Abstract
Nanoscale and bulk SrAl2O4 : Eu2+, Dy3+ were prepared, respectively, by auto-combustion of citrate gelatin (gel) and high temperature solid state method. The crystalline structure was examined by X-ray diffraction. The morphological and size aspects of nanoscale sample were carried out with transmission electron microscopy. Spectral shift of nanoscale phosphor was confirmed by measuring excitation and emission spectra. The current-voltage characteristic and optical power were measured with a Keithley source meter 2410. Ionic conductivity plays the leading role when the sample is not irradiated by light and electron conductivity should be in the charge of the current gain when the sample is irradiated by light. The result showed that light irradiation simultaneously increased the luminescence intensity and the current. The current increasing range of nanoscale phosphor is smaller than that of bulk phosphor. It was further concluded that the excited state energy levels of emission centre overlap partly with the conduction band of host crystal. Otherwise, surface effect was analysed deeply by comparing the decay curves of nanoscale and bulk SrAl2O4 : Eu2+, Dy3+.
1. Introduction
Long persistent phosphor absorbs energy from sunlight and releases it in the darkness. As a kind of environment-friendly material, it has very high commercial value. Many researchers have focused on it Citation1–4. Compared with sulphide long persistent phosphor, alkaline earth aluminate long persistent phosphor is much stabler and brighter and has a much longer decay time. It is tending to replace the outdated sulphide Citation5–7. However, many aspects of the persistent luminescence mechanism still remain unclear and the presented theories are even contradictory Citation8,Citation9.
In recent years, many researchers have began to pay more and more attention to nanoscale phosphor because it has some characteristics different from bulk material Citation10–13. In this work we report, for the first time, the electrooptical characteristics of SrAl2O4 : Eu, Dy, which are helpful for probing and understanding the mechanism in depth.
2. Experiments
2.1. Synthesis of nanoscale and bulk SrAl2O4 : Eu, Dy
Eu(CH3COO)3 · 3H2O (99.99%), Dy(CH3COO)3 · 3H2O (99.99%), analytically pure Sr(NO3)2, Al(NO3)3 · 9H2O and citric acid were used as starting materials. Deionised water was used as solvent. Ammonia was used to adjust pH value.
Eu(CH3COO)3 · 3H2O, Dy(CH3COO)3 · 3H2O, Sr(NO3)2 and Al(NO3)3 9H2O were dissolved in water on the nominal composition of Sr0.97Al2O4 : Eu0.01, Dy0.02, two times citric acid of all metal ions was added. The pH value was adjusted to 7. The resultant transparent solution was refluxed for 4 h at 60°C and then was slowly concentrated at 80°C until semitransparent gel appeared in the system. Dried gel was obtained by heating the wet gel for 48 h at 120°C in a drying oven. The precursor was resulted after prefiring the dry gel for 2 h at 700°C. Nanoscale SrAl2O4 : Eu2+, Dy3+ was obtained after sintering the precursor for 3 h at 1000°C in active carbon atmosphere.
The bulk material was prepared by traditional high temperature solid state method.
2.2. Characterisations
Crystal structure of the samples was checked by Rigaku D/max-2000 X-ray diffraction (XRD), using Cu Kα of 1.5405 Å as the X-ray source. The particle size and morphology of nanoscale phosphor were measured by JEM-2000FX transmission electron microscope.
2.3. Electrooptical measurements
The excitation and emission spectra were measured at room temperature using a Flurolog-3 fluorescent spectrometer with a Xe lamp as light source. The nanoscale and the bulk powders were pressed into tablets, the optical power and the current–voltage characteristics were measured with an I–V–L measurement system and a Keithley source meter 2410.
3. Results and discussion
3.1. Crystal structure and size
shows the XRD patterns of the prepared nanoscale and bulk crystals. The diffractive peaks in the two curves are in good accordance with the JCPDS card of 34-0379, which indicates that the nanocrystal has the same crystal type as bulk one. Both of them are well crystallised in cubic structure. Thus, they can have comparability in every aspect.
The mean crystalline size can be deduced according to the Sherrers’ equation D = Kλ/βcos θ. The mean calculated crystalline size of the nanoparticles is 26 nm, which is basically in accordance with which is seen in the transmission electron microscopy (TEM) (). It is clear that the size distribution is homogeneous.
3.2. Electrooptical characteristics
shows the excitation and the emission spectra. The emission at 520 is attributed to the typical 4f65d1-4f7 transition of Eu2+. Although the 5d electrons are exposed out of Eu2+, the energy level difference between the lowest excited state 4f65d1 and ground state 4f7 does not change clearly. So the emission spectrum of nanoscale SrAl2O4 : Eu, Dy is essentially coincident with that of the bulk phosphor. However, the 5d energy level has close relation to the conduction band of host lattice. In addition, The band gap of SrAl2O4 will increase if the crystal size decreases to nanometre and the energy levels of doped Eu2+ ions will shift upward correspondingly Citation14. So the energy centre of 4f65d shifts upwards clearly because of the surrounding crystal field decreasing. As a result, the peak value of the excitation spectrum for nanometre SrAl2O4 : Eu, Dy shifts to the shorter wavelength.
Figure 3. The normalised excitation spectra monitored at 520 nm and the emission spectra excited at 360 nm of nanoscale and bulk SrAl2O4 : Eu2+, Dy3+.
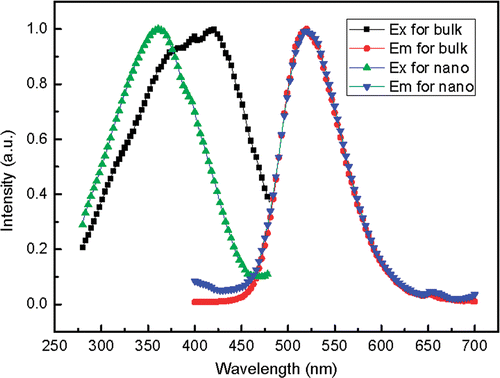
SrAl2O4 has a wide band gap (6.52 eV) Citation14, the light ranging from 280 to 480 nm cannot excite the electrons from the valence band to the conduction band. According to the above analysis, it is the electrons of emission centre (Eu2+) that is excited but not that of host crystal. If these electrons do not relate the emission centres with the electron traps, the exciting light would not intensify the afterglow. In fact, it was found in the experiment that all the exciting light could give intensification of afterglow. In view of this, some of the electrons must have been to the trap level. In order to confirm how the excited electrons get to the trap level, we design the following experiment.
The synthesised bulk and nanoscale powders were pressed into pallets with the same diameter and thickness. The two electrodes of Keithley source meter 2410 cramped either of the two pallets. and show the current-voltage characteristics in dark and in light. In general, SrAl2O4 has a very wide bandgap and the theoretical current should be very weak. Here the experimental value reaches 10−7 order of magnitude. In fact, only electron conductivity cannot give such a high conductivity. It is considered that ionic conductivity plays the leading role when the sample is not irradiated by light. It can also be seen in and that the current of the pallet radiated by light is higher than that in dark under the same voltage, which is fit for both nanoscale and bulk materials. This phenomenon indicates that light irradiation can increase current, that is to say, the electrons are excited to the conduction band of host lattice. This conductivity is electron conductivity. The increased range of nanoscale phosphor is smaller than that of bulk phosphor because the energy level difference between the excited state and the ground state increases with size decreasing. In addition, the built in voltage comes into being and increases as external voltage. The current gain decreases at first and then reaches a stable value, so the slopes of the I–V curves decrease at first and then get stable.
The optical power characteristic was measured by maintaining the voltage at 0 V and the inspected wavelength at 520 nm which is the characteristic emission of the afterglow. The time delay designed was 0.2 s. We defined the horizontal coordinate as time by 0.2 multiplied by a positive integer. It can be seen that the initial intensity of bulk material is stronger than that of nanoscale one and the former decays more slowly than the latter (). The numbers of surface bonds, surface defects and surface absorptions of hydrated or carbohydrate species increase suddenly when the particle size decreases, which leads to non-radiative relaxation Citation15–17. As a result, the initial emission intensity decreases greatly when the size decreases to nanometre. At the same time, the energy transmission between luminescence centre and surface quenching centre increases the phosphorescence decay. In short, surface effect decreases the initial luminance power and increases the rate of decay Citation18,Citation19.
3.3. Theoretical model
The electrons in the valence band of host lattice or the ground state of activator ions are excited to the conduction band, then they migrate in the conduction band and some of them relax to traps and are stored there. Afterwards, these electrons may be released slowly by heat back to the conduction band, migrate and relax to the excited state of the activators. The irradiative transition from the excited state to the ground state is the long persistent luminescence. The key point is that the excited state energy levels of the emission centre overlap with the conduction band of host crystal ().
4. Conclusion
Nanoscale SrAl2O4 : Eu, Dy long persistent phosphor was prepared by auto-combustion of Citrate Gels. It has the same emission peak at 520 nm as bulk material, but the excitation spectrum shifts to the shorter wavelength because of energy level shifting. Surface effect decreases the initial optical power and increases the rate of decay. The electrons always arrive at conduction band at first after they are excited by light irradiation. The excited state energy levels of the emission centre overlap partly with the conduction band of host crystal.
Acknowledgements
The authors express their thanks to the support extended by the National Natural Science Foundation of China (10774012) and Beijing Jiaotong University Program (2007XM048, 2006XM038).
References
- Chang , C and Mao , D . 2004 . Long lasting phosphorescence of Sr4Al14O25:Eu2+,Dy3+ thin films by magnetron sputtering . Thin Solid Film , 460 : 48 – 52 .
- Lü , X . 2005 . Silica encapsulation study on SrAl2O4:Eu2+, Dy3+ phosphors . Mater. Chem. Phys. , 93 : 526 – 530 .
- Nag , A and Kutty , TRN . 2003 . Role of B2O3 on the phase stability and long phosphorescence of SrAl2O4:Eu, Dy . J. Alloys Compd. , 354 : 221 – 231 .
- Jia , D . 2003 . Charging curves and excitation spectrum of long persistent phosphor SrAl2O4:Eu2+, Dy3+ . Opt. Mater. , 22 : 65 – 69 .
- Escribano , P , Marchal , M , Sanjuán , ML , Gutiérrez , PA , Julián , B and Cordoncillo , E . 2005 . Low-temperature synthesis of SrAl2O4 by a modified sol–gel route: XRD and Raman characterization . J. Solid State Chem. , 178 : 1978 – 1987 .
- Chang , C , Yuan , Z and Mao , D . 2006 . Eu2+ activated long persistent strontium aluminate nano scaled phosphor prepared by precipitation method . J. Alloys Compd , 415 : 220 – 224 .
- Hölsä , J , Jungner , H , Lastusaari , M and Niittykoski , J . 2001 . Persistent luminescence of Eu2+ doped alkaline earth aluminates, MAl2O4:Eu2+ . J. Alloys Compd , 323-324 : 326 – 330 .
- Arellano-Tánorr , O , Meléndrez , R , Pedroza-Montero , M , Castañeda , B , Chernov , V , Yen , WM and Barboza-Flores , M . 2008 . Persistent luminescence dosimetric properties of UV-irradiated SrAl2O4:Eu2+, Dy3+ phosphor . J. Luminescence , 128 : 173 – 184 .
- Peng , T , Liu , H , Yang , H and Yan , C . 2004 . Synthesis of SrAl2O4:Eu, Dy phosphor nanometer powders by sol–gel processes and its optical properties . Mater. Chem. Phys. , 85 : 68 – 72 .
- Peng , T , Yang , H , Pu , X , Hu , B , Jiang , Z and Yan , C . 2004 . Combustion synthesis and photoluminescence of SrAl2O4:Eu,Dy phosphor nanoparticles . Mater. Lett. , 58 : 352 – 356 .
- Tang , Z , Zhang , F , Zhang , Z , Huang , C and Lin , Y . 2000 . Luminescent properties of SrAl2O4 : Eu, Dy material prepared by the gel method . J. Eur. Ceram. Soc. , 20 : 2129 – 2132 .
- Sophya Preethi , KR , Lu , C , Thirumalai , J , Jagannathan , R , Natarajan , TS , Nayak , NU , Radhakrishna , I , Jayachandran , M and Trivedi , DC . 2004 . SrAl4O7:Eu2+ nanocrystals: Synthesis and fluorescence properties . J. Phys D Appl. Phys. , 37 : 2664 – 2669 .
- Chang , C , Xu , J , Jiang , L , Mao , D and Ying , W . 2006 . Luminescence of long-lasting CaAl2O4:Eu2+,Nd3+ phosphor by co-precipitation method . Mater. Chem. Phys. , 98 : 509 – 513 .
- Fu , Z , Zhou , S , Pan , T and Zhang , S . 2005 . Band structure calculations on the monoclinic bulk and nano-SrAl2O4 crystals . J. Solid State Chem. , 178 : 230 – 233 .
- Kim , JS , Kim , JS and Park , HL . 2004 . Optical and structural properties of nanosized ZnGa2O4:Cr3+ phosphor . Solid State Commun. , 131 : 735 – 738 .
- Hu , H and Zhang , W . 2006 . Synthesis and properties of transition metals and rare-earth metals doped ZnS nanoparticles . Opt. Mater. , 28 : 536 – 550 .
- Fan , L , Song , H , Li , T , Yu , L , Liu , Z , Pan , G , Lei , Y , Bai , X , Wang , T , Zheng , Z and Kong , X . 2007 . Hydrothermal synthesis and photoluminescent properties of ZnO nanorods . J. Luminescence , 122–123 : 819 – 821 .
- Huang , S , You , F , Peng , H , Luo , W , Feng , Y , Lü , S , Sun , L and Yan , C . 2007 . Influence of surface on spectroscopic properties of rare earth ions in nanocrystals . J. Chin. Rare Earth Soc. , 25 : 396 – 401 .
- Gao , C , Huang , S , You , F , Kang , K and Feng , Y . 2008 . Influence of surface quenching effects on luminescent dynamics of ZnS:Mn2+ . Chinese Phys. Lett. , 25 : 698 – 699 .