Abstract
We report here the humidity-sensing characteristics of conducting polypyrrole (PPY)-silver nanocomposites prepared by wet-chemical technique. Addition of silver into the conducting polymer network shows remarkable change in resistance with relative humidity (RH). The resistance of PPY-silver nanocomposites is found to increase with RH till a threshold value, above which the resistance tends to decrease. The threshold RH value is significantly reduced with the increase in silver concentration into the conducting polymer network. The modified metal–polymer interface and the associated interfacial water molecules play the critical role in determining the electrical transport and hence the humidity-response characteristics of the nanocomposites.
1. Introduction
Humidity sensors find widespread application in many practical purposes, e.g. air conditioning systems, electronic devices, aviation systems and food processing units. Among the varieties of materials, conducting polymers have been largely examined as sensor materials by various researchers Citation1–4. The basis of sensing is the change in electrical resistivity of conducting polymers when exposed to humid environments, UV irradiation, temperature variations and stressed conditions. In humid atmosphere, the reaction between the conducting polymer and water molecules is found to result in a change (increase or decrease) in resistivity. The response is not always very straightforward and strongly depends on the detailed reaction mechanisms between water molecules and polymer backbone. Our interest lies in looking into humidity-response characteristics for conducting polymer and metal composites. Embedding of metal nanoparticles in the polymer has become very popular way of obtaining nanocomposites with improved physical and chemical properties and finds wide range of applications in various devices Citation5, Citation6. In this article, we report the humidity-sensing properties of conducting polypyrrole (PPY)-silver composite nanowires and to find the role of water molecules in changing the resistivity of such low-dimensional organic–inorganic composite nanostructures. An array of such nanowires may readily be used to construct smart humidity sensors giving a direct low-power electrical signal read-out. Now, among the numerous conducting polymers synthesised to date, PPY is one of the most extensively studied polymers because of its high environmental stability, high electrical conductivity, high thermal stability and can also be easily oxidised both chemically and electrochemically Citation7, Citation8. On the other hand, silver has been chosen as the inorganic filler because of its high electrical conductivity and chemical stability. While designing such conducting polymer-based composite sensors, a good compromise has to be made between the optimal electrical conductivity and the sensitivity to external parameters, for example, here the humidity. Addition of metal fillers within the conducting polymer can endow the tunable and measurable electrical conductivity with high sensitivity to humid conditions. One key aspect of the incorporation of metal nanoparticles into conducting polymer matrix on the mechanical properties has to be kept in mind while designing the sensor geometry Citation9, Citation10. Now, despite the volume of literature available on the various aspects of conducting PPY, humidity-sensing characteristics of metal-conducting polymer nanocomposites have scarcely been reported.
2. Experimental
2.1. Synthesis of conducting polymer
In the typical synthesis procedure, at first pyrrole monomer is dissolved in a solvent mixture of ethanol (99.99%, Jiangsu Huaxi, China) and distilled water. The volume ratio of water and alcohol is maintained 2 : 1 throughout to prepare solvent. On the other hand, ammonium per sulfate (NH4)2H2S2O8 (APS) (SRL, India) has been used as the oxidising agent for the synthesis of conducting PPY. A solution of APS in ethanol–water is prepared separately. Both the solutions are continuously stirred for 1 h. The molar ratio of PPY and APS is maintained as PPY : APS = 1 : 2. The pyrrole solution is then slowly added to the APS containing solution (ice-cooled) and results an instantaneous formation of black precipitate which is kept in an ice bath for 12 h. The precipitate is then washed in methanol and distilled water for five times, filtered and finally dried at room temperature in vacuum. The pure conducting PPY sample thus prepared is referred as Sample 1. A schematic diagram for the synthesis of conducting PPY is shown in .
2.2. Synthesis of conducting polymer-silver nanocomposites
A solution of AgNO3 and pyrrole in ethanol–water is prepared with desired molar ratio and rapidly stirred for 2 h. The molar ratio of PPY and APS is maintained as PPY : APS = 1 : 2. The AgNO3–pyrrole solution is slowly added to the APS solution (ice-cooled) under stirring conditions instantly forming the precipitate containing PPY-silver composites. The mixture kept in ice bath for 12 h is then removed, washed in methanol and distilled water for five times, filtered and dried at room temperature in vacuum. The silver concentration in the composites is varied with choice of different concentration of AgNO3 solutions. We prepared three composite samples with different silver loadings in the PPY : 0.6 wt%, 1.2 wt% and 2.4 wt%, respectively. The samples are hereinafter referred as Sample 2, Sample 3 and Sample 4, respectively. The reaction mechanism responsible for the simultaneous oxidation of pyrrole monomer and reduction of Ag+ ions to metallic silver in the composites can be attributed to a redox interaction between the components. The presence of Ag+ ions possibly did not hinder the oxidation of pyrrole and hence the formation of PPY. The redox type of reaction can be written as:
2.3. Structural and electrical measurements
The morphological and structural studies have been done using scanning electron microscopy (SEM, Camscan-2, JEOL), transmission electron microscopy (TEM, CM-12, Philips) and X-ray diffraction (XRD) techniques. XRD studies are performed in a Philips PW-1710 diffractometer using Ni-filtered Co-K α radiation (λ = 0.178897 nm) as X-ray source at 40 kV, 20 mA. The θ/2θ scans are carried out at a scanning speed of 2°/min in the 2θ range ∼ 10–100°. The humidity-sensing characteristics is obtained by measuring the change in resistance of the samples with different values of relative humidity (RH) of the environment. Prior to measurements, the samples are properly dried in vacuum and then inserted into the test chamber equipped with temperature and RH controllers. The resistance and current–voltage (I–V) characteristics are measured by conventional two-probe technique using an electrometer (Keithley-7514). Electrical connections are made on the 1 mm thick palletised samples with fine copper wires using electro-conductive silver paste.
3. Results and discussion
The morphological structures obtained from SEM and TEM are given in , respectively, for a representative Sample 4. The SEM image shows the interpenetrating polymer-silver nanoparticle network and is observed for all the polymer-silver composite samples. The typical size (diameter) of the silver nanoparticles as measured from TEM for Sample 4 to be ∼15 nm. The diffuse rings in the selected area diffraction pattern (SAED) pattern shown in the inset of also reflect the growth of silver nanoparticles in the conducting polymer network. The XRD data given in for a representative sample 4 also clearly shows the cubic crystalline growth of silver nanoparticles in the PPY with the appearance of (100), (101), (103), (105), (110), (202) and (203) peaks. The data has been compared with JCPDS (card no. 411402) data file for bulk silver. The experimental data shows slight shift in the XRD peak positions with some preferential orientations compared to bulk. This can be ascribed due to the nanocrystalline growth of silver as well as the strain introduced during the growth of nanocomposites Citation11.
Figure 2. (a) SEM image for a representative Sample 4 indicating growth of conducting polymer-silver nanocomposites and (b) TEM image of a silver nanoparticle in the nanocomposites Sample 4 indicating typical size ∼15 nm. The inset of (b) shows the SAED of silver nanoparticles.
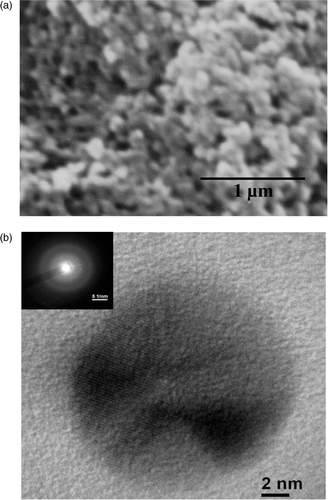
The current–voltage (I–V) characteristics of the samples are plotted in d). shows that for pure conducting polymer sample, I–V curve is non-ohmic. Addition of silver into the polymer results into the decrease in resistivity, and also the linearity in I–V curve is increased. This clearly confirms the increase in number of ohmic conductive paths with the increase in silver particle concentration into the polymer network. Now we look into humidity-response characteristics by measuring the electrical resistance of the sample with RH of the environment. The data are plotted in d) for conducting PPY and that of silver-conducting PPY nanocomposites. The data reveals that there are marked differences in the humidity-response behaviour of nanocomposites samples compared to pure conducting PPY sample. Apart from an increase in conductance, concentration of silver plays an important role in determining the overall humidity-response characteristics of the nanocomposites. For the pure conducting polymer sample, the resistance changes with the increase in RH almost in a linear way. This is explicable by considering the porous morphology of the conducting polymer, as revealed in SEM image. Initial exposure of humidity to the nanocomposites leads to possibly swelling of water and may block the electronic conducting paths Citation12, Citation13. As the nanocomposite swells, polymers chains as well as the silver nanoparticles are pulled apart giving rise to a poor electrical connectivity of the network. The degree of swelling of course depends on the bonding between polymer chains and silver nanoparticles, the duration of exposure of the nanocomposite to the sensing environment and its chemical nature. This phenomenon consequently gives rise to an increase in the resistance value as observed in . The resistance R is observed to follow the equation Citation13: R/R 0 = (s/s0)exp[γΔs)], where R 0 is the initial resistance before swelling, Δs is the change in separation (between conducting particles/polymer chains) under swelling and γ be a factor depending the potential barrier height between conducting particles/polymer chains. The overall response, however, depends on the detailed porous morphology and may be subjected to different synthesis conditions. Addition of metal fillers, of course, deviate the system from both morphological and electronic conduction points of view. Presence of metal interfaces within polymer and surrounding water molecules leads to complex pathways of electronic conduction. d) reveals that the resistance of the nanocomposites shows an initial increase with RH value, reaches a threshold value beyond which it decreases further. The initial increase of resistances with RH is not very straightforward, and changes become more rapid with the increase in silver nanoparticle concentrations. Also the threshold value of RH beyond which the resistance shows the opposite trend of decrease in resistances severely depends on the metal concentration in the conducting polymer. The two opposite behaviours on either side of the threshold point clearly suggest the critical role of water molecules in regulating the conduction pathways in the presence of metal interfaces within the polymer. To explain the data, we have to consider three possible electronic pathways: first, the blocking of pathways between conducting polymer chains; second, the increase or decrease in connectivity between the metal nanoparticles in presence of water molecules; and finally, any change in the metal–polymer interfaces. Besides, one has to consider both the presence of bulk water and the interfacial water molecules (if any) at the metal–polymer interface governing the electronic transports Citation14–17.
Figure 4. Room temperature current–voltage (I–V) characteristics for (a) Sample 1, (b) Sample 2, (c) Sample 3 and (d) Sample 4.
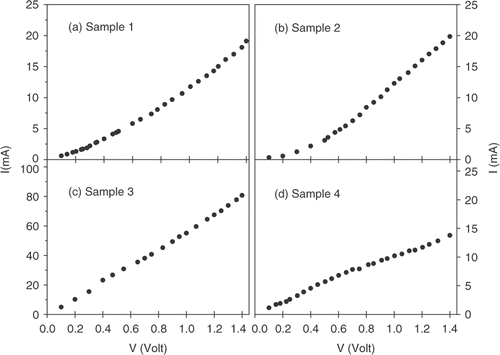
Figure 5. Variation of resistance with RH at room temperature for (a) Sample 1, (b) Sample 2, (c) Sample 3 and (d) Sample 4.
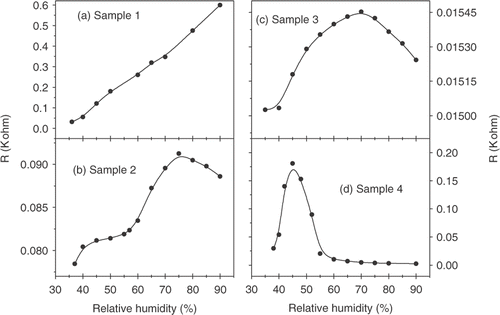
Many authors have studied the effect of water molecules in various composites on the mechanical and electrical properties Citation15. In most situations, water can diffuse to the polymer or metal–polymer interfaces by chemisorption or physisorption processes. The total water accumulation, of course, depends on the RH of the environment and chemical nature of the interfaces. The accumulation of both bulk and interfacial water molecules has been confirmed by many experiments Citation16, Citation17. The increase in resistance can be attributed to the reduction in interchain connectivity of the polymer network due to interstitial water molecules and low connectivity between metal nanoparticles for the composite samples. For pure conducting polymer, with the reduction in interchain connectivity conduction is mainly dominated by tunnel effect. At this regime, electronic conduction through the polymer networks dominates rather than due to metal nanoparticles. However, with the increase RH value, partial water vapour pressure forces the water to penetrate at the core of the metal particles and subsequently modifies the interface of metal nanoparticles with better connectivity. This results into the shorting effect causing the resistance to decrease Citation17. Of course, when the concentration of metal fillers increases, the increasing number of ohmic conductive paths leads to the rapid decrease of resistance and hence the sharper response of the sensor material with RH values. The threshold value of RH decreases with the increase in metal concentration in the nanocomposites. This further explains the shorting effect, which occurs at a lower content of water molecules (i.e at lower RH) present in the sample when the metal concentration is large.
4. Conclusions
In summary, humidity-sensing characteristics of conducting PPY-silver nanocomposites with different silver concentrations is reported here. The results show that addition of silver into the conducting polymer network shows significant effect in the I–V curves as well as in the resistance versus RH data. The variation in resistance of conducting PPY-silver nanocomposites is demarcated by two distinct regimes. The resistance of the nanocomposites is found to increase with RH until a threshold value is reached, above which opposite trend is observed. The threshold RH value is significantly reduced with the increase in silver concentration. The modified metal–polymer interface and the associated interfacial water molecules play the critical role in determining the electrical transport and hence the humidity-response characteristics of the nanocomposites.
Acknowledgements
The authors acknowledge with thanks to Council of Scientific and Industrial Research (CSIR), Government of India, New Delhi, for giving financial support to this research program, under Grant No. 03/1038/05-EMR-II.
References
- Cochrane , C , Koncar , V , Lewandowski , M and Dufour , C . 2007 . Design and development of a flexible strain sensor for textile structures based on a conductive polymer composite . Sensors , 7 : 473 – 492 .
- Bidan , G . 1992 . Electroconducting conjugated polymers: new sensitive matrices to build up chemical or electrochemical sensors. A review . Sensor. Actuat. B-Chem. , 6 : 45 – 56 .
- Freund , MS and Lewis , NS . 1995 . A Chemically diverse conducting polymer-based “electronic nose” . Proc. Natl Acad. Sci. USA , 92 : 2652 – 2656 .
- Sata , T . 1995 . Possibility for potentiometric humidity sensor of composite membranes prepared from anion-exchange membranes and conducting polymer . Sensor. Actuat. B-Chem. , 23 : 63 – 69 .
- Sershen , SR , Westcott , SL , Halas , NJ and West , JL . 2002 . Independent optically addressable nanoparticle-polymer optomechanical composites . Appl. Phys. Lett. , 80 : 4609 – 4611 .
- Cioffi , N , Farella , I , Torsi , L , Valentini , A and Tafuri , A . 2002 . Correlation between surface chemical composition and vapor sensing properties of gold-flurocarbon nanocomposites . Sensor. Actuat. B-Chem. , 84 : 49 – 54 .
- Elsenbaumer , RL and Reynolds , JR . 1997 . Handbook of Conducting Polymers , Edited by: Skotheim , TA . Vol. 1–2 , New York : Marcel Dekker .
- Dutta , P and Mandal , SK . 2004 . Charge transport in chemically synthesized, DNA-doped polypyrrole . J. Phys. D: Appl. Phys. , 37 : 2908 – 2913 .
- Novak , I , Krupa , I and Chodak , I . 2002 . Investigation of the correlation between electrical conductivity and elongation at break in polyurethane-based adhesives . Synth. Met. , 131 : 93 – 98 .
- Buxton , GA and Balazs , AC . 2004 . Predicting the mechanical and electrical properties of nanocomposites formed from polymer blends and nanorods . Mol. Simulat. , 30 : 249 – 257 .
- Mandal , AR and Mandal , SK . 2007 . Polymer assisted preferential growth of PbS and PbS:Mn nanorods: structural and optical properties . J. Exp. Nanosci. , 2 : 257 – 267 .
- Cho , J-H , Yu , J-B , Kim , J-S , Sohn , S-O , Lee , D.-D and Huh , J-S . 2005 . Sensing behaviors of polypyrrole sensors under humidity condition . Sensor. Actuator. B , 108 : 389 – 392 . (b) I. Krupa and I. Chodak, Physical properties of thermoplastic/graphite composites, Eur. Polym. J. 37225 (2001), pp. 2159–2168
- Zhang , XW , Pan , Y , Zheng , O and Yi , XS . 2000 . Time dependence of piezoresistance for the conductor-filled polymer composites . J. Polym. Sci. B , 38 : 2739 – 2749 .
- Leidheiser , H and Funke , W . 1987 . Water disbondment and wet adhesion of organic coatings on metals: A review and interpretation . J. Oil Color Chem. Assoc. , 70 : 121 – 132 .
- Takahashi , KM and Sullivan , TM . 1989 . AC impedance measurement of environmental water in adhesive interfaces . J. Appl. Phys. , 66 : 3192 – 3201 .
- Takahashi , KM . 1990 . AC impedance measurements of moisture in interfaces between epoxy and oxidized silicon . J. Appl. Phys. , 67 : 3419 – 3429 .
- Berčič , B , Pirnat , U , Kusar , P and Mihailovic , D . 2006 . Transport properties of Mo6S3I6 nanowire networks . Appl. Phys. Lett. , 88 : 173103