Abstract
Colloidal CdSe/CdS/ZnS core/multishell nanostructure with the different thicknesses of CdS and ZnS shell was prepared by chemical method using CdO and ZnO. The absorption, photoluminescence and Raman scattering spectra of CdSe core, CdSe/CdS core/shell and CdSe/CdS/ZnS core/multishell structure have been comparatively studied. The photoluminescence full width at half maximum of CdSe core is less than 20 nm, indicating the monodisperse colloidal CdSe nanocrystals. It was found that the reaction temperature needed for the formation of ZnS shell is higher than that for the growth of CdS shell. The strong increase in the emission intensity by successively coating the CdS and ZnS shell around the CdSe core has been discussed. The effect of shell thickness on the spectroscopic characteristics of CdSe/CdS/ZnS core/multishell nanostructure has been investigated.
1. Introduction
Colloidal semiconductor nanocrystals have attracted much attention due to their size tunable optoelectronic properties. The application of the high quality nanocrystals to bio-imaging, photovoltaic devices and multicolour light emitting diodes is important technological issues at present Citation1–3. Because of their size-dependent photoluminescence (PL) tunable across the visible spectrum, CdSe nanocrystals have become the most extensively investigated object. Besides the development of synthesis techniques to prepare samples with narrow size distribution, much experimental work is devoted to molecular surface modification to improve the luminescence efficiency and colloidal stability of the particles or to develop a reliable processing chemistry. To suppress surface effects, inorganic passivation with wide band gap material is a well developed solution to enhance the quantum yield and stability of nanocrystals Citation4,Citation5. The CdSe/CdS core/shell nanocrystal has less lattice mismatch (3.9%) between the core and shell than the CdSe/ZnS nanocrystal (12%), so it can provide better stability by decreasing interfacial strain. However, the energy levels of the valence band maximum and conduction band minimum of CdS are not sufficient to confine both electrons and holes in the CdSe core so that the CdSe/CdS nanostructure exhibited less quantum yield compared to CdSe/ZnS Citation6. In order to solve above limitation the core/multishell structures such as CdSe/CdS/ZnS, CdSe/ZnSe/ZnS Citation7, CdSe/CdS/Zn0.5Cd0.5S/ZnS Citation1 have been prepared by inserting a interlayer between the CdSe core and ZnS outermost shell.
In this work, the colloidal CdSe/CdS/ZnS core/multishell nanostructure was synthesized in octadecene using CdO, ZnO. This core/multishell nanostructure is based on engineering both energy band gap and lattice spacing to effectively confine the exciton in the emitting core and reduce of the strain inside heterostructure. The preparation condition and the effect of shell thickness on the PL intensity were investigated for obtaining the core/multishell nanostructure with narrow size distribution, high quantum yield and stable optical characteristics.
2. Experimental
Chemicals: Cadmi oxide (CdO), zinc oxide (ZnO), oleic acid (OA), sulfur (S), selenium (Se), octadecene (ODE), tri-n-octylphosphine (TOP).
The CdSe/CdS/ZnS core/multishell nanostructure was prepared by three main steps:
Step A
Preparation of CdSe core. A mixture of CdO, OA and ODE was stirred and heated to 190–200°C. At this temperature, a solution of TOPSe in ODE was rapidly injected into this hot solution to grow CdSe core.
Step B
Preparation of CdS interlayer. A quantity of ODE was loaded into a reaction flash and heated to various temperatures. Subsequently, the CdSe nanocrystals in toluene were added, then the Cd2+ injection solution (CdO dissolved with OA in ODE) and the S2− injection solution (S powder dissolved in ODE) were slowly injected into CdSe solution.
Step C
Preparation of ZnS exterior shell was performed by a slow injection of Zn2+ and S2− precursor solution into the solution of CdSe/CdS in ODE at various temperatures.
The preparation of CdSe core, CdS and ZnS shell layer was performed under nitrogen gas flow. The reaction mixture obtained after each step was allowed to cool to room temperature, and a centrifugation procedure was used to purify the nanocrystals from unreacted precursors and side products. After centrifugation, the nanocrystals were dispersed in toluene for further processing. The CdSe core size was estimated from the first exciton peak position of the absorption spectrum. The particle concentration of the purified CdSe solution in toluene, as stock solution for core/shell growth, was determined using Beer's law with the reported extinction coefficient of CdSe nanocrystals Citation8. The quantity of precursors added into the growth solution for each CdS and ZnS shell monolayer (ML) was based on the size of the nanocrystal templates, the concentration of the nanocrystals in the solution, and the lattice constants of the crystal system Citation9.
The optical absorption spectra were recorded using Jasco V670 UV-Vis-NIR spectrometer. The PL and Raman scattering (RS) spectra were measured by LABRAM-1B spectrometer using 488 nm excitation line of Ar+ ion laser. All the optical measurements were performed at room temperature.
3. Results and discussion
The evolution of the absorption and PL spectra of colloidal CdSe nanocrystals during the growth at 190–200°C (, respectively) shows the rapid increase in their size during first reaction minutes, and the CdSe nanocrystals grow more slowly in the next time. Simultaneously, the full width at half maximum (FWHM) of the PL peak gradually narrows and achieves the value of 19 nm after 20 min of the reaction. Note that the PL-FWHM observed by single dot spectroscopy is less than 20 nm Citation10, so the obtained CdSe nanocrystals are monodisperse. In the next reaction time the PL-FWHM gradually broadens to 25 nm after 180 min of the reaction because of the monomer concentration in the solution is depleted during the growth Citation11. By varying the Cd to Se molar ratio in the stock solution we have obtained the colloidal CdSe nanocrystals with the PL-FWHM less than 20 nm for 2 h before the size distribution is defocused.
Figure 1. Evolution of the absorption (a) and PL (b) spectra in the growth process of CdSe nanocrystals.
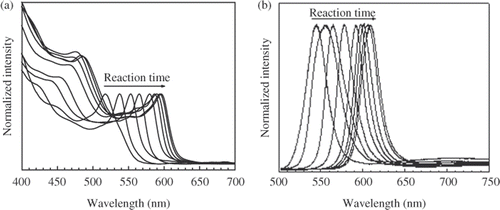
The emission property of CdSe/CdS and CdSe/ZnS core/shell nanostructure with the shells prepared during the same reaction time at the various temperatures was investigated for determining the suitable reaction temperature. The PL spectra of CdSe core and obtained core/shell nanocrystals are compared in . All spectra are normalized in the intensity. The CdS shell cannot provide potential barrier large enough to prevent the leakage of the exciton. The increase of reaction temperature leads to strong redshift of the short wavelength emission peak of CdSe/CdS nanostructure, reflecting an increased leakage of the exciton into the thicker CdS shell Citation7. In the case of CdSe/ZnS nanostructure, due to the large band gap of ZnS the redshift in the PL spectra proves the formation of an alloy layer between CdSe core and ZnS shell. Similarly, it is possible that a CdSe1− x S x layer also was created between CdSe core and CdS shell of CdSe/CdS nanostructure.
Figure 2. Photoluminescence spectra of CdSe/CdS and CdSe/ZnS nanostructure with the shell layers prepared at various temperatures. PL spectrum of CdSe core is inserted for comparison.
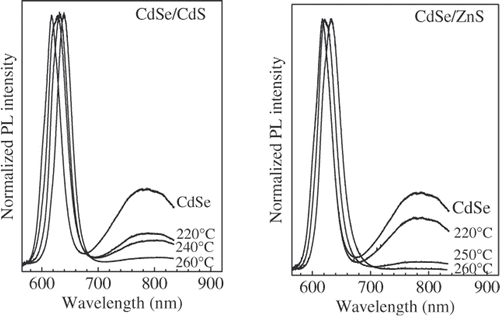
As the reaction temperature increases, the intensity of the surface emission band decreases due to the increased passivation of CdSe core surface by the exterior shell layer. Comparing the surface emission intensities of the obtained CdSe/CdS and CdSe/ZnS nanostructure it is found that a higher reaction temperature was necessary for the formation of the ZnS shell layer. The lattice mismatch between the core and the shell creates a barrier for nucleation of the shell material Citation7. Therefore, the reaction temperatures for growing the CdS and ZnS shell layer were chosen at 250 and 260°C, respectively.
The PL spectra of the CdSe/CdS and CdSe/CdS/ZnS nanostructure with the different thickness of CdS and ZnS shell are compared in , respectively. All the PL spectra are normalized in the intensity according to the absorbance at 488 nm excitation wavelength. The CdS shell layer around the CdSe core results in an improvement of PL intensity. However, the PL intensity of CdSe/CdS nanostructure decreases when the CdS shell thickness exceeds two MLs, presumably as a result of the higher concentration of structural defects created within the thicker CdS shell Citation12.
Figure 3. Absorption and PL spectra of CdSe/CdS (a) and CdSe/CdS/ZnS (b) nanostructure with different shell thicknesses. (The thickness of CdS interior layer in CdSe/CdS/ZnS structure is three MLs).
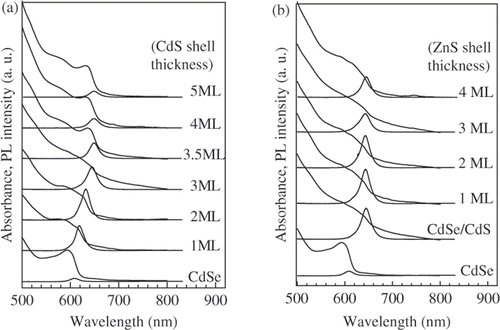
The ZnS shell created around CdSe/CdS nanocrystals results in a small blueshift of the emission peak. The reason of this blueshift is the partial formation of a Zn1− x Cd x S alloy layer between CdS and ZnS shell when the CdSe/CdS particles are covered with addition ZnS. At high temperature the Zn-atoms might diffuse into the Cd-rich regions of the CdS shell, thus increasing the band-offset of the shell and hence the effective confinement Citation1. The highest emission intensity of CdSe/CdS/ZnS nanostructure with three MLs CdS shell was obtained for the ZnS shell thickness of one ML. The further increase of the ZnS shell thickness is accompanied with the decrease in the PL intensity. The dependence of PL intensity on the thickness of CdS shell () shows that the most suitable thickness of CdS shell is about two MLs.
The emission peaks of CdSe core, CdSe/CdS and CdSe/CdS/ZnS nanostructure are compared in . The strong increase in PL intensity of core/multishell nanostructre is due to two causes: (1) The CdS/ZnS multishell could passivate surface defects of the CdSe core to enhance the emission intensity and (2) By investigating the optical stability of CdSe core it was found that a thin disorder CdSe layer was formed around the high quality CdSe core from unreacted precursors when the reaction mixture was cooled to room temperature. The annealing of CdSe core in the growth process of CdS and ZnS shell results in an improvement of its crystal quality and leads to the increase in PL intensity.
Figure 4. The emission peaks of CdSe core (a), CdSe/CdS (b) and CdSe/CdS/ZnS (c) nanostructure. The thicknesses of CdS and ZnS layer are three and one MLs, respectively.
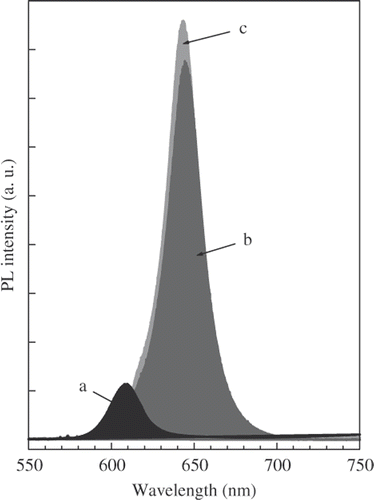
The RS spectra of CdSe core and CdSe/CdS core/shell nanostructure with CdS shell thickness of three MLs are presented in . The strong scattering due to the longitudinal optical (LO) phonon mode of the CdSe core was observed (). The LOCdSe peak shifts to lower frequency side in comparison with that of bulk CdSe crystal, and has the asymmetric shape due to the quantum confinement of phonons and the contribution of the surface optical (SO) phonon mode Citation13.
Figure 5. Raman scattering spectra of CdSe core (a) and CdSe/CdS structure (b). The LO and SO phonon mode are shown by dashed and dotted lines, respectively.
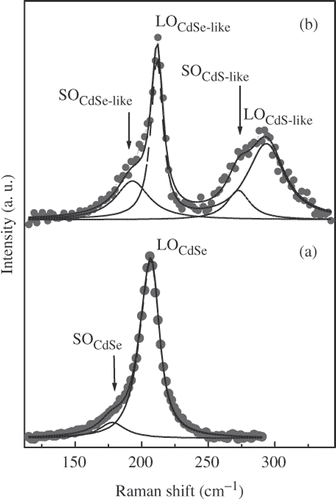
In order to determine the size of CdSe core, the experimental RS spectrum was fitted applying the phonon confinement model of Campbell and Fauchet Citation14 for LOCdSe mode and using Lorentz function for SOCdSe mode. The best fitting result is shown by the solid line in . The LOCdSe and SOCdSe phonon mode are shown by dashed and dotted line, respectively. The obtained diameter of the CdSe core is 5.8 nm, in good agree with the value of 5.9 nm determined from the absorption spectrum.
The RS spectrum of the CdSe/CdS structure is characterized by two strong peaks (). The LOCdSe peak shifts to higher frequency side (211 cm−1), in comparison with that of CdSe core (207 cm−1), and narrows. Moreover, the shoulder of LOCdSe peak increases in the intensity. The appearance of other peak at 294 cm−1 indicates the presence of a thin CdS shell around the CdSe core. The RS spectrum of CdSe/CdS structure can be well described by the phonon confinement model with LOCdSe-like, LOCdS-like confined optical phonon mode, and SOCdSe-like, SOCdS-like surface phonon mode as reported in Citation15. The best fitting result is shown by the solid line, the LO and SO phonon components are shown by dashed and dotted lines, respectively, in . It is possible that a thin CdSe1− x S x layer was formed between the CdSe core and CdS shell as mentioned above. However, the characteristic peaks of this alloy layer were not exhibited clearly in the obtained RS spectrum.
4. Conclusion
The CdSe/CdS/ZnS core/multishell nanostructure with the CdS interlayer between CdSe core and ZnS exterior shell was prepared by three separate steps. The PL-FWHM of CdSe core is less than 20 nm, indicating the monodisperse colloidal CdSe nanocrystals. The emission intensity of core/multishell nanostructure is enhanced not only by passivating the surface defects but also due to the improvement of the crystal quality of CdSe core in the growth process of shell layers, and strongly depends on the shell thickness. The highest PL efficiency of CdSe/CdS/ZnS core/multishell nanostructure can be obtained for the CdS and ZnS shell thickness of two MLs and about 1–2 MLs, respectively. The formation of the alloy layer at the CdSe/CdS and CdS/ZnS interface causes the change in the emission and vibrational characteristics of the core/multishell nanostructure.
Acknowledgement
This work was supported by the National Fundamental Research Foundation of Vietnam.
References
- Xie , R , Kolb , U , Li , J , Basché , T and Mews , A . 2005 . Synthesis and characterization of highly luminescent CdSe-Core CdS/Zn0.5Cd0.5S/ZnS multishell nanocrystals . J. Am. Chem. Soc. , 127 : 7480 – 7488 .
- Mekis , I , Talapin , DV , Kornowski , A , Haase , M and Welker , H . 2003 . One-pot synthesis of highly luminescent CdSe/CdS core/shell nanocrystals via organometallic and “Greene” chemical approaches . J. Phys. Chem. B , 107 : 7454 – 7462 .
- Steckel , JS , Zimmer , JP , Coe-Sullivan , S , Stott , NE , Bulović , V and Bawendi , MG . 2004 . Blue luminescence from (CdS)ZnS core-shell nanocrystals . Angew. Chem. Int. Ed. , 43 : 2154 – 2158 .
- Xu , L , Wang , L , Huang , X , Zhu , J , Chen , H and Chen , K . 2000 . Surface passivation and enhanced quantum-size effect and photo stability of coated CdSe/CdS nanocrystals . Physica E , 8 : 129 – 133 .
- Li , J , Wang , YA , Guo , W , Keay , JC , Mishima , TD , Johnson , MB and Peng , X . 2003 . Large-scale synthesis of nearly monodisperse CdSe/CdS core/shell nanocrystals using air-stable reagents via successive ion layer adsorption and reaction . J. Am. Chem. Soc. , 125 : 12567 – 12575 .
- Jun , S , Jang , E and Lim , JE . 2006 . Synthesis of multi-shell nanocrystals by a single step coating process . Nanotechnology , 17 : 3892 – 3896 .
- Talapin , DV , Mekis , I , Göetzinger , S , Kornowski , A , Benson , O and Weller , H . 2004 . CdSe/CdS/ZnS and CdSe/ZnSe/ZnS core-shell-shell nanocrystals . J. Phys. Chem. B , 108 : 18826 – 18831 .
- Yu , WW , Qu , L , Guo , W and Peng , X . 2003 . Experimental determination of the extinction coefficient of CdTe, CdSe, and CdS nanocrystals . Chem. Mater. , 15 : 2854 – 2860 .
- Battaglia , D , Li , JL , Wang , Y and Peng , X . 2003 . Colloidal two-dimensional systems: CdSe quantum shells and wells . Angew. Chem. Int. Ed. , 42 : 5035 – 5039 .
- Qu , L and Peng , X . 2002 . Control of photoluminescence properties of CdSe nanocrystals in growth . J. Am. Chem. Soc. , 124 : 2049 – 2055 .
- Peng , X , Wickham , J and Alivisatos , AP . 1998 . Kinetics of II–VI and III–V colloidal semiconductor nanocrystal growth: “Focusing” of size distributions . J. Am. Chem. Soc. , 120 : 5343 – 5344 .
- Reiss , P , Bleuse , J and Pron , A . 2002 . Highly luminescent CdSe/ZnSe core/shell nanocrystals of low size dispersion . Nano Lett. , 2 : 781 – 784 .
- Arora , AK , Rajalakshmi , M and Ravindran , TR . 2004 . Phonon confinement in nanostructured materials . Encyclopedia of Nanoscience and Nanotechnology , 8 : 499 – 512 .
- Campbell , H and Fauchet , PM . 1986 . The effects of microcrystal size and shape on the one phonon Raman spectra of crystalline semiconductors . Solid State Commun. , 58 : 739 – 741 .
- Singha , A and Roy , A . 2005 . Phonon confinement and surface phonon modes in CdSe-CdS core-shell nanocrystals . Rev. Adv. Mater. Sci. , 10 : 462 – 466 .