticles for co-delivery of siRNA and chemotherapeutics to overcome multi-drug resistance in tumor cells. Int J Pharm. 2020;590:119915.
- Solanki R, Rostamabadi H, Patel S, et al. Anticancer nano-delivery systems based on bovine serum albumin nanoparticles; a critical review. Int J Biol Macromol. 2021;193:528–540.
- Kola Srinivas NS, Verma R, Pai Kulyadi G, et al. A quality by design approach on polymeric nanocarrier delivery of Gefitinib: formulation, in vitro, and in vivo characterization. Int J Nanomed. 2017;12:15–28.
- Mack JT, Helke KL, Normand G, et al. ABCA2 transporter deficiency reduces incidence of TRAMP prostate tumor metastasis and cellular chemotactic migration. Cancer Lett. 2011;300(2):154–161.
- Liang J, Yang B, Zhou X, et al. Stimuli-responsive drug delivery systems for head and neck cancer therapy. Drug Deliv. 2021;28(1):272–284.
- Velpurisiva P, Rai P. Synergistic action of Gefitinib and GSK41364A simultaneously loaded in ratiometrically-engineered polymeric nanoparticles for glioblastoma multiforme. JCM. 2019;8(3):367.
- Li X, Zhao Z, Yang Y, et al. Novel β-1,3-d-glucan porous microcapsule enveloped folate-functionalized liposomes as a trojan horse for facilitated oral tumor-targeted co-delivery of chemotherapeutic drugs and quantum dots. J Mater Chem B. 2020;8(11):2307–2320.
- Noronha V, Patil VM, Joshi A, et al. Gefitinib versus Gefitinib plus pemetrexed and carboplatin chemotherapy in EGFR-Mutated lung cancer. J Clin Oncol. 2020;38(2):124–136.
- Qi M, Gao S, Nie S, et al. Precise engineering of cetuximab encapsulated gadollium nanoassemblies: in vitro ultrasound diagnosis and in vivo thyroid cancer therapy. Drug Deliv. 2021;28(1):569–579.
- Amirani E, Hallajzadeh J, Asemi Z, et al. Effects of chitosan and oligochitosans on the phosphatidylinositol 3-kinase-AKT pathway in cancer therapy. Int J Biol Macromol. 2020;164:456–467.
- Bharathala S, Singh R, Sharma P. Controlled release and enhanced biological activity of chitosan-fabricated carbenoxolone nanoparticles. Int J Biol Macromol. 2020;164:45–52.
- Chen W, Cheng H, Jiang Q, et al. The characterization and biological activities of synthetic N, O-selenized chitosan derivatives. Int J Biol Macromol. 2021;173:504–512.
- Javed R, Rais F, Kaleem M, et al. Chitosan capping of CuO nanoparticles: facile chemical preparation, biological analysis, and applications in dentistry. Int J Biol Macromol. 2021;167:1452–1467.
- Liu J, Zhang X, Kennedy JF, et al. Chitosan induces resistance to tuber rot in stored potato caused by alternaria tenuissima. Int J Biol Macromol. 2019;140:851–857.
- Qu B, Luo Y. A review on the preparation and characterization of chitosan-clay nanocomposite films and coatings for food packaging applications. Carbohydr Polym Technol Appl. 2021;2:100102.
- Wusigale WT, Hu Q, Xue J, et al. Partition and stability of folic acid and caffeic acid in hollow zein particles coated with chitosan. Int J Biol Macromol. 2021;183:2282–2292.
- Faraji Dizaji B, Hasani Azerbaijan M, Sheisi N, et al. Synthesis of PLGA/chitosan/zeolites and PLGA/chitosan/metal organic frameworks nanofibers for targeted delivery of paclitaxel toward prostate cancer cells death. Int J Biol Macromol. 2020;164:1461–1474.
- Zhang Z, Li D, Ma X, et al. Carboxylated nanodiamond-mediated NH2-PLGA nanoparticle-encapsulated fig polysaccharides for strongly enhanced immune responses in vitro and in vivo. Int J Biol Macromol. 2020b;165(Pt A):1331–1345.
- Bhattacharya S. Fabrication of poly(sarcosine), poly (ethylene glycol), and poly (lactic-co-glycolic acid) polymeric nanoparticles for cancer drug delivery. J Drug Delivery Sci Technol. 2021;61:102194.
- Reys LL, Silva SS, Oliveira C, et al. Angiogenic potential of airbrushed fucoidan/polycaprolactone nanofibrous meshes. Int J Biol Macromol. 2021;183:695–706.
- Shirzaei Sani I, Rezaei M, Baradar Khoshfetrat A, et al. Preparation and characterization of polycaprolactone/chitosan-g-polycaprolactone/hydroxyapatite electrospun nanocomposite scaffolds for bone tissue engineering. Int J Biol Macromol. 2021;182:1638–1649.
- Aga MB, Dar AH, Nayik GA, et al. Recent insights into carrageenan-based bio-nanocomposite polymers in food applications: a review. Int J Biol Macromol. 2021;192:197–209.
- Ribeiro IS, Pontes FJG, Carneiro MJM, et al. Poly(ε-caprolactone) grafted cashew gum nanoparticles as an epirubicin delivery system. Int J Biol Macromol. 2021;179:314–323.
- Simpson A. Retraction: polycaprolactone composites with TiO2 for potential nanobiomaterials: tunable properties using different phases. Phys Chem Chem Phys. 2021;23(15):9612–9612.
- Pan Q, Tian J, Zhu H, et al. Tumor-Targeting polycaprolactone nanoparticles with codelivery of paclitaxel and IR780 for combinational therapy of drug-resistant ovarian cancer. ACS Biomater Sci Eng. 2020;6(4):2175–2185.
- Najaf Oshani B, Davachi SM, Hejazi I, et al. Enhanced compatibility of starch with poly(lactic acid) and poly(ɛ-caprolactone) by incorporation of POSS nanoparticles: study on thermal properties. Int J Biol Macromol. 2019;141:578–584.
- Esim O, Bakirhan NK, Yildirim N, et al. Development, optimization and in vitro evaluation of oxaliplatin loaded nanoparticles in non-small cell lung cancer. Daru. 2020a;28(2):673–684.
- Alshetaili AS. Gefitinib loaded PLGA and chitosan coated PLGA nanoparticles with magnified cytotoxicity against A549 lung cancer cell lines. Saudi J Biol Sci. 2021;28(9):5065–5073.
- Diwan R, Ravi PR, Agarwal SI, et al. Cilnidipine loaded poly (ε-caprolactone) nanoparticles for enhanced oral delivery: optimization using DoE, physical characterization, pharmacokinetic, and pharmacodynamic evaluation. Pharm Dev Technol. 2021;26(3):278–290.
- Zuppolini S, Maya IC, Diodato L, et al. Self-associating cellulose-graft-poly(ε-caprolactone) to design nanoparticles for drug release. Mater Sci Eng C Mater Biol Appl. 2020;108:110385.
- El-Habashy SE, Eltaher HM, Gaballah A, et al. Hybrid bioactive hydroxyapatite/polycaprolactone nanoparticles for enhanced osteogenesis. Mater Sci Eng C Mater Biol Appl. 2021;119:111599.
- El Fawal G, Abu-Serie MM, Mo X, et al. Diethyldithiocarbamate/silk fibroin/polyethylene oxide nanofibrous for cancer therapy: fabrication, characterization and in vitro evaluation. Int J Biol Macromol. 2021;193:293–299.
- Zaichik S, Steinbring C, Jelkmann M, et al. Zeta potential changing nanoemulsions: impact of PEG-corona on phosphate cleavage. Int J Pharm. 2020;581:119299.
- Mendes LP, Sarisozen C, Torchilin VP. Physiological barriers in cancer: a challenge to be overcome functional lipid nanosystems in cancer. Singapore: Jenny Stanford Publishing; 2021. p. 3–43.
- Subhan MA, Torchilin VP. Neutrophils as an emerging therapeutic target and tool for cancer therapy. Life Sci. 2021;285:119952.
- Zhang Y, Zhang Q, Sun J, et al. The combination therapy of salinomycin and Gefitinib using poly(d,l-lactic-co-glycolic acid)-poly(ethylene glycol) nanoparticles for targeting both lung cancer stem cells and cancer cells. Onco Targets Ther. 2017;10:5653–5666.
- Szczęch M, Szczepanowicz K. Polymeric core-shell nanoparticles prepared by spontaneous emulsification solvent evaporation and functionalized by the layer-by-layer method. Nanomaterials. 2020;10(3):496.
- Dou M, Lu C, Sun Z, et al. Natural cryoprotectants combinations of l-proline and trehalose for red blood cells cryopreservation. Cryobiology. 2019;91:23–29.
- Yang H, Yang S, Kong J, et al. Obtaining information about protein secondary structures in aqueous solution using Fourier transform IR spectroscopy. Nat Protoc. 2015;10(3):382–396.
- Sarkar P, Bhattacharya S, Pal TK. Application of statistical design to evaluate critical process parameters and optimize formulation technique of polymeric nanoparticles. R Soc Open Sci. 2019;6(7):190896.
- Picarazzi S, Bergamaschi D, Tavazzi S. Differences between tears of contact lens wearers studied by photon correlation spectroscopy. Cont Lens Anterior Eye. 2019;42(2):212–215.
- Muhamad N, Plengsuriyakarn T, Chittasupho C, et al. The potential of Atractylodin-Loaded PLGA nanoparticles as chemotherapeutic for cholangiocarcinoma. Asian Pac J Cancer Prev. 2020;21(4):935–941.
- Ozaki H, Nakano Y, Sakamaki H, et al. Basic eluent for rapid and comprehensive analysis of fatty acid isomers using reversed-phase high performance liquid chromatography/Fourier transform mass spectrometry. J Chromatogr A. 2019;1585:113–120.
- Basak S, Mondal S, Dey S, et al. Fabrication of β-cyclodextrin-mediated single bimolecular inclusion complex: characterization, molecular docking, in-vitro release and bioavailability studies for Gefitinib and simvastatin conjugate. J Pharm Pharmacol. 2017;69(10):1304–1317.
- Dhiman V, Patil K, Velip L, et al. Comprehensive degradation profiling and influence of different oxidising reagents on tinoridine hydrochloride: structural characterization of its degradation products using HPLC and HRMS. Rapid Commun Mass Spectrom. 2022;36(1):e9210.
- Afzalipour R, Khoei S, Khoee S, et al. Thermosensitive magnetic nanoparticles exposed to alternating magnetic field and heat-mediated chemotherapy for an effective dual therapy in rat glioma model. Nanomed Nanotechnol Biol Medi. 2021;31:102319.
- Barshtein G, Arbell D, Yedgar S. Hemolytic effect of polymeric nanoparticles: role of albumin. IEEE Trans Nanobiosci. 2011;10(4):259–261.
- Smyth E, Solomon A, Vydyanath A, et al. Induction and enhancement of platelet aggregation in vitro and in vivo by model polystyrene nanoparticles. Nanotoxicology. 2015;9(3):356–364.
- Lin L, Yang L, Chen J, et al. High-molecular-weight fucosylated glycosaminoglycan induces human platelet aggregation depending on α(IIb)β(3) and platelet secretion. Platelets. 2021;32(7):975–983.
- Farhana A, Lappin SL. Biochemistry, lactate dehydrogenase. Treasure Island (FL): StatPearls Publishing; 2021.
- Guo Y, Wang D, Song Q, et al. Erythrocyte Membrane-Enveloped polymeric nanoparticles as nanovaccine for induction of antitumor immunity against melanoma. ACS Nano. 2015;9(7):6918–6933.
- Raval N, Maheshwari R, Shukla H, et al. Multifunctional polymeric micellar nanomedicine in the diagnosis and treatment of cancer. Mater Sci Eng C Mater Biol Appl. 2021;126:112186.
- Subhan MA, Yalamarty SSK, Filipczak N, et al. Recent advances in tumor targeting via EPR effect for cancer treatment. JPM. 2021;11(6):571.
- Mosmann T. Rapid colorimetric assay for cellular growth and survival: application to proliferation and cytotoxicity assays. J Immunol Methods. 1983;65(1–2):55–63.
- Xu YL, Jiang XM, Zhang LL, et al. Establishment and characterization of pemetrexed-resistant NCI-H460/PMT cells. ACAMC. 2019;19(6):731–739.
- van Gisbergen MW, Cebula M, Zhang J, et al. Chemical reactivity window determines prodrug efficiency toward glutathione transferase overexpressing cancer cells. Mol Pharm. 2016;13(6):2010–2025.
- Grek C, Townsend DM. Protein disulfide isomerase superfamily in disease and the regulation of apoptosis. Endoplasmic Reticulum Stress Dis. 2014;1(1):4–17.
- Kumar R, Saneja A, Panda AK. An annexin V-FITC-Propidium Iodide-Based method for detecting apoptosis in a Non-Small cell lung cancer cell line. Methods Mol Biol (Clifton, NJ). 2021;2279:213–223.
- Shah P, Sarolia J, Vyas B, et al. PLGA nanoparticles for nose to brain delivery of clonazepam: formulation, optimization by 32 factorial design. Curr Drug Deliv. 2021;18(6):805–824.
- Esim O, Savaser A, Ozkan CK, et al. Nose to brain delivery of eletriptan hydrobromide nanoparticles: preparation, in vitro/in vivo evaluation and effect on trigeminal activation. J Drug Delivery Sci Technol. 2020b;59:101919.
- Vollrath A, Kretzer C, Beringer-Siemers B, et al. Effect of crystallinity on the properties of polycaprolactone nanoparticles containing the dual FLAP/mPEGS-1 inhibitor BRP-187. Polymers. 2021;13(15):2557.
- Ajiboye AL, Trivedi V, Mitchell JC. Preparation of polycaprolactone nanoparticles via supercritical carbon dioxide extraction of emulsions. Drug Deliv Transl Res. 2018;8(6):1790–1796.
- Omarch G, Kippie Y, Mentor S, et al. Comparative in vitro transportation of pentamidine across the blood-brain barrier using polycaprolactone nanoparticles and phosphatidylcholine liposomes. Artif Cells Nanomed Biotechnol. 2019;47(1):1428–1436.
- Mady MF, Bagi A, Kelland MA. Synthesis and evaluation of new bisphosphonates as inhibitors for oilfield carbonate and sulfate scale control. Energy Fuels. 2016;30(11):9329–9338.
- Badri W, Miladi K, Robin S, et al. Polycaprolactone based nanoparticles loaded with indomethacin for anti-Inflammatory therapy: from preparation to ex vivo study. Pharm Res. 2017;34(9):1773–1783.
- Diyanat M, Saeidian H, Baziar S, et al. Preparation and characterization of polycaprolactone nanocapsules containing pretilachlor as a herbicide nanocarrier. Environ Sci Pollut Res Int. 2019;26(21):21579–21588.
- Rahat I, Imam SS, Rizwanullah M, et al. Thymoquinone-entrapped chitosan-modified nanoparticles: formulation optimization to preclinical bioavailability assessments. Drug Deliv. 2021;28(1):973–984.
- Hu Q, Zhang Y, Wang C, et al. Hemocompatibility evaluation in vitro of methoxy polyethyleneglycol-polycaprolactone copolymer solutions. J Biomed Mater Res A. 2016;104(3):802–812.
- Dhanka M, Shetty C, Srivastava R. Injectable methotrexate loaded polycaprolactone microspheres: physicochemical characterization, biocompatibility, and hemocompatibility evaluation. Mater Sci Eng C Mater Biol Appl. 2017;81:542–550.
- Gomes MLS, da Silva Nascimento N, Borsato DM, et al. Long-lasting anti-platelet activity of cilostazol from poly(ε-caprolactone)-poly(ethylene glycol) blend nanocapsules. Mater Sci Eng C Mater Biol Appl. 2019;94:694–702.
- Badran MM, Alomrani AH, Harisa GI, et al. Novel docetaxel chitosan-coated PLGA/PCL nanoparticles with magnified cytotoxicity and bioavailability. Biomed Pharmacother. 2018;106:1461–1468.
- Tayebi T, Baradaran-Rafii A, Hajifathali A, et al. Biofabrication of chitosan/chitosan nanoparticles/polycaprolactone transparent membrane for corneal endothelial tissue engineering. Sci Rep. 2021;11(1):7060.
- Jhala D, Rather H, Kedaria D, et al. Biomimetic polycaprolactone-chitosan nanofibrous substrate influenced cell cycle and ECM secretion affect cellular uptake of nanoclusters. Bioact Mater. 2019;4(1):79–86.
- Maatouk B, Jaffa MA, Karam M, et al. Sulfated alginate/polycaprolactone double-emulsion nanoparticles for enhanced delivery of heparin-binding growth factors in wound healing applications. Colloids Surf B Biointerfaces. 2021;208:112105.
- Liu F, Wang X, Liu Q, et al. Biocompatible nanoparticles as a platform for enhancing antitumor efficacy of cisplatin–tetradrine combination. Nanoscale Res Lett. 2021;16(1):61.
- Gong YC, Xiong XY, Ge XJ, et al. Effect of the folate ligand density on the targeting property of Folated-Conjugated polymeric nanoparticles. Macromol Biosci. 2019;19(2):e1800348.