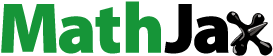
Abstract
Cobalt oxide/graphene oxide (Co3O4/GO) nanocomposites were synthesised using the co-precipitation synthesis process. The polycrystalline nature of Co3O4 nanoparticles onto GO sheets is studied by X-ray diffraction (XRD) pattern where nanoparticles were found in polycrystalline nature with a particle size of 35 nm. The structural and morphological were using field emission scanning electron microscopy (FESEM) and EDX, where a distribution of Co3O4 nanoparticles on GO nanosheets was observed. The effect of Co3O4 nanoparticles on GO nanosheets was studied using a Raman spectrometer and found enhancement in the Raman peaks of GO sheets after the decoration of Co3O4 nanoparticles on GO nanosheets. It is observed that the ID/IG ratio of the D and G bands was increased from 1.08 (GO) to 1.11 (Co3O4/GO). In our present study, we explored the potential cytotoxic effects of Co3O4/GO nanocomposites in Mice melanoma cells (B16F10), where MTT assay suggested that (Co3O4/GO) nanocomposite shows a significant effect on cell viability compared to GO i.e. 60% cell viability was observed at 200 µg/mL of GO whereas it was only 37% for Co3O4/GO nanocomposite indicating improved anti-cancerous activity at this concentration. This is the first time that Co3O4/GO nanocomposite is tested for its cytotoxic effect and the results suggest that it can be used as an alternative source for tumour or cancer treatment.
1. Introduction
Rapidly growing cancer cases and the mortality rate is a subject of huge concern worldwide, especially in countries where population density is high and socioeconomic status is low. GLOBOCAN 2018, predicted that the number of incidences and mortality cases in 2018 was 18.1 million and estimated to be 29.5 million by 2040. shows the number of new cases and deaths reported worldwide in 2018 including both sexes and all ages [Citation1, Citation2].
Figure 1. The number of new cases and deaths reported worldwide in 2018 including both sexes and all ages.
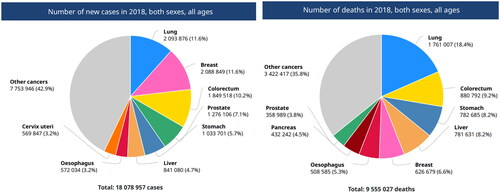
Medical technologies for the diagnosis and treatment of cancers have developed in past decades, still, the mortality rate is increasing which indicates the need for better treatment modalities to control this burden. In recent years, significant research has been conducted in the field of nanotechnology for its medical applications i.e. nanomedicine, including various types of nanomaterials for efficient diagnostics and treatment of cancer. Earlier, nanomaterials were used for the diagnosis of cancer and drug delivery system for cancer drugs but recently various organic and inorganic nanomaterials have been explored as anti-cancerous agents [Citation3, Citation4].
Organic nanomaterials for cancer therapies (natural or synthetic polymer-formed) are liposomes, polymeric nanoparticles, polymeric micelles, dendrimers, and polymer-drug conjugates which possess excellent biological compatibility and degradability. Further, inorganic nanomaterials for cancer therapies with unique chemical and physical properties include: silica nanoparticles, carbon-based nanomaterials, nanographene, gold nanoparticles, magnetic nanoparticles and quantum dots () adapted from [Citation5, Citation6]. Among all nanomaterials metal nanoparticles have been reported for various applications such as anti-microbial [Citation7–9] photocatalyst for wastewater treatment [Citation10, Citation11] dye degradation [Citation12], drug delivery [Citation8], and other biomedical applications including cancers [Citation8, Citation13]. Recent anti-cancerous studies of various metal and metal oxide nanoparticles have been reported such as iron oxide (Fe3O4) nanoparticles have been demonstrated to persuade cell death and damage of DNA in human breast cancer cells [Citation14]. Silver nanoparticles (AgNPs) (including physical, chemical, and biological) have been studied extensively for various biomedical applications including anti-cancerous properties. However, in vitro cell cultivation tests result indicated that AgNPs are toxic to several human cell lines such as immortal human keratinocytes, red blood cells, human peripheral blood mononuclear cells, liver cells, human umbilical vein endothelial cells, human bronchial epithelial cells, etc. AgNPs are known to be double-edged blade that kills microorganisms while inducing cytotoxicity in mammalian cells [Citation15].
Table 1. Types of organic and inorganic nanomaterials for cancer therapy and imaging.
Toxicity and degradation of metal nanoparticles still limit their application in biological systems, therefore, formation of nanocomposites via addition of some less toxic organic or inorganic material as substrate can help in overcome this issue. In organic materials carbon-based materials namely fullerenes, carbon nanotubes and graphene are the good examples for nanocomposite substrate [Citation16]. Among these carbon-based materials, graphene has been extensively used in fields like solar cell, sensors and capacitors due to its excellent mechanical, thermal and electronic properties [Citation17–21]. While, the oxide form of graphene i.e. graphene oxide (GO) is the most interesting material these days and more preferred in biomedical applications due to its stability in water and biocompatibility. The layered GO sheets containing sp2 hybridised carbon atoms are generated via oxidation and exfoliation of solid graphite flakes. GO sheets possess functional oxygenated groups: hydroxyl, carbonyl, carboxylic and epoxy gathering, which act to restrain electron move that makes the nuclear layer hydrophilic and extend interlayer separation [Citation7, Citation22–25]. Reduced graphene oxide (RGO) sheets are generated by removal of oxygen functional groups of GO simply by reduction process, however, GO sheets preferred to be used as a progenitor for producing RGO sheets. GO sheets have been used for synthesis of nanocomposites, where different types of nanomaterials such as metallic, semiconducting and magnetic nanoparticles can be grown on these sheets with different shapes and sizes [Citation26–28].
As an interesting study Professor Michael Lisanti and Dr Aravind Vijaya Raghavan, University of Manchester in 2015 shows that GO which is earlier used as drug delivery vehicle can itself act as anti-cancer agent. The study shows that nano sized GO can neutralise cancer stem cells while not harming other cells [www.manchester.ac.uk], the results were really interesting. However, the anti-cancerous properties of GO are lesser as compared to metal nanoparticles [Citation29]. This motivates researchers to explore GO-metal nanocomposites as potential anti-cancerous agents where GO has been used for creating fewer toxic nanocomposites [Citation30]. Various studies have reported application of GO and its nanocomposites as anti-cancerous agents based on the toxicological mechanism of generation reactive oxygen species (ROS) in cytotoxicity via cell-belting down influence (i.e. cell apoptosis or cell necrosis). When nanoparticles interact with cells it brings change in the ROS production, primarily in mitochondria and affecting antioxidant defense system that induces oxidative stress. ROS formations usually includes the hydroxyl radical (OH·), superoxide radical (O2−) and hydrogen peroxide (H2O2) which damages cellular components and creates events like DNA damage and ultimately apoptotic cell death. Fozia Shaheen et al., 2018 published the outcomes of the GO-ZnO nanocomposite on ROS generation via diverse doses to MCF-7 cell [Citation31]. Similarly, Daoud Ali et al., 2018 reported inhibition of ROS production due to N-acetylcysteine and significant cytotoxic and apoptotic effect of the rGO–Ag nanocomposite in HepG2 and CHANG cells using MTT assay [Citation32]. , shows the comparative analysis of some of the recent works on exploring anti-cancerous activities of GO based nanocomposites.
Table 2. Shows a comparative study of synthesised material with other reported materials for their anti-cancerous activities.
Researches are going on to develop GO nanocomposite with other metal/metal oxide nanoparticles which can be used for biomedical applications [Citation25]. One of the emerging materials is Co3O4 nanoparticles which is studied for the potential cytotoxic and anti-cancerous effects these days. Khan et al., 2015 investigated Co3O4 in human colorectal types of cancerous cells (HT29 and SW620) and several bacteria, however, results show significant anticancer activity but no antibacterial effect [Citation33]. Recently, the cytotoxicity and anticancer properties of Co3O4 nanoparticles functionalised by glutamic acid and conjugated with thiosemicarbazide have been investigated on gastric cancer (AGS) cell line, where MTT test results showed significant cytotoxic effect and anti-cancer activities and suggested its application for the drug delivery system [Citation34]. Rauwel et al., 2020 demonstrated uptake of superparamagnetic cobalt (Co) metal nanoparticle by HCT116 and MCF7 cancer cells and DPSC mesenchymal stem cells using confocal Raman microscopy, opening new routes for cancer phototherapy [Citation35]. MTT assay studied the toxicity of graphene based metal nanocomposites to cancerous cells may also be synergistic, which exploit
Till date Co3O4 nanoparticles have been studied for biomedical applications while nanocomposites of Co3O4/GO have studied only for their magnetic and catalytic properties [Citation36–37]. Therefore, in the present work cytotoxic properties of Co3O4/GO nanocomposite are explored. Here, GO is synthesised by using modified Hummer’s method and the GO nanosheets are decorated with Co3O4 nanoparticles by co-precipitation method. Well characterised Co3O4/GO nanocomposites were used for study of cytotoxicity on Mice melanoma cells (B16F10) via MTT assay.
2. Experimental section
2.1. Material
For synthesis of GO and Co3O4/GO nanocomposites different chemicals and reagents were used. Graphite flakes (99.8%, 325 mesh) were obtained from Alfa Aesar and hydrazine hydrate (NH2NH2) from Sigma-Aldrich. Sulphuric acid (H2SO4), potassium permanganate (KMnO4), sodium nitrate (NaNO3), hydrogen peroxide (H2O2), ethanol (CH3CH2OH), hydrochloric acid (HCl), cobalt acetate tetrahydrate (Co(C2H3O2)2·4H2O) and double distilled water (H2O) were obtained from Merck. All chemicals were used without further purification.
RPMI-1640, FBS, Antibiotics were purchased from Himedia Laboratories Pvt. Ltd. Mumbai- 400086, India. Mice melanoma cells (B16F10) was obtained from National Centre for Cell Science, Department of Biotechnology, Pune, India. Cells were grown as monolayer in RPMI-1640 medium, supplemented with 10% (v/v) FBS, antibiotics (penicillin 100 U/mL, streptomycin 10 μg/mL) and 1 mmol/L sodium pyruvate under standard conditions (37 °C) in a controlled humidified atmosphere containing 5% CO2.
2. Experimental section
2.1. Synthesis of graphene oxide (GO) sheets
Graphene oxide (GO) sheets were synthesised by modified Hummers method from expanded acid washed graphite flakes. In typical synthesis procedure, 2 g of sodium nitrate was added to 50 mL of sulphuric acid followed and the mixture was stirred for 30 min at 80 °C. In this mixture, 2 g of graphite powder was added under continuous stirring for 50 min at 70 °C. Further, the mixture was kept below 5 °C in the ice bath, and 12 g of potassium permanganate was slowly added into the mixture. The temperature of mixture was allowed to rise to 40 °C and the mixture was continuous stirred for another 6 h until it became a viscous reddish-brown paste. After stirring, 100 mL of double distilled water was slowly added to the mixture and the mixture was kept at 95 °C for another 1 h. Finally, 180 mL of hot double distilled water and 10 mL of 5% hydrogen peroxide solution were added to the mixture to stop the reaction. The residual metal ions were removed after the process of mixing by centrifugation and the precipitate was washed several times with 10% hydrochloric acid solution. The precipitate was dried at 50 °C under a vacuum condition and GO sheets were obtained.
2.2. Synthesis of Co3O4/GO nanocomposites
Nanocomposites of Co3O4 and reduced graphene oxide were successfully synthesised by co-precipitation method. Firstly, GO (100 mg) was dissolved into 80 mL in alcohol through sonication for 5 h. In the meantime, a fixed amount of Co (NO3)2⋅6H2O (300 mg) was dispersed into another 50 mL in alcohol. The mixture was magnetically stirred for 5 h. Afterthought the resulting solution was heated to 150 °C under reflux and vigorous magnetic stirring for 15 h. After that the system was cooled to room temperature. Finally, the obtained solution was centrifuged, washed with absolute ethanol and water many times and dried in a vacuum oven at 50 °C for 24 h. The obtained solid product was labelled Co3O4/GO nanocomposites. The schematic diagram of synthesis process of Co3O4/GO nanocomposites is given in .
2.3. Characterisation of nanomaterial
The GO and Co3O4/GO nanocomposites were characterised by different experimental techniques. For crystalline nature study, the X-ray diffraction pattern of nanocomposites was analysed using X-ray diffractometer from Panalytical. The surface morphology of these samples was analysed by scanning electron microscopy (Care-Zeiss EVO-40, working voltage 20 kV, Germany) and EDAX setup is inbuilt in SEM. The structure of GO and particles size distribution of Co3O4 NPs on GO sheets were studied using transmission electron microscopy (Zeiss EVO ED15), where accelerating potential was set as 200 keV. Raman spectra of GO and Co3O4/GO nanocomposites were recorded by using Raman spectrometer (HORIBA Xplora), where excitation source was 514 nm Ar-ion laser.
2.4. Cell viability by MTT assay
B16F10 5000 cells/well were seeded in a 96-well plate and after attachment the fresh medium having different concentrations of GO (100–500 μg/mL) added to the wells. The cells were allowed to grow for further 24 h. After completion of incubation, 20 μL of MTT (5 mg/mL in PBS) was added into each well and incubated further for 3 h and media was removed and 200 μL of DMSO was added to each well. The cells were again incubated for another 5–10 min at room temperature. The purple colour thus developed was measured at 595.
3. Result and discussion
3.1. XRD Studies
The phase formation of the Co3O4/GO nanocomposites, were confirmed by XRD spectrum (). The data were collected between scattering angle (2θ) from 10° to 70° at scanning rate of 5° min−1. XRD pattern of Co3O4/GO nanocomposites shows the characteristics peak at 37.3°, which indicates the polycrystalline nature and particle size of Co3O4 nanoparticles whereas, other XRD peaks appeared at 18.8° (111), 30.4° (220), 37.3° (311), 43.1° (400), 54.3° (422), 57.1° (511) and 62.6° (440)38. These XRD peaks indicate the ultra-fine nature and small polycrystalline size of Co3O4.
The average particle size of magnetic Co3O4 nanoparticles is calculated by using the Debye–Scherrer's formula [Citation39].
where d is grain size, λ is X-rays wavelength, β is full width at half maximum (FWHM) of diffraction peak (in radian), and θ is Bragg’s diffraction angle (in degree). The average particle size of Co3O4 nanoparticles decorated onto GO was found to be 35 nm which was evaluated from the line broadening of the (311) peak using Debye–Scherrer's formula. Peaks of Co3O4/GO nanocomposites shows that presence of all pure form with observable shifting [Citation38]. XRD analysis of GO ( Inset) also reveals that graphite powder has completely oxidised into graphene oxide as confirmed by peak observed at 11.3 (001) [Citation25].
3.2. SEM and EDX analysis
The surface morphology studies of GO nano sheets and Co3O4/GO nanocomposites were carried out using FESEM. The crumpled sheets like structure in confirms GO while irregular spherical structures deposited onto GO sheets in attributed to formation of Co3O4 nanoparticles on GO nanosheets [Citation40]. Elemental analysis confirms the presence of the targeted material of nanocomposite, here, EDX spectrum of GO and Co3O4/GO nanocomposite is shown in , respectively. EDX spectrum of Co3O4/GO nanocomposites confirms the presence of cobalt. EDX shows the presence of all elements CO, O and C particles with red, green, and pink colours, respectively and no foreign atom is obtained. Si peak arises due to silicon substrate.
3.3. Raman analysis
The degree of graphitisation was evaluated using Raman spectra, where main features of GO nano-sheets are the position and intensity of D and G peaks. In case of carbon-based materials, the higher ratio of intensity (ID/IG) corresponds to a lower degree of graphitisation [Citation41]. depicts the Raman spectra of GO nanosheets and Co3O4/GO nanocomposites. The position of D, G, 2D and D + G bands were obtained at 1341, 1594, 2670 and 2961 cm−1, respectively. Raman spectra of Co3O4/GO nanocomposites show slight shift in Raman peak position of D and G band relative to GO nanosheets. The D peak and G bands were slightly shifted from 1341 to 1339 cm−1 and 1594 to 1597 cm−1, while 2D and D + G bands were shifted from 2670 to 2675 cm−1 and 2961 to 2963 cm−1 respectively, signifying the possible charge transfer between the GO nanosheets and Co3O4 nanoparticles. It is well reported that highly disordered graphene shows a broaden G band and a relatively intense D band. The intensity ratio of the D and G peak (ID/IG) was used to evaluate the disordered crystal structure of carbon-based materials. The values of ID/IG for GO nanosheets and Co3O4/GO nanocomposites clearly shows that ID/IG ratio of the D and G bands was significantly increased from 1.08 (GO) to 1.11 (Co3O4/GO) after the synthesis process [Citation42, Citation43].
3.4. Morphological study of cell line and MTT assay
Then, cell viability test was performed using MTT assay for which mice melanoma cells (B16F10) were seeded in a 96-well plate. Firstly, changes in morphology of cell line was observed via seeding equal number of cells in the 6 well plates (i.e. 5000 cells per well). After proper attachment of the cells the RPMI-1640 medium is discarded and the fresh RPMI-1640 medium with different concentrations of GO and Co3O4/GO (10–200 μg/mL) added to the wells. The cells were allowed to grow for further 24 h then post treatment, cells were fixed in fixative solution and stained with crystal violet solution [Citation30, Citation44]. The excess stain was washed and images of cells were captured under inverted microscope (Nikon ECLIPSE Ti-S, Tokyo, Japan), at 10× magnification. The images of treated cells are shown in .
Similarly, next cell samples were seeded, washed and incubated for 24 h with different concentrations of GO and Co3O4/GO (10–200 μg/mL). After completion of incubation, 20 μL of MTT (5 mg/mL in PBS) was added into each well and incubated further for 3 h, then media was removed and 200 μL of DMSO was added to each well. The cells were again incubated for another 5–10 min at room temperature and after the development of the purple colour the absorbance was measured at 595 nm using a microplate reader. The cytotoxicity of the pure GO was dose dependent i.e. the viability of B16F10 cells is decreased with increased dose of GO. shows 60% cell viability was observed at 200 µg/mL of GO whereas shows that at 200 µg/mL of Co3O4/GO nanocomposite the viability of cells was only 37% indicating improved anti-cancerous activity [Citation31, Citation45]. This cell viability study suggests that GO possess anti-cancerous properties but integration of Co3O4 nanoparticles onto GO generates a nanocomposite possessing remarkable anti-cancer activity. In case of Co3O4/GO nanocomposite GO and Co3O4 synergistically inhibit cell aggregation and the death of cancer cells occur because of free radical production by Co3O4 [Citation30, Citation46]. In addition to altering cell morphology, Co3O4/GO nanocomposite decreases metabolic activity in cells, increases oxidative stress, damaging mitochondria, and ultimately damaging DNA by producing reactive oxygen species [Citation45, Citation47–50]. , shows comparative analysis of synthesised material with other reported materials for their anti-cancerous activities [Citation30, Citation31, Citation34, Citation44, Citation46, Citation47].
4. Conclusion
Co3O4/GO nanocomposites were synthesised using simple co-precipitation method, characterised and used for anti-cancer activity studies in mice melanoma cell line. Cobalt oxide nanoparticles have been reported for good anti-cancerous activity but it has high cell toxicity also, which limits its direct application in health care. While GO is reported for its mild anti-cancer activity but good biocompatibility. Addition of GO offer biocompatibility and lesser the toxicity of cobalt oxide nanoparticles while maintaining its anti-cancerous activity. Here, synergistic effect of GO and Co3O4 generate anti-cancerous activities by producing ROS which results in increased oxidative stress, damaging mitochondria, damaging DNA and finally death of cancer cell. This is the first study of using Co3O4/GO nanocomposite as anti-cancerous agents where results suggested that the nanocomposite shows significant cytotoxic effect and can be used as an alternative source for tumour or cancer treatment. However, the detailed molecular mechanism underlying cytotoxic effect of Co3O4/GO nanocomposites needs to be studied. This study can play an important role for its applications as cancer treatment and medicals etc.
Acknowledgements
AM is thankful to UGC for providing fellowship. The authors are thankful to AIRF, JNU, New Delhi for providing XRD, FESEM characterisation.
Disclosure statement
The authors report there are no competing interests to declare.
Data availability
The data that supports the findings of this study are available within the article.
Additional information
Funding
Notes on contributors
Anju Mishra
• • •
References
- K C, Thomas B. Cancer trends and burden in India. Lancet Oncol. 2018;19(12):e663.
- Sharma R. An examination of colorectal cancer burden by socioeconomic status: evidence from GLOBOCAN 2018. EPMA J. 2020;11(1):95–117.
- Sun P, Jin M, Ding L, et al. The Israeli journal of aquaculture-bamidgeh. 2017;69:1421.
- Al-Smadi M, Arqub OA. Computational algorithm for solving fredholm time-fractional partial integrodifferential equations of Dirichlet functions type with error estimates. Appl Maths Comput. 2019;342:280–294.
- Zhai X, Zhou Z, Tin C. Semi-supervised learning for ECG classification without patient-specific labelled data. Expert Syst Appl. 2020;158:113411.
- Mishra A, Mohanty T. Structural and morphological study of magnetic Fe3O4/ reduced graphene oxide nanocomposites. Mater Today Proc. 2016;3(6):1576–1581.
- Shaheen S, Iqbal A, Ikram M, et al. Graphene oxide-ZnO nanorods for efficient dye degradation, antibacterial and in-silico analysis. Appl Nanosci. 2022;12(2):165–177.
- Alves NM, Mano JF. Chitosan derivatives obtained by chemical modifications for biomedical and environmental applications. Int J Biol Macromol. 2008;43(5):401–414.
- Ali M, Ikram M, Ijaz M, et al. Green synthesis and evaluation of n-type ZnO nanoparticles doped with plant extract for use as alternative antibacterials. Appl Nanosci. 2020;10(10):3787–3803.
- Rashid M, Ikram M, Haider A, et al. Photocatalytic, dye degradation, and bactericidal behaviour of Cu-doped ZnO nanorods and their molecular docking analysis. Dalton Trans. 2020;49(24):8314–8330. Jun
- Saleem A, Imran M, Shahzadi A, et al. Materials research express. Mater Res Express. 2018;2019;6(1):015003.
- Wahab A, Imran M, Ikram M, et al. Dye degradation property of cobalt and manganese doped iron oxide nanoparticles. Appl Nanosci. 2019;9(8):1823–1832.
- Choi YJ, Gurunathan S, Kim JH. Graphene oxide–silver nanocomposite enhances cytotoxic and apoptotic potential of salinomycin in human ovarian cancer stem cells (OvCSCs): a novel approach for cancer therapy. IJMS. 2018;19(3):710. 1
- Almeer RS, Alarifi S, Alkahtani S, et al. The potential hepatoprotective effect of royal jelly against cadmium chloride-induced hepatotoxicity in mice is mediated by suppression of oxidative stress and upregulation of Nrf2 expression. Biomed Pharmacother. 2018;106:1490–1498.
- Liao C, Li Y, Tjong SC. Bactericidal and cytotoxic properties of silver nanoparticles. IJMS. 2019;20(2):449.
- Parnianchi F, Nazari M, Maleki J, et al. Combination of graphene and graphene oxide with metal and metal oxide nanoparticles in fabrication of electrochemical enzymatic biosensors. Int Nano Lett. 2018;8(4):229–239.
- Han W, Ren L, Qi X, et al. Synthesis of CdS/ZnO/graphene composite with high-efficiency photoelectrochemical activities under solar radiation. Appl Surface Sci. 2014;299:12–18.
- Mishra A, Singh VK, Mohanty T. Coexistence of interfacial stress and charge transfer in graphene oxide-based magnetic nanocomposites. J Mater Sci. 2017;52(13):7677–7687.
- Hong L, Yao H, Wu Z, et al. Eco‐compatible solvent‐processed organic photovoltaic cells with over 16% efficiency. Adv Mater. 2019;31(39):1903441. (ev. B 91, 094429 (2015)).
- Mishra A. Study of organic pollutant removal capacity for magnetite@ graphene oxide nanocomposites. Vacuum. 2018;157:524–529.
- Compton OC, Nguyen ST. Graphene oxide, highly reduced graphene oxide, and graphene: versatile building blocks for carbon-based materials. Small. 2010;6(6):711–723.,
- Paredes JI, Villar-Rodil S, Martínez-Alonso A, et al. Graphene oxide dispersions in organic solvents. Langmuir. 2008;24(19):10560–10564.
- Mishra A, Kuanr BK, Mohanty T. AIP Conference Proceedings 1832, 050006 2017.
- Qumar U, Hassan J, Naz S, et al. Silver decorated 2D nanosheets of GO and MoS2 serve as nanocatalyst for water treatment and antimicrobial applications as ascertained with molecular docking evaluation. Nanotechnology. 2021;32(25):255704. 25 10.1088/1361-6528/abe43c. 1 Apr
- Ikram M, Raza A, Imran M, et al. Hydrothermal synthesis of silver decorated reduced graphene oxide (rGO) nanoflakes with effective photocatalytic activity for wastewater treatment. Nanoscale Res Lett. 2020;15(1):95.
- Wang X, Yu S, Jin J, et al. Application of graphene oxides and graphene oxide-based nanomaterials in radionuclide removal from aqueous solutions. Sci Bull. 2016;61(20):1583–1593.
- Loh KP, Bao Q, Eda G, et al. Graphene oxide as a chemically tuneable platform for optical applications. Nat Chem. 2010;2(12):1015–1024.
- Mishra A, Mohanty T. One step synthesis of Fe3O4/GO nanocomposites at 100 °C and its magnetic properties. Integr Ferroelectr. 2017;184(1):178–185.
- Kavinkumar T, Krishnamoorthy V, Ravikumar V, et al. J Colloid Interface Sci. 2017;505:1125–1133.
- Shaheen F, Aziz MH, Fatima M, et al. In vitro cytotoxicity and morphological assessments of GO-ZnO against the MCF-7 cells: determination of singlet oxygen by chemical trapping. Nanomaterials. 2018;8(7):539.
- Sharif S, Murtaza G, Meydan T, et al. Structural, surface morphology, dielectric and magnetic properties of holmium doped BiFeO3 thin films prepared by pulsed laser deposition. Thin Solid Films. 2018;662:83–89.
- Ali D, Alarifi S, Alkahtani S, et al. Silver-doped graphene oxide nanocomposite triggers cytotoxicity and apoptosis in human hepatic normal and carcinoma cells. Int J Nanomed. 2018;13:5685–5699.
- Khan GMA, Shaikh H, Alam MS, et al. Effect of chemical treatments on the physical properties of non-woven jute/PLA biocomposites. BioResources. 2015;10(4):7386.
- Jarestan M, Khalatbari K, Pouraei A, et al. Preparation, characterisation, and anticancer efficacy of novel cobalt oxide nanoparticles conjugated with thiosemicarbazide. 3 Biotech. 2020;10(5):1.
- Rauwel E, Al-Arag S, Salehi H, et al. Assessing cobalt metal nanoparticles uptake by cancer cells using live Raman spectroscopy. Int J Nanomed. 2020;15:7051–7062.
- Elkhadragy MF, Al-Olayan EM, Al-Amiery AA, et al. Protective effects of fragaria ananassa extract against cadmium chloride-induced acute renal toxicity in rats. Biol Trace Elem Res. 2018;181(2):378–387.
- Zacharopoulou N, Tsapara A, Kallergi G, et al. 2020;21:533–540.
- Zhao Y, Chen S, Sun B, et al. Graphene-Co3O4 nanocomposite as electrocatalyst with high performance for oxygen evolution reaction. Sci Rep. 2015;5:7629.
- Qian C, Guo X, Zhang W, et al. Co3O4 nanoparticles on porous bio-carbon substrate as catalyst for oxygen reduction reaction. Microporous Mesoporous Mater. 2019;277:45–51.
- Pourzare K, Farhadi S, Mansourpanah Y. Graphene oxide/Co3O4 nanocomposite: synthesis, characterization, and its adsorption capacity for the removal of organic dye pollutants from water. Acta Chim Slov. 2017;64(4):945–958.
- Mishra A, Sharma V, Mohanty T, et al. Microstructural and magnetic properties of rGO/MnFe2O4 nanocomposites; relaxation dynamics. J Alloys Compd. 2019;790:983–991.
- Wang T, Wang J, Yang Y, et al. Co3O4/reduced graphene oxide nanocomposites as effective phosphotriesterase mimetics for degradation and detection of paraoxon. Ind Eng Chem Res. 2017;56(34):9762–9769.
- Mishra A, Mishra A. Two fold characteristics of swift heavy ion irradiation on Co3O4/RGO nanocomposites. Appl Surf Sci. 2019;486:474–481.
- Halkai KR, Mudda JA, Shivanna V, et al. Cytotoxicity evaluation of fungal-derived silver nanoparticles on human gingival fibroblast cell line: an in vitro study. J Conserv Dent. 2019;22(2):160.
- Ahmadi S, Fazilati M, Mousavi SM, et al. Anti-bacterial/fungal and anti-cancer performance of green synthesised Ag nanoparticles using summer savoury extract. J Exp Nanosci. 2020;15(1):363–380.
- Xu X, Shen J, Qin J, et al. Cytotoxicity of bacteriostatic reduced graphene Oxide-Based copper oxide nanocomposites. JOM. 2019;71(1):294–301.
- Alarifi S, Ali D, Alkahtani S, et al. Iron oxide nanoparticles induce oxidative stress, DNA damage, and caspase activation in the human breast cancer cell line. Biol Trace Elem Res. 2014;159(1–3):416–424.
- Mahendran D, Kavi Kishor PB, Geetha N, et al. Efficient antibacterial/biofilm, anti-cancer and photocatalytic potential of titanium dioxide nanocatalysts green synthesised using gloriosa superba rhizome extract. J Exp Nanosci. 2021;16(1):11–30.
- Yuan C, Jiang B, Xu X, et al. Anti-human ovarian cancer and cytotoxicity effects of nickel nanoparticles green-synthesised by Alhagi maurorum leaf aqueous extract. J Exp Nanosci. 2022;17(1):113–125.
- Zhang H, Li T, Luo W, et al. Green synthesis of Ag nanoparticles from Leucus aspera and its application in anticancer activity against alveolar cancer. J Exp Nanosci. 2022;17(1):47–60.