1. Introduction
Aptamers are naturally occurring or artificially selected structured nucleic acid ligands that bind targets with a high affinity and specificity. Artificially selected aptamers can be applied to the specific detection, inhibition, and characterization of proteins, functioning like antibodies. These characteristics are very attractive for developing pharmaceutical leads, detection reagents, and functional proteomic tools.
For more than two decades, the development of aptamers has been constrained by patent protections of the aptamer selection process known as ‘Systematic evolution of Ligands by EXponential Enrichment (SELEX).’ However, with the recent expiration of SELEX patents, aptamers are expected to be used to develop human diagnostics and therapeutics. According to a market research report by ‘MarketsandMarkets’ published in 2015 (report code: BT 3550), the global aptamer market is expected to reach $244.93 million by 2020.
Since the Precision Medicine Initiative was unveiled in 2015, future therapeutics are expected to be tailored to unique genetic changes in each patient, instead of a one-size-fits-all approach. Thus, selecting aptamers tailored to somatic mutated targets with chemical modification is a promising new strategy to accelerate the development of therapeutic aptamers for future medicine.
2. Functionalized aptamers for precision medicine
Precision medicine aims to target specific cells and minimize damage to normal tissues. It also aims to identify diagnostic and treatment platforms whose central focus is on the individual disease variability. In the field of precision medicine, theranostics initiated in 2002 is an emerging concept to combine both a diagnostics and therapeutics for more specific and individualized treatment for the clinic. As active targeting moieties, aptamers against cell-surface receptors have been functionalized. Such functionalized aptamers have shown great success as theranostic tools for targeted therapeutics [Citation1], bioimaging [Citation2], and even macromolecule delivery [Citation3].
Genomic sequencing of an individual patient allows researchers to identify patient-specific somatic mutations. Such technologies identify biomarkers such as mutated gene products, differentially expressed proteins, and altered cell-surface antigens. These biomarkers may be drug-specific targets that can directly affect the response of a patient’s disease tissue to a therapeutic regimen. Aptamers targeting point-mutated protein [Citation4] and misfolded proteins [Citation5] might be served as a personalized treatment that affects therapeutic responses.
The US FDA recently approved immune checkpoint antibodies against cytotoxic T-lymphocyte-associated protein 4, programmed death 1 (PD-1,), and programmed death 1 ligand for use in clinical cancer therapy. However, administration of immune checkpoint blockade with antibodies is associated with immune-related adverse toxicity and resistance [Citation6] in patients. Immune checkpoint blockades aptamer against PD-1 [Citation7], T-cell immunoglobulin and mucin-domain containing-3 [Citation8], and other immunotherapeutic aptamers [Citation9] offer desired specificity and low immunogenicity as an emerging immunotherapeutic platform.
3. Chemically modified aptamers
As aptamers are nucleic acid ligands, the in vivo therapeutic potency is crucially limited by their physicochemical characteristics. Therefore, various chemical modifications have been developed to improve serum stability [Citation1]. Most of aptamers in clinical studies are chemically modified by replacing the hydroxyl group at the 2′-position with 2′-fluoro (2′F), 2′-O-methyl (2ʹOMe), or 2ʹ-amino (2ʹNH2) groups [Citation1]. Slow Off-rate Modified DNA Aptamers [Citation10] containing a positively charged modification at the 5′-position of deoxyuridine have showed the therapeutic potential in cynomolgus monkeys [Citation10]. To incorporate modified nucleotides into aptamers during the SELEX, the development of tolerable polymerase have been accompanied. 2ʹF, 2ʹOMe, and 2ʹNH2 nucleotides have been incorporated into RNA aptamers with Y639F mutant T7 RNA polymerase [Citation1]. Other chemically modified nucleotides such as 2ʹ-deoxy-2ʹ-fluoroarabinonucleotide [Citation11], 2ʹ-O,4ʹ-C-methylene bridged/locked nucleic acid (2ʹ,4ʹ-BNA/LNA) [Citation12], and C2ʹ-O-methyl (C2ʹ-OMe)/C2ʹ-fluorine (C2ʹ-F) [Citation13] have been incorporated into aptamers with engineered polymerase. The 2ʹ-O-carbamoyl uridine (Ucm) is successfully incorporated by a wild-type T7 RNA polymerase [Citation14].
4. Conclusion
The recently expired SELEX patent would help promote the development of therapeutic aptamers. As disease-specific functionalized aptamers improve the efficacy of intervention for targeted therapeutics and theranostics in the era of precision medicine, aptamer selection against somatic mutated antigens is necessary to take full advantage of the high specificity of aptamers. In addition, employing chemically modified nucleotides into aptamers is required for the implementation of therapeutic aptamers in preclinical and clinical trials.
5. Expert opinion
Aptamers as therapeutic molecules hold great promise for the future of medicine. The field of aptamers is currently expanding, but has not yet come of age. Currently, multiple pharmaceutical companies and academics actively participate in developing aptamers worldwide. To develop therapeutic aptamers for the future medicine, two strategies are required to develop.
First, taking advantage of high specificity of aptamers. Although the high specificity of aptamers is the compelling features of aptamers for precision medicine, this property might have turned out to be double-edged sword. When the first aptamer drug (Macugen®, an anti-VEGF (anti-vascular endothelial growth factor) aptamer) was approved to treat all types of neovascular age-related macular degeneration (AMD) in 2004, it was considered a revolutionary treatment. However, Macugen was overshadowed by improved clinical success with the off-label use of Avastin® (Genentech/Roche), a full-length anti-VEGF antibody, for treatment of AMD [Citation15]. Because Macugen specifically binds to the heparin-binding domain of only the most abundant isoform of VEGF-A (VEGF165) [Citation16]. In contrast, the binding site of Avastin is in the receptor-binding region of VEGF, and neutralizes all human VEGF-A isoforms [Citation17]. Therefore, the selection of the specific target of interest is a critically important matter. For developing broadly neutralizing aptamers, it may be best practice to select against a common hot spot, while considering biological and functional variance.
Recent advances in next-generation sequencing and epitope prediction will allow identification of mutant neoantigens. Therefore, it is clear that these disease-specific mutations are ideal targets for functionalizing aptamers. Theranostics would integrate disease diagnostics and therapeutics in a single system. Thus, engineered aptamer with multicomponent system in which targeting, imaging diagnostic, and therapeutic is expected to expand in the near future. summarizes the strategies targeting newly revealed disease-specific mutations with aptamers that might be useful for the development of focused therapeutics and theranostics. As yet unexplored options to functionalize aptamers for improved therapeutic interventions are antisense oligonucleotides (ASOs) and extracellular vesicles (EVs). ASOs in the therapeutics field have seen remarkable progress over the past few years, with improved potency, stability, and biodistribution, and minimized toxic effects. However, effective delivery of ASOs to their target, while minimizing exposure of other tissues, remains a major impediment. Chimerization of aptamers with ASOs will be an interesting approach for future strategies to functionalize aptamers. EVs such as exosomes and microvesicles are biologically active and intrinsically transport cargos. However, the use of EVs as delivery cargos has mainly remained in the realm of nontargeted delivery. To use EVs as targeted delivery cargos, aptamers against EV membrane markers suggest a promising approach for therapeutic delivery of EVs.
Figure 1. Schematic diagram depicting theranostic aptamers. Functionalizing aptamers against mutant antigens allows us to deliver theranostics such as imaging agents, drugs, antisense oligonucleotides (ASOs), and extracellular vesicles (EVs) to target cells specifically. This approach can also inhibit the function of mutant intracellular proteins.
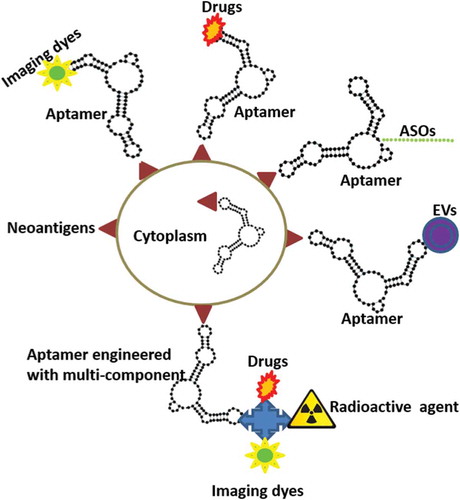
The efficacy of immune checkpoint blockade is widely variable across individuals, even though immune checkpoint blockades have provided substantial clinical benefit. In this regard, for more precise immunotherapies and optimal use, additional immune blockade aptamers would be developed. The targets of interest for immunotherapeutic aptamer are listed in .
Table 1. Immune blockade targets for immunotherapeutic aptamers.
Second, improvement of the serum stability and pharmacokinetics. To increase serum stability, in-SELEX and post-SELEX have been applied to incorporate modified nucleotide into aptamers. So far, it is very encouraging that 2′F, 2ʹOMe, 2ʹNH2 [Citation1], and newly developed modified nucleotides [Citation11–Citation14] have been successfully incorporated with the engineered polymerases in the in-SELEX. Currently available modified nucleotides are modified at the 2′-position of ribose. As the 2ʹ-modification can change RNA structure flexibility [Citation18], the structural stability of aptamers incorporating with recently developed modified nucleotides [Citation11–Citation14] remains to be validated.
Post-SELEX modification can result in loss of activity and, even if it succeeds, is a time-consuming process that requires the iterative examination of different substitutes at different positions to determine which combination is tolerated. In this respect, application of click-SELEX [Citation19] is a very interesting concept that enables introduction of multiple alkyne functional groups. Typically, the SELEX tediously repeats between 6 and 20 rounds of selection. To avoid the laborious SELEX procedures, the big movement of the SELEX method is to automate for the high-throughput discovery of aptamers [Citation20]. For the personalized aptamer selection, combining high-throughput discovery of aptamers [Citation20] with click-SELEX [Citation19] will be a very powerful and efficient tool.
To increase the pharmacokinetics of aptamers, polyethylene glycol(PEG)ylation of aptamer has been employed. But, unfortunately, PEGylation has induced severe allergic reactions, which are associated with preexisting antibodies to PEG [Citation21]. Herein, PASylation (addition of Pro/Ala/Ser polypeptide biopolymers) might be an effective biological alternative, as hydrophilic and uncharged biological polymers show serum stability, lack toxicity and immunogenicity [Citation22].
Despite extensive research in the field of therapeutic aptamers, translation to clinics remains very limited, which may be due to the tedious optimization step, limited stability of aptamers, and poorly selected targets of interest. Even though optimization of aptamer with chemically modified nucleotides remains an impediment, there are benefits after optimization: low manufacturing costs, high purity, and sustainability. Despite these limitations, aptamers remain promising therapeutic molecules for the future of medicine. In our opinion, automation of personalized aptamers with chemical modification would provide the best strategies for therapeutic aptamer development.
Declaration of interest
John J. Rossi (City of Hope) is co-founder of Apterna Ltd. in the United Kingdom. JJ Rossi and S Yoon hold stock in Apterna Ltd. The authors have no other relevant affiliations or financial involvement with any organization or entity with a financial interest in or financial conflict with the subject matter or materials discussed in the manuscript apart from those disclosed.
Acknowledgment
The authors are grateful to Sarah T Wilkinson from City of Hope for her language editing.
Additional information
Funding
References
- Zhou J, Rossi J. Aptamers as targeted therapeutics: current potential and challenges. J Clin Oncol. 2016. DOI:10.1038/nrd.2016.199
- Xiang D, Shigdar S, Qiao G, et al. Nucleic acid aptamer-guided cancer therapeutics and diagnostics: the next generation of cancer medicine. Theranostics. 2015;5:23–42.
- Heo K, Min SW, Sung HJ, et al. An aptamer-antibody complex (oligobody) as a novel delivery platform for targeted cancer therapies. J Control Release. 2016;229:1–9.
- Chen L, Rashid F, Shah A, et al. The isolation of an RNA aptamer targeting to p53 protein with single amino acid mutation. Proc Natl Acad Sci U S A. 2015;112:10002–10007.
- Madsen JB, Andersen LM, Dupont DM, et al. An RNA aptamer inhibits a mutation-induced inactivating misfolding of a serpin. Cell Chem Biol. 2016;23:700–708.
- Postow MA, Callahan MK, Wolchok JD. Immune checkpoint blockade in cancer therapy. J Clin Oncol. 2015;33:1974–1982.
- Prodeus A, Abdul-Wahid A, Fischer NW, et al. Targeting the PD-1/PD-L1 immune evasion axis with DNA aptamers as a novel therapeutic strategy for the treatment of disseminated cancers. Mol Ther Nucleic Acids. 2015;4:e237.
- Hervas-Stubbs S, Soldevilla MM, Villanueva H, et al. Identification of TIM3 2ʹ-fluoro oligonucleotide aptamer by HT-SELEX for cancer immunotherapy. Oncotarget. 2016;7:4522–4530.
- Khedri M, Rafatpanah H, Abnous K, et al. Cancer immunotherapy via nucleic acidaptamers. Int Immunopharmacol. 2015;29:926–936.
- Hirota M, Murakami I, Ishikawa Y, et al. Chemically modified interleukin-6 aptamerinhibits development of collagen-induced arthritis in cynomolgus monkeys. Nucleic Acid Ther. 2016;26:10–19.
- Alves Ferreira-Bravo I, Cozens C, Holliger P, et al. Selection of 2ʹ-deoxy-2ʹ-fluoroarabinonucleotide (FANA) aptamers that bind hiv-1 reverse transcriptase with picomolar affinity. Nucleic Acids Res. 2015;43:9587–9599.
- Kuwahara M, Obika S. In vitro selection of BNA(LNA) aptamers. Artif DNA PNA XNA. 2013;4:39–48.
- Chen T, Hongdilokkul N, Liu Z, et al. Evolution of thermophilic DNA polymerases for the recognition and amplification of C2ʹ-modified DNA. Nat Chem. 2016;8:556–562.
- Masaki Y, Ito H, Oda Y, et al. Enzymatic synthesis and reverse transcription of RNAs incorporating 2ʹ-O-carbamoyl uridine triphosphate. Chem Commun. 2016;52:12889–12892. DOI:10.1039/c6cc05796a
- Nagpal M, Nagpal K, Nagpal PN. A comparative debate on the various anti-vascular endothelial growth factor drugs: pegaptanib sodium (macugen), ranibizumab (lucentis) and bevacizumab (avastin). Indian J Ophthalmol. 2007;55:437–439.
- Lee JH, Canny MD, de Erkenez A, et al. A therapeutic aptamer inhibits angiogenesis by specifically targeting the heparin binding domain of VEGF165. Proc Natl Acad Sci U S A. 2005;102:18902–18907.
- Wang Y, Fei D, Vanderlaan M, et al. Biological activity of bevacizumab, a humanized anti-vegf antibody in vitro. Angiogenesis. 2004;7:335–345.
- Masaki Y, Miyasaka R, Ohkubo A, et al. Linear relationship between deformability and thermal stability of 2ʹ-O-modified RNA hetero duplexes. J Phys Chem B. 2010;114:2517–2524.
- Tolle F, Brandle GM, Matzner D, et al. A versatile approach towards nucleobase-modified aptamers. Angewandte Chemie. 2015;54:10971–10974.
- Csordas AT, Jorgensen A, Wang J, et al. High-throughput discovery of aptamers for sandwich assays. Anal Chem. 2016;88:10842–10847.
- Ganson NJ, Povsic TJ, Sullenger BA, et al. Pre-existing anti-polyethylene glycol antibody linked to first-exposure allergic reactions to pegnivacogin, a pegylated RNA aptamer. J Allergy Clin Immunol. 2016;137:1610–1613.
- Schlapschy M, Binder U, Borger C, et al. Pasylation: a biological alternative to pegylation for extending the plasma half-life of pharmaceutically active proteins. Protein Eng Des Sel. 2013;26:489–501.