ABSTRACT
Introduction: Chronic, nonhealing skin wounds claim >3% of the health-care budget in industrialized countries, and the incidence is rising. Currently, two parallel trends influence innovations within the field of wound healing: the need to reduce spread of antibiotic resistance and the emerging use of health economy and value-based models.
Areas covered: This review focuses on the discovery of drug candidates and development of treatments aiming to enhance wound healing in the heterogeneous group of patients with nonhealing wounds.
Expert opinion: Nonhealing wounds are multifaceted and recognized as difficult indications. The majority of products currently in use are medical device dressings, or concepts of negative pressure or hyperbaric oxygen treatment. Global best practice guidelines for the treatment of diabetic foot ulcers recommend debridement, redressing, as well as infection control, and are critical to the lack of coherent clinical evidence for many approved products in active wound care. To accelerate wound healing, there is an emerging trend toward biologics, gene therapy, and novel concepts for drug delivery in research and in the pipeline for clinical trials. Scientific delineation of the therapeutic mechanism of action is, in our opinion, vital for clinical trial success and for an increased fraction of medical products in the pharmaceutical pipeline.
1. Introduction
The skin forms a physical barrier crucial for protection against microbial invasions, as well as maintaining temperature and fluid homeostasis. Skin wounds transiently destroy this barrier, and thereby comprise a great health challenge. Historically, skin wounds were the major cause of gangrene and of septic death [Citation1]. Understandably, treatments intending to improve healing of such skin wounds have a long history. Lowering of wound pH is acknowledged to counteract wound infections and accelerate wound healing and was first mentioned by Hippocrates who recommended vinegar to clean the wounds before applying dressings in the form of fig leaves [Citation2]. Current drug development, aiming to accelerate wound healing, often finds inspiration in the past. For instance, herbs used to heal wounds within traditional Ayurvedic medicine are today being screened scientifically in the hunt for active substances, but presently those substances available in the clinic.
Wounds can be divided into acute and nonhealing chronic wounds depending on the time to healing. If the wounds do not heal within 3 months, they are referred to as chronic, nonhealing wounds. Nonhealing wounds are often coupled to underlying pathologies associated with reduced healing abilities (as in persons with arterial or venous insufficiencies or persons having diabetes or receiving systemic steroid treatment) and/or increased susceptibility for acquiring wounds (e.g. peripheral neuropathies) [Citation3]. Patients developing nonhealing wounds form a heterogeneous group, and nonhealing wounds are commonly divided into pressure ulcers, venous leg ulcers, arterial ulcers, and diabetic foot ulcers (DFUs). Nonhealing wounds do not only cause discomfort, pain, and increased risk for amputation, but are also associated with substantial health-care costs. Wound care is divided into different treatment types depending on its purpose (): traditional, advanced, and active wound care. Traditional wound care is applied on acute wounds and only aims to cover and protect the wounds. If the wounds fail to heal, advanced wound care in the form of dressings is used to keep wounds moist. For the last segment of nonhealing wounds, active wound care aims at accelerated healing. According to a recently published systematic review of studies from all continents with the majority from the USA, the annual mean cost for a nonhealing wound was established to be $23 300 per afflicted patient ranging from $1800 to $61,500 [Citation4]. In Europe, the prevalence for nonhealing wounds is estimated between 1% and 2%, and associated total costs thereby become extensive [Citation5–Citation8].
Figure 1. Wound healing occurs in different but overlapping phases involving distinct cells and effector mechanisms that all are required for efficient wound healing. The type and purpose of wound care applied on different time points following wounding are also indicated.
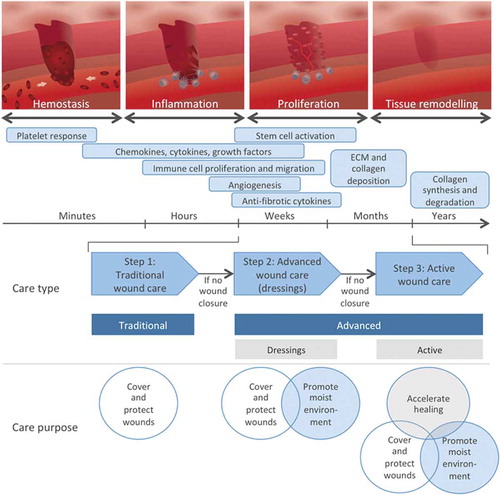
1.1. Wound healing
Wound healing is a complex process characterized by dynamic changes of the wound microenvironment to recruit and direct the different participating cell types. The entire process is classically divided in four consecutive and overlapping phases: hemostasis, inflammation, proliferation, and remodeling () [Citation9,Citation10]. During the hemostasis phase, the bleeding is controlled by sympathetic-induced vasoconstriction followed by clot formation [Citation10,Citation11]. Cells of wounded tissue release alarm signals, chemokines, and growth factors that recruit immune cells from blood circulation and stimulate the proliferation of tissue resident populations, resulting in immune cell accumulation at the wound site [Citation12,Citation13]. The proliferation phase is characterized by the formation of granulation tissue that consists of newly formed blood vessels, immune cells, and fibroblasts, and allows epidermal cell migration on top of this tissue during re-epithelialization of the wound. To strengthen the repaired tissue, fibroblasts in the dermis and epidermis deposit new extracellular matrix during the remodeling phase when the scar tissue is also formed [Citation9]. The structure and composition of skin differs between species, as do the rate of healing [Citation14].
Nonhealing wounds are, when compared to healing wounds, associated with high levels of proteolytic enzymes [Citation15], bacterial colonization, and formation of biofilms [Citation16], as well as altered cell signaling [Citation17]. As a consequence, the sequential changes of the microenvironment depicted in are hampered, resulting in failure to heal. Interestingly, the vast majority of nonhealing wounds contain biofilms [Citation18] that contribute to the prolonged inflammation and pathology of nonhealing wounds [Citation19], as well as favor antibiotic resistance transfer. Biofilms are very persistent and hard to treat as the contained bacteria enter a dormant state where they are more resilient against topical or systemic antibiotics [Citation16,Citation20]. Further, the biofilm matrix also protects contained bacteria from detection by the immune system in the form of antibodies, complement, and phagocytosis. Mechanical debridement in combination with systemic and topical antibiotics is the standard treatment for biofilms [Citation20].
1.2. Immune cells in wound healing
Immune cells have an important role throughout all phases of wound healing. However, it has been shown that their roles and functions vary during the healing process. In addition, functional heterogeneity within the populations of the innate immune cells, namely neutrophils, monocytes, and macrophages, is currently being revealed.
The importance of neutrophils for wound healing is demonstrated in mice genetically deficient in CXCR2, a chemokine receptor important for neutrophil recruitment to the wound site [Citation21]. Using these CXCR2-/- mice, delayed re-epithelialization was reported and resulted in impaired wound healing [Citation21]. However, extended presence of neutrophils is a characteristic of nonhealing wounds, and another study revealed that wound healing was accelerated when neutrophils were depleted at the time of wound induction but restored in overall numbers 5 days later [Citation22]. Increasing age clearly delays wound healing, and a mouse study demonstrated that wound closure in young mice (2 months of age) occurs independently of neutrophils, whereas older mice (6, 10, and 20 months) healed even slower when depleted of neutrophils [Citation23]. Depletion protocols target all neutrophils, and thereby preclude studies addressing the importance of distinct populations with different functions. For instance, a proangiogenic neutrophil population was recently discovered and is recruited from blood circulation to tissue by vascular endothelial growth factor-A (VEGF-A) [Citation24,Citation25]. These neutrophils are very likely to induce angiogenesis during the granulation phase, acting in parallel to classic neutrophils with important bactericidal effects during an interrupted skin barrier.
Macrophages are important in wound healing as they produce many factors that drive the different phases of the healing cascade (). During the wound healing process, the macrophages shift phenotype from pro-inflammatory to a more regulatory phenotype with tissue restorative functions. This was demonstrated in an elegant study wherein macrophages were conditionally depleted during different phases of wound healing, which clearly revealed distinct functions of macrophages in the inflammatory versus the proliferating phase [Citation26]. When macrophages were depleted in the inflammatory phase, the formation of vascularized granulation tissue was reduced, epithelialization was impaired, and the scar formation was minimized. In contrast, depletion of macrophages in the proliferating phase resulted in wound hemorrhage and halting of the wound healing process [Citation26,Citation27]. The different functions of macrophages at different stages of healing are further emphasized by changes in their expression. In the early stages of wound healing, the majority of the macrophages express a pro-inflammatory phenotype characterized by production of pro-inflammatory molecules such as nitric oxide, reactive oxygen species, interleukin-1 (IL-1), IL-6, and tumor necrosis factor-alpha (TNF-α), as well as the matrix metalloproteinases MMP-2 and MMP-9 for matrix degradation. As the wound healing progresses, the macrophages change phenotype and upregulate the expression of factors such as platelet-derived growth factor (PDGF), insulin-like growth factor 1 (IGF-1), VEGF-A, and transforming growth factor-β (TGF-β), as well as the MMP inhibitor TIMP1. During the late stages of wound healing, macrophages have a regulatory role by suppressing inflammation through IL-10 production [Citation28–Citation30], returning the balance of MMP/TIMP expression [Citation29] and reducing fibrosis. Macrophages in nonhealing wounds are to a large degree pro-inflammatory and have been shown to have a reduced ability to phagocyte dead neutrophils, an important trigger for their shift to the regulating resolving phenotype promoting tissue restoration.
Figure 2. Immune cells recruited to the wounded site release agents that are instrumental for all stages of healing. The figure depicts active agents produced by macrophages, of which some have been investigated as treatment to accelerate wound healing.
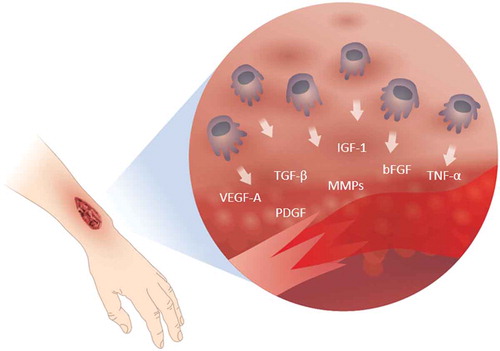
The ability of scar-less healing observed in fetuses has intrigued the field of wound healing for decades [Citation31]. Scar-less healing is also observed experimentally in mice lacking immune cells [Citation32]. The link between the immune system and scar formation is this far elusive; however, it is generally concluded that the immature immune system of fetuses possesses healing abilities that are later lost when they gain classic immune competent functions.
1.3. Wound healing and chemokines, cytokines, and growth factors
Wound healing is a dynamic process that is largely regulated by cellular instructions from the microenvironment. These instructions often come in the form of chemokines, cytokines, and growth factors, which together orchestrate the healing process at different levels.
Chemokines are cytokines with chemotactic properties and are crucial for immune cell recruitment to afflicted sites. They are produced and secreted by activated cells and regulate cell actions through both paracrine and autocrine signaling. Dependent on their structure, chemokines are divided into the following families: CC-, CXC-, C- and CX3C-chemokines [Citation33]. They bind to one or multiple seven loop transmembrane G protein-coupled chemokine receptors [Citation34] that are either abundantly expressed on many cell types or specifically expressed by distinct cells [Citation35,Citation36]. Importantly, secreted chemokines bind negatively charged extracellular matrix glycoproteins, e.g. heparan sulfate, and thereby form haptotactic gradients important for cell migration, but they also undergo processing in tissue by extracellular enzymes depending on the local pH [Citation37–Citation39]. The chemokine profile of nonhealing wounds is altered compared to wounds with normal healing. In effluents from nonhealing wounds or from burn wounds, the pro-inflammatory chemokine CXCL8 was found to be upregulated, while CCL5, CXCL10, and CXCL11 were downregulated in effluents from nonhealing wounds [Citation40].
Growth factors are polypeptides that have a large array of functions. The same growth factor and receptor can activate different pathways and thereby induce different responses depending on the target cell type as well as the microenvironment [Citation41,Citation42]. Growth factors are involved in cell growth, proliferation, differentiation, and migration. Growth factors are essential for normal wound healing and increased expression of certain growth factors is detected in acute wounds, including epithelial growth factor (EGF), basic fibroblast growth factor (bFGF), TGF-β, PDGF, and VEGF. Additionally, expression of the same growth factors are depressed in nonhealing wounds, and they are therefore interesting drug candidates for promoting wound healing [Citation41]. For instance, levels of PDGF were found to be reduced in human nonhealing wounds compared to what is observed in acute surgical wounds [Citation10,Citation41]. This was also observed in two experimental models of delayed wound healing, the db/db mice [Citation43] as well as in mice treated with glucocorticoids [Citation43,Citation44]. Nonhealing wounds of diabetic patients have been demonstrated to contain lower levels of IGF-1 and TGF-β1, and in model with diabetic mice bFGF and VEGF also are reduced [Citation45].
1.4. Wound healing and skin stem cells
As mentioned above, immune cells are known to release cytokines and growth factors such as TGF-βs, IGFs, and FGFs when recruited to wounded skin. These factors promote angiogenesis, migration, and proliferation of keratinocytes and dermal fibroblasts, synthesis of extracellular matrix, and sometimes generation of new hair follicles in the process of epidermal regeneration [Citation46–Citation48]. During healing, cells originating from both hair follicles and interfollicular epidermis migrate into full-thickness wounds [Citation49–Citation54]. The importance of hair follicle stem cell (HFSC) niche is nicely shown in mice lacking either hair follicles or HFSCs, as delayed wound healing was observed [Citation55]. However, very few HFSC-derived cells are found at later stages of repair, indicating that input from hair follicles plays relatively minor roles, whereas cells originating from stem cells located within the interfollicular epidermis play a major role in wound re-epithelialization.
2. Topical medicine for wound healing
Most wounds heal by themselves and the treatment provided to these wounds is therefore restricted to protecting them from the environment. However, for nonhealing wounds a more active treatment promoting healing is needed. The wound care thus depends on the type of wound as well as the purpose of treatment ( and ). While acute and nonhealing wounds are discriminated depending on the time to healing, burn wounds are classified according to the depth of the burn. In superficial burn there is only damage to the epidermis, superficial partial-thickness burns affect the epidermis and papillary dermis, while deep partial-thickness burns also affect the reticular dermis, and full-thickness burns reach the subcutaneous fat. For superficial burns, the standard care is cleaning and debridement of the wound, together with antibiotics if needed. Deep partial-thickness and full-thickness burn wounds are normally treated with topical antimicrobial agents, but if covering a larger area, skin substitutes or skin grafts like autologous split thickness graft are the standard worldwide [Citation56].
For nonhealing wounds, the current standard of care includes repeated dressings changes, wound debridement, antibiotics to treat infections, and avoidance of increased pressure at the injured site [Citation57]. Few pharmaceutical options are today available to accelerate healing, and only four products have been registered for wound healing as active medical products by the Food and Drug Administration (FDA) [Citation58,Citation59]. Due to the huge societal impact of nonhealing wounds, great efforts are being placed on finding means to improve wound healing, as demonstrated in –, and further discussed below. Despite many promising preclinical results, clinical efficacy often remains to be demonstrated. Topical application of therapeutics is appealing as it exerts local effects while limiting systemic side effects, but is simultaneously hampered by the proteolytic wound environment that reduces drug bioavailability. Furthermore, the common and different co-morbidities of persons with nonhealing wounds that in different ways impair healing have greatly impeded the transition of promising preclinical treatments into clinically available regiments. Instead, there has been an extensive development of wound dressings during the last decades, spanning from traditional dry dressings of gauze and plasters that are primarily intended to cover the wound to modern dressings designed to maintain the moist wound environment and promote healing ( and , ) [Citation60]. Modern dressings also aim to convey therapeutic effects such as stimulation of re-epithelialization and collagen synthesis as well as promoting angiogenesis within the wounds, but with so far very limited cohesive clinical support.
Table 1. Different wound dressings available on the market to treat various wounds.
Table 2. Ongoing clinical trials assessing the effect of different medicinal products in wound healing.
Table 3. Completed clinical trials assessing the effect of different medicinal products in wound healing.
2.1. Growth factors
The extensive delineation of the wound healing process has resulted in identification of several growth factors that serve important functions throughout the different stages of wound healing. Even though clinical trials have assessed topical application of growth factors to wounds since the 1980s, only one growth factor, PDGF, was approved by the FDA for treatment of nonhealing wounds [Citation59]. PDGF is the first growth factor to be expressed in response to injury and is important through all phases of wound healing [Citation17]. Becaplerim, a gel containing recombinant PDGF (the BB isoform), was shown to accelerate wound closure in DFUs in several randomized clinical trials, and was approved by the FDA for treatment of DFUs in 1997. Effectiveness of Becaplerim has also been assessed in a retrospective analysis of patients with neuropathic foot ulcers where the treatment increased the healing rate with 32%, which corresponds well to the results of the clinical trials [Citation66]. Despite positive results from clinical trials, this treatment option is not a first-line treatment, probably due to product pricing [Citation59].
One of the major challenges for topical growth factor and chemokine administration is the proteolytic environment of the wound that efficiently degrades delivered proteins [Citation15]. The pro-inflammatory environment in nonhealing wounds further increases the expression of peptidases and proteases, such as MMPs, in the wound, and increased levels of MMPs were demonstrated in biopsies from DFUs compared to biopsies from acute wounds of non-diabetic patients [Citation67]. In parallel, the levels of TIMP-2, an endogenous inhibitor of MMPs, were decreased in DFUs [Citation67]. To overcome the problem of degradation and to increase bioavailability of drug candidates, different delivery methods have been explored during the last decades including sprays, intralesional injections, conjugation to nanoparticles, incorporation into dressings, and on-site production by gene therapy.
There are several clinical studies presenting results from intralesional injections of growth factors. For example, local injections of granulocyte macrophage colony stimulating factor (GM-CSF) in to the wound bed showed efficiency in treatment of chronic venous leg ulcers in a double-blinded, randomized, placebo-controlled clinical study [Citation68]. However, intralesional injections into hyper-sensitized areas such as wounds are associated with significant pain. A less invasive approach is offered by topical sprays aiming to provide a more direct contact between the wound surface and the active drug, the growth factor, than when applied in a cream or gel. Both intralesional injections and sprays decrease the transmission time of growth factor into the wound tissue. Recently, a randomized, double-blinded, placebo-controlled, multi-center clinical study assessing efficiency of an EGF spray applied topically twice a day to DFUs showed increased healing compared to control treated with saline spray, with complete healing rates of 73.2% and 50.6% respectively [Citation69]. In two phase III clinical trials, a spray containing 0.01% bFGF was used to treat DFU, but failed to meet the primary outcome of difference in complete healing over 12 weeks compared to the control group (NCT01217476 and NCT01217463).
Incorporation of growth factors into dressings is an approach that enables a slower release over an extended period of time. Recently, a hydrogel containing GM-CSF has also been demonstrated to increase the healing rate of deep partial-thickness burns [Citation70]. There are also dressings designed to reduce the proteolytic activity within the wound. A planned clinical trial evaluates Becaplerim together with a protease inhibitor wound dressing consisting of 44% oxidized regenerated cellulose (ORC), 55% collagen, and 1% silver-ORC applied three times per day to venous ulcers and DFUs for 6 weeks (NCT02845466).
Gene therapy aims to induce in situ expression of growth factors or chemokines by inserting DNA encoding genes into the cells of the wound tissue by viral or non-viral delivery. In a phase I clinical trial, safety of PDGF gene therapy (the B isoform) was evaluated. In this study, replication-deficient adenoviral vectors encoding human PDGF-B gene were applied topically onto wounds using a bovine type-1 collagen scaffold. The collagen holds the vector in place, thereby limiting the uncontrolled dispersion of the drug substance until the infiltrating cells arrive and the gene is taken up. These cells then function as local bioreactors that produce the PDGF protein [Citation71]. In this study, no difference between single or multi-dose was observed, and no placebo group was included. This study was followed by a phase II clinical trial (NCT00493051) that is completed but no results are reported.
Platelet-rich plasma (PRP) is the plasma fraction that contains higher concentration of platelets and thereby higher levels of platelet-sequestered proteins such as growth factors and chemokines compared to whole blood. Thus, administration of PRP to wounds increases the levels of growth factors in the wound by platelet release of PDGF, VEGF, FGF, hepatocyte growth factor, and TGF-β [Citation72]. Cochranes’ review concludes that topically applied PRP may be effective treatment of DFU, while the efficiency for other chronic wounds was unclear. The number of participants and the quality of the studies were not considered high enough for a confident assessment of the therapeutic ability of PRP in chronic wounds [Citation73]. In 2007, the first system for preparation of PRP was approved as a medical device by the FDA for wound management. This product is currently further evaluated in three clinical trials investigating the efficiency in venous leg ulcers, DFUs, and pressure ulcers (NCT02352467, NCT02352454, and NCT02352480). As recently as last year, FDA approved another PRP preparation kits for preparation of PRP intended for topical treatment of exuding wounds such as leg ulcers, pressure ulcers, and diabetic ulcers and mechanically or surgically debrided wounds [Citation74].
2.2. Chemokines
Several chemokines have also been explored as targets for wound treatment based on promising preclinical observations. For example, experiments have demonstrated that single administration of CCL2 increased the levels of infiltrating macrophages to the wound in the first days after wounding, and accelerated wound healing in db/db mice [Citation75]. In rats, injections in the wound edge of genetically modified mesenchymal stem cells (MSCs) expressing CXCL12 are reported to accelerate wound healing [Citation76]. This approach is excellent for experimental drug delivery, but faces significant difficulties when clinical feasibility and regulatory aspects are taken into account. Treatment with human CXCL12 has been shown to accelerate healing in a porcine full-thickness incisions model were non-viral gene therapy that expressed human CXCL12 were injected into the wound edges [Citation77]. A phase I clinical trial was initiated in 2012, but the current status in unknown (NCT016557045).
Many chemokines are demonstrated to require continous delivery in order to be effective. However, multiple applications per day are not desirable since excessive dressing changes both disturb the healing process and increase the health-care cost [Citation78]. Thus, administration of recombinant proteins is not a clinically relevant option to treat wounds, and instead onsite release from administered cells or adhesives is explored. Using live probiotic Lactobacillus as a vehicle for prolonged protein delivery to the local environment of the wound shows promising results [Citation79]. Topical application of live Lactobacillus reuteri engineered to express human or mouse CXCL12 has demonstrated rapid wound closure in healthy mice, as well as hyperglycemic and ischemic murine models [Citation80]. In addition to the prolonged delivery of chemokines following application on the wound, Lactobacillus produces lactic acid as a natural by-product of their metabolic pathways and thereby decreases pH in the wound microenvironment. The CXCL12 degrading protease DPP4/CD26 was shown to be completely inhibited in pH conditions associated with proliferating L. reuteri [Citation80], and the bioavailability of the delivered chemokine is thereby enhanced by demonstrating synergetic effect of using lactic acid-producing bacteria as protein vectors. Inhibition of the proteolytic enzymes (DPP4/CD26) has yielded interesting results, and improved wound healing was observed in CD26−/- mice [Citation21].
It is well established that prolonged inflammation and production of pro-inflammatory chemokines can lead to nonhealing wounds, and broad-spectrum chemokine inhibitors are therefore tried to limit wound inflammation. The chemokine inhibitor 35K, which inhibits the entire class of CC-chemokines, enhanced wound closure and neovascularization in murine wounds [Citation81]. How this approach influence on wound infections needs to be further established.
2.3. Cell therapy
MSCs derived from different tissues have also been investigated for improving wound healing, as well as to treat other diseases including diabetes. The mechanism of action for this approach is most likely a transient release of anti-inflammatory chemokines and growth factors from these cells. In rats, wound treatment of MSCs has been shown to reduce the number of inflammatory cells, decrease the levels of pro-inflammatory cytokines IL-1 and TNF-α, and increase the levels of anti-inflammatory IL-10. Several studies have also indicated that polarization of macrophages from M1 to M2 is induced by MSC treatment [Citation82]. There are several ongoing phase I or II clinical trials investigating the therapeutic potential of topically applied autologous and allogenic MSCs in wound healing of chronic wounds and second-degree burns ().
Immune cells have likewise been investigated as potential candidates for cell therapy treatment of wounds. Macrophages cultured with TNF-α and IFN-γ were injected into the wound bed of rats with streptozotocin-induced hyperglycemia. Wounds receiving macrophage injections demonstrated accelerated healing compared to untreated wounds [Citation85]. In another study, wounds of healthy or db/db mice were treated with IL-4- or IL-10-activated macrophages, which did not promote healing in healthy mice and even delayed wound healing in db/db mice [Citation86]. In open clinical study, pressure ulcer patients were treated with local injections with a suspension of activated allogeneic macrophages. Macrophages were isolated from healthy donors and activated with hypo-osmotic shock. During the study period, 69.5% of the ulcers healed following treatment with activated macrophages while only 13.3% of the ulcers healed in the control group receiving treatment with standard care only [Citation87].
2.4. MicroRNA
MicroRNAs have the ability to upregulate or suppress gene expression, and different microRNAs will be upregulated or downregulated during the phases of wound healing depending on their function. The miRNA expression in normal, healing wounds has been shown to differ from that of chronic wounds [Citation88]. In patients with nonhealing wounds, the miRNA miR-92a showed twofold higher expression compared to patients with wounds that were healing [Citation89]. In murine models, upregulation of miR-92a decreased angiogenesis while inhibition of miR-92a improved wound healing and angiogenesis [Citation90]. Inhibition of miR-92a with the inhibitor MRG-110 has also demonstrated accelerated wound healing in healthy pigs and diabetic mice [Citation90], and consequently there are ongoing clinical trials with this inhibitor in healthy volunteers (NCT03603431).
2.5. Antimicrobial peptides (AMPs)
AMPs are small peptides that are post-translationally processed from a pre-cursor protein into the active form when local environmental cues signal their release or upregulation. They are part of the innate immune system of most organisms and are among the first defenders to arrive to the site of infection. They have been attributed with many of the roles that other immune factors have, such as immune cell recruitment through chemotaxis, involvement with regulation of chemokines and chemokine receptors, and have also been highlighted for important roles in wound healing and tissue repair, for example, phosphorylation of epidermal growth factor receptor that has been associated with triggering pathways prompting tissue regeneration [Citation91–Citation93]. However, most concede that the primary role of AMPs is defense and subsequent antimicrobial activity. This fact leads to the controversy involving their potential for widespread use in drug development [Citation94]. Targeting nonhealing wounds, which are often infected with multiple microorganisms, is especially concerning when taking into account recent reports of antimicrobial resistance found not only in the experimental setting [Citation94], as a recent report detected a colistin (approved drug where the active substance is an AMP) resistant gene, mcr-1, expressed on a horizontally transferred plasmid. Since then, many more mcr-1 findings have been made as well as several additional mcr genes. This discovery dispelled the long-held belief that AMP resistance was unlikely rare and only found in inherent resistance mechanisms [Citation95]. Additionally, AMP drug development has been hindered by their susceptibility to protease activity and their high levels of cytotoxity [Citation96,Citation97]. Despite the awareness of resistance development using endogenous AMPs, several AMPs are considered potential drug candidates aimed toward wound healing [Citation98]. Pexiganan has a long and troubled drug development history. The cream-based formulation was intended for treatment of DFUs, but it did not pass phase III clinical studies to gain FDA approval based on that it did not reach its primary end point of overall resolution of infection (NCT01590758and NCT01594762). LL-37, an endogenous human AMP, showed significant wound healing of venous leg ulcers in a phase I/II clinical trial with accepted tolerability [Citation99]. Dose–response efficacy demonstrated consistently higher healing rates for lower (0.5 and 1.6 mg/ml) but not higher (3.2 mg/ml) doses of LL-37 compared to placebo, demonstrating that correct dosing is critical for endogenous AMPs.
2.6. Non-endogenous molecules
Non-endogenous molecules that exhibit wound healing properties are also being discovered and developed. Silver containing dressings and topical silver agents are used in the clinic despite the inconclusive nature of evidence to support effectiveness. A systemic review evaluating topical silver on infected wounds together with another in burn wounds both concluded that there are not enough evidence to support silver treatment against wound infections [Citation100,Citation101].
Betulin is isolated from birch bark, and in vitro studies of human keratinocytes demonstrate that betulin enhances keratinocyte migration and upregulation of pro-inflammatory cytokines, such as IL-6, IL-8, and COX-2 [Citation102]. Two parallel clinical phase III studies demonstrated accelerated wound closure following topical treatment with a betulin gel in split-thickness skin graft donor sites (NCT01807650 and NCT01657305) [Citation103].
A synthetic chemical, prifenidone (PFD), was found to modulate the expression of TNF-α, TGF-β, FGF, PDGF, and VEGF, giving it anti-fibrotic and anti-inflammatory properties. In two phase III clinical trials, topical PFD addition to conventional treatment significantly improved healing in DFUs [Citation104,Citation105].
Insufficient circulation is a common characteristic of tissues with nonhealing wounds. In a recent phase III clinical trial, isosorbide dinitrate, a vasodilator of arteries and veins, showed improved healing in DFUs in following topical application in spray format. Greater treatment effects were observed when the treatment was combined with chitosan gel [Citation106].
2.7. Other
Skin substitutes are commonly used to treat larger burn wounds, but are also used for nonhealing wounds. There are several kinds of skin substitutes: acellular, cellular, and composite. The acellular skin substitutes can be composed by matrixes of collagen and/or cellulose, epithelial from different species or donated human skin. These substitutes aim to mimic the human extra cellular matrix and thereby provide a surface for migrating host cells. Matrix seeded with fibroblast, autologous or isolated from neonatal foreskin, or keratinocytes, is called cellular skin substitutes. Another cellular substitute is derived from human placenta membranes [Citation107,Citation108]. Placenta membranes contain growth factors, collagen-rich extracellular matrix, and cells including MSCs, neonatal fibroblasts, and epithelial cells. In a multi-center, randomized, single-blinded clinical trial, a cryopreserved placenta membrane was compared to standard wound care. In this study, 62% of the wounds treated with placenta membranes healed during the 12 weeks of the study, whereas only 21.3% of the wounds healed in the control group [Citation109].
In negative pressure wound therapy (NPWT), an airtight open-pore filler fitted to the wound size is placed onto the wound and covered by a transparent bacteria proof, and airtight dressing. The sealed wound is then connected to a vacuum source and a negative pressure is created. The negative pressure at the wound site reduces the size of the wound through contraction; it continuously cleans the wound by removing small debris through suction and reduces levels of proteases through wound fluid removal. Removal of exudate from the wound also permits fewer dressing changes, and the sealed environment provides a moist environment for the wound. The mechanical force induced by NPWT also alters the blood perfusion, promotes angiogenesis and the formation of granulation tissue [Citation110]. The efficiency of NPWT has been evaluated for nonhealing wounds as well as for traumatic wounds, partial thickness burns, skin grafts, and surgical wounds. Evidence for NPWT to promote wound healing is still weak for DFUs and leg ulcers, and for pressure ulcers, partial thickness burns, surgical and skin grafts evidence is lacking [Citation111–Citation115].
Hyperbaric oxygen therapy (HBOT) encompasses inhalation of pure oxygen after entering a special compression chamber, and the treatment aims to increase the oxygen supply to the wound. In a Cochrane review, the authors concluded that HBOT improved short-term but not long-term healing in patients with DFUs [Citation116].
2.8. Challenges and limitations for topical delivery of drugs accelerating wound healing
In the development of topical drugs for wound healing, there are many challenges to considerate. In addition to accelerating wound healing, required qualities for such a treatment include the ability to withstand the proteolytic wound environment to ensure bioavailability of the active substance. In addition, dressing changes disturb the healing process and the optimal treatment should therefore include prolonged release and/or effects. Further, the treatment needs to be cost-efficient to penetrate the market. Combining all of these qualities poses a large challenge. To overcome the proteolytic environment as well as to provide prolonged drug release, the new-generation biological drugs in the form of growth factors and chemokines are incorporated into wound dressings and/or co-administered with protease inhibitors. In addition, cell and gene therapy provide continuous release of, for example, growth factors or chemokines, which also increases the demand on ensuring limited dispersion outside the wound area to minimize potential systemic affects. However, these promising products of recombinant proteins, gene, or cell therapies are today expensive, which limits clinical availability.
3. Conclusion
For academic projects aiming at accelerating wound healing, as well as for projects in discovery phase or in the late preclinical or clinical pipeline, there is a growing trend toward development of biologics, gene therapy, and new technologies for drug delivery. An increasing fraction of treatments in pipeline to be tested in clinical trials are compounds classified or to be classified as medical products. To meet the study objectives and to become a clinical option to treat nonhealing wounds, these approaches are all dependent on increased knowledge of the physiology and pathology of wound healing, as well as the clinical reality, and the factors shifting a healing wound to a nonhealing.
4. Expert opinion
The current lack of efficient treatments for nonhealing wounds is dependent on many factors. First of all, we still do not fully understand why wounds fail to heal but we know that many underlying pathologies increase the risk for acquiring nonhealing wounds. In which phase the healing cascade is halted during development of nonhealing wounds might vary remarkably depending on associated co-morbidities, but also on age or location of the wound. Second, poor translation of promising preclinical results to successful clinical trials meeting the study objectives greatly limits the availability of clinical options. This can be assigned to poor preclinical models of nonhealing wounds, as well as species differences, as preclinical experiments most often are performed in rodents that heal their wounds differently compared to pigs and humans. Third, the proteolytic nature of the wound microenvironment reduces bioavailability of topically delivered peptides and proteins including growth factors and chemokines, which hampers the ability to locally boost signals to stimulate healing [Citation76,Citation78,Citation117,Citation118,Citation75].
The first obstacle should be met by applying a scientific approach to delineate the mechanism of impaired wound healing as well as the mode of action of drug candidates, which in our opinion, are vital for success in clinical trials. The ongoing unraveling of immune cell phenotypes and functions also in the wound healing context opens up new targets as their restorative functions could be exploited. Second, preclinical models have to be designed to match the clinical reality and include comorbidities when evaluating efficacy. Further, other species than rodents should be considered. The third obstacle has to be addressed by creative means to enhance the bioavailability of the active substance by onsite production and/or reduced degradation. Co-administration of enzyme inhibitors or coupling of the active drug substance to different biomaterials are all exciting new avenues that hopefully will lead to efficient and economically feasible treatment strategies to accelerate the healing of nonhealing wounds.
Excitingly, current treatments aiming at accelerating wound healing that are in the pipeline for clinical trials take these concerns into consideration and comprise of biologics, gene therapy, and new concepts for drug delivery. However, in the end, success of new compounds in clinical trials and through approval relies on cost efficiency in manufacturing (cost of goods), market penetration and that the product delivers clinical value to multiple stakeholders through the wound care process.
Article highlights
Development of topical drugs for wound healing is today greatly influenced by the need to reduce spread of antibiotic resistance and the emerging emphasis of health economy
Topical drugs require means to ensure sufficient bioavailability of the active substance within the wound by, for example, onsite production and/or reduced degradation
There is growing trend toward biologics, gene therapy, and novel concepts for drug delivery in drug discovery and in the pipeline for clinical trials
Increased understanding of the pathology of nonhealing wounds as well as the mechanism of action of drug candidates are vital for success in clinical trials
The increasing knowledge of multiple functions of immune cells in wound healing is likely to reveal novel targets.
This box summarizes key points contained in the article.
Declaration of interest
M Philipson and E Vagesjo are co-founders of Ilya Pharma AB with M Phillipson serving as chief strategy officer and E Vagesjo as the CEO. E Öhnstedt and H Lofton Tomenius are employees of Ilya Pharma AB and are supervised by Professor Phillipson in her laboratory at Uppsala University. The authors have no other relevant affiliations or financial involvement with any organization or entity with a financial interest in or financial conflict with the subject matter or materials discussed in the manuscript apart from those disclosed.
Reviewer disclosures
Peer reviewers on this manuscript have no relevant financial or other relationships to disclose.
Acknowledgments
The authors would like to thank Hans Nuctelius at Roland Berger and Henrik Tomenius for helping out with the article’s illustrations.
Additional information
Funding
References
- Funk DJ, Parrillo JE, Kumar A. Sepsis and septic shock: a history. Crit Care Clin. 2009;25:83–101.
- Shah JB. The history of wound care. J Am Coll Certif Wound Spec. 2011;3:65–66.
- Moura Neto A, Zantut-Wittmann DE, Fernandes TD, et al. Risk factors for ulceration and amputation in diabetic foot: study in a cohort of 496 patients. Endocrine. 2013;44:119–124.
- Chan B, Cadarette S, Wodchis W, et al. Cost-of-illness studies in chronic ulcers: a systematic review. J Wound Care. 2017;26:S4–S14.
- Heyer K, Herberger K, Protz K, et al. Epidemiology of chronic wounds in Germany: analysis of statutory health insurance data: epidemiology of chronic wounds in Germany. Wound Repair Regen. 2016;24:434–442.
- Guest JF, Ayoub N, McIlwraith T, et al. Health economic burden that wounds impose on the National Health Service in the UK. BMJ Open. 2015;5:e009283.
- Gottrup F. A specialized wound-healing center concept: importance of a multidisciplinary department structure and surgical treatment facilities in the treatment of chronic wounds. Am J Surg. 2004;187:S38–S43.
- Sen CK, Gordillo GM, Roy S, et al. Human skin wounds: A major and snowballing threat to public health and the economy. Wound Repair Regen. 2009;17:763–771.
- Gurtner GC, Werner S, Barrandon Y, et al. Wound repair and regeneration. Nature. 2008;453:314–321.
- Werner S, Grose R. Regulation of wound healing by growth factors and cytokines. Physiol Rev. 2003;83:835–870.
- Herndon DN, Tompkins RG. Support of the metabolic response to burn injury. Lancet. 2004;363:1895–1902.
- Phillipson M, Kubes P. The neutrophil in vascular inflammation. Nat Med. 2011;17:1381–1390.
- Bianchi ME. DAMPs, PAMPs and alarmins: all we need to know about danger. J Leukoc Biol. 2007;81:1–5.
- Sullivan TP, Eaglstein WH, Davis SC, et al. The pig as a model for human wound healing. Wound Repair Regen. 2001;9:66–76.
- Yager DR, Nwomeh BC. The proteolytic environment of chronic wounds. Wound Repair Regen. 1999;7:433–441.
- Rahim K, Saleha S, Zhu X, et al. Bacterial contribution in chronicity of wounds. Microb Ecol. 2017;73:710–721.
- Demidova-Rice TN, Hamblin MR, Herman IM. Acute and impaired wound healing: pathophysiology and current methods for drug delivery, part 1. Adv Skin Wound Care. 2012;25:304–314.
- Malone M, Bjarnsholt T, McBain AJ, et al. The prevalence of biofilms in chronic wounds: a systematic review and meta-analysis of published data. J Wound Care. 2017;26:20–25.
- Percival SL, Hill KE, Williams DW, et al. A review of the scientific evidence for biofilms in wounds: biofilms in wounds. Wound Repair Regen. 2012;20:647–657.
- Schultz G, Bjarnsholt T, James GA, et al. Consensus guidelines for the identification and treatment of biofilms in chronic nonhealing wounds: guidelines for chronic wound biofilms. Wound Repair Regen. 2017;25:744–757.
- Devalaraja RM, Nanney LB, Qian Q, et al. Delayed wound healing in CXCR2 knockout mice. J Invest Dermatol. 2000;115:234–244.
- Dovi JV, He L-K, DiPietro LA. Accelerated wound closure in neutrophil-depleted mice. J Leukoc Biol. 2003;73:448–455.
- Nishio N, Okawa Y, Sakurai H, et al. Neutrophil depletion delays wound repair in aged mice. Age (Omaha). 2008;30:11–19.
- Christoffersson G, Vagesjo E, Vandooren J, et al. VEGF-A recruits a proangiogenic MMP-9-delivering neutrophil subset that induces angiogenesis in transplanted hypoxic tissue. Blood. 2012;120:4653–4662.
- Massena S, Christoffersson G, Vagesjo E, et al. Identification and characterization of VEGF-A-responsive neutrophils expressing CD49d, VEGFR1, and CXCR4 in mice and humans. Blood. 2015;126:2016–2026.
- Lucas T, Waisman A, Ranjan R, et al. Differential roles of macrophages in diverse phases of skin repair. J Immunol. 2010;184:3964–3977.
- Wynn TA, Vannella KM. Macrophages in tissue repair, regeneration, and fibrosis. Immunity. 2016;44:450–462.
- Boniakowski AE, Kimball AS, Jacobs BN, et al. Macrophage-mediated inflammation in normal and diabetic wound healing. J Immunol. 2017;199:17–24.
- Krzyszczyk P, Schloss R, Palmer A, et al. The role of macrophages in acute and chronic wound healing and interventions to promote pro-wound healing phenotypes. Front Physiol. [Internet]. 2018 [cited 2018 Nov 2];9. DOI:10.3389/fphys.2018.00419/full
- Snyder RJ, Lantis J, Kirsner RS, et al. Macrophages: A review of their role in wound healing and their therapeutic use: review of macrophages in wound healing. Wound Repair Regen. 2016;24:613–629.
- Leung A, Crombleholme TM, Keswani SG. Fetal wound healing: implications for minimal scar formation. Curr Opin Pediatr. 2012;24:371–378.
- Gawronska-Kozak B, Bogacki M, Rim J-S, et al. Scarless skin repair in immunodeficient mice: scarless skin repair in immundeficient mice. Wound Repair Regen. 2006;14:265–276.
- Zlotnik A, Yoshie O. Chemokines: a new classification system and their role in immunity. Immunity. 2000;12:121–127.
- Murdoch C, Finn A. Chemokine receptors and their role in inflammation and infectious diseases. Blood. 2000;95:3032–3043.
- Griffith JW, Sokol CL, Luster AD. Chemokines and chemokine receptors: positioning cells for host defense and immunity. Annu Rev Immunol. 2014;32:659–702.
- Zhang Y, Foudi A, Geay J-F, et al. Intracellular localization and constitutive endocytosis of CXCR4 in human CD34 + hematopoietic progenitor cells. Stem Cells. 2004;22:1015–1029.
- Proost P, Struyf S, Schols D, et al. Processing by CD26/dipeptidyl-peptidase IV reduces the chemotactic and anti-HIV-1 activity of stromal-cell-derived factor-1alpha. FEBS Lett. 1998;432:73–76.
- Lambeir A-M, Proost P, Durinx C, et al. Kinetic investigation of chemokine truncation by CD26/Dipeptidyl Peptidase IV reveals a striking selectivity within the chemokine family. J Biol Chem. 2001;276:29839–29845.
- Mortier A, Gouwy M, Van Damme J, et al. CD26/dipeptidylpeptidase IV-chemokine interactions: double-edged regulation of inflammation and tumor biology. J Leukoc Biol. 2016;99:955–969.
- Ridiandries A, Tan J, Bursill C. The role of chemokines in wound healing. Int J Mol Sci. 2018;19:3217.
- Barrientos S, Stojadinovic O, Golinko MS, et al. PERSPECTIVE ARTICLE: growth factors and cytokines in wound healing: growth factors and cytokines in wound healing. Wound Repair Regen. 2008;16:585–601.
- Park J, Hwang S, Yoon I-S. Advanced growth factor delivery systems in wound management and skin regeneration. Molecules. 2017;22:1259.
- Beer HD, Longaker MT, Werner S. Reduced expression of PDGF and PDGF receptors during impaired wound healing. J Invest Dermatol. 1997;109:132–138.
- Beer HD, Fässler R, Werner S. Glucocorticoid-regulated gene expression during cutaneous wound repair. Vitam Horm. 2000;59:217–239.
- Blakytny R, Jude E. The molecular biology of chronic wounds and delayed healing in diabetes. Diabet Med. 2006;23:594–608.
- Gay D, Kwon O, Zhang Z, et al. Fgf9 from dermal γδ T cells induces hair follicle neogenesis after wounding. Nat Med. 2013;19:916–923.
- Jameson J. A role for skin gamma delta T cells in wound repair. Science. 2002;296:747–749.
- Sharp LL, Jameson JM, Cauvi G, et al. Dendritic epidermal T cells regulate skin homeostasis through local production of insulin-like growth factor 1. Nat Immunol. 2005;6:73–79.
- Brownell I, Guevara E, Bai CB, et al. Nerve-derived sonic hedgehog defines a niche for hair follicle stem cells capable of becoming epidermal stem cells. Cell Stem Cell. 2011;8:552–565.
- Ito M, Liu Y, Yang Z, et al. Stem cells in the hair follicle bulge contribute to wound repair but not to homeostasis of the epidermis. Nat Med. 2005;11:1351–1354.
- Levy V, Lindon C, Zheng Y, et al. Epidermal stem cells arise from the hair follicle after wounding. FASEB J. 2007;21:1358–1366.
- Mascré G, Dekoninck S, Drogat B, et al. Distinct contribution of stem and progenitor cells to epidermal maintenance. Nature. 2012;489:257–262.
- Page ME, Lombard P, Ng F, et al. The epidermis comprises autonomous compartments maintained by distinct stem cell populations. Cell Stem Cell. 2013;13:471–482.
- Tumbar T. Defining the epithelial stem cell niche in skin. Science. 2004;303:359–363.
- Langton AK, Herrick SE, Headon DJ. An extended epidermal response heals cutaneous wounds in the absence of a hair follicle stem cell contribution. J Invest Dermatol. 2008;128:1311–1318.
- Singer AJ, Dagum AB. Current management of acute cutaneous wounds. N Engl J Med. 2008;359:1037–1046.
- International best practice guidelines: wound management in diabetic woot ulcers [Internet]. Wound international; 2013. [cited 2018 Nov 16]. Available from: www.woundinternational.com
- U.S. Food & Drug Administration. PMA approvals [Internet]; 2018 [cited 2018 Nov 19]. Available from: https://www.fda.gov/MedicalDevices/ProductsandMedicalProcedures/DeviceApprovalsandClearances/PMAApprovals/default.htm
- Papanas N, Maltezos E. Becaplermin gel in the treatment of diabetic neuropathic foot ulcers. Clin Interv Aging. 2008;3:233–240.
- Dhivya S, Padma VV, Santhini E. Wound dressings – a review. Biomedicine (Taipei). [Internet]. 2015 [cited 2018 Nov 2];5. Available from: http://www.globalsciencejournals.com/article/10.7603/s40681-015-0022-9
- Simader E, Traxler D, Kasiri MM, et al. Safety and tolerability of topically administered autologous, apoptotic PBMC secretome (APOSEC) in dermal wounds: a randomized Phase 1 trial (MARSYAS I). Sci Rep. 2017;7:6216.
- Gardien KLM, Marck RE, Bloemen MCT, et al. Outcome of burns treated with autologous cultured proliferating epidermal cells: a prospective randomized multicenter intrapatient comparative trial. Cell Transplant. 2016;25:437–448.
- Valentini SR, Nogueira AC, Fenelon VC, et al. Insulin complexation with hydroxypropyl-beta-cyclodextrin: spectroscopic evaluation of molecular inclusion and use of the complex in gel for healing of pressure ulcers. Int J Pharm. 2015;490:229–239.
- Lantis IJC, Gordon I. Clostridial collagenase for the management of diabetic foot ulcers: results of four randomized controlled trials. Wounds Compend Clin Res Pract. 2017;29:297–305.
- Tonaco LAB, Gomes FL, Velasquez-Melendez G, et al. The proteolytic fraction from latex of vasconcellea cundinamarcensis (P1G10) enhances wound healing of diabetic foot ulcers: a double-blind randomized pilot study. Adv Ther. 2018;35:494–502.
- Barrientos S, Brem H, Stojadinovic O, et al. Clinical application of growth factors and cytokines in wound healing: wound management: growth factors & cytokines. Wound Repair Regen. 2014;22:569–578.
- Lobmann R, Ambrosch A, Schultz G, et al. Expression of matrix-metalloproteinases and their inhibitors in the wounds of diabetic and non-diabetic patients. Diabetologia. 2002;45:1011–1016.
- Da Costa RM, Jesus FMR, Aniceto C, et al. Randomized, double-blind, placebo-controlled, dose- ranging study of granulocyte-macrophage colony stimulating factor in patients with chronic venous leg ulcers. Wound Repair Regen. 1999;7:17–25.
- Park KH, Han SH, Hong JP, et al. Topical epidermal growth factor spray for the treatment of chronic diabetic foot ulcers: A phase III multicenter, double-blind, randomized, placebo-controlled trial. Diabetes Res Clin Pract. 2018;142:335–344.
- Yuan L, Minghua C, Feifei D, et al. Study of the use of recombinant human granulocyte-macrophage colony-stimulating factor hydrogel externally to treat residual wounds of extensive deep partial-thickness burn. Burns. 2015;41:1086–1091.
- Mulder G, Tallis AJ, Marshall VT, et al. Treatment of nonhealing diabetic foot ulcers with a platelet-derived growth factor gene-activated matrix (GAM501): results of a Phase 1/2 trial. Wound Repair Regen. 2009;17:772–779.
- Chicharro-Alcántara D, Rubio-Zaragoza M, Damiá-Giménez E, et al. Platelet rich plasma: new insights for cutaneous wound healing management. J Funct Biomater. 2018;9:10.
- Martinez-Zapata MJ, Martí-Carvajal AJ, Solà I, et al. Autologous platelet-rich plasma for treating chronic wounds. Cochrane Wounds Group, editor. Cochrane Database Syst Rev. [Internet]. 2016 [cited 2018 Nov 2]. DOI:10.1002/14651858.CD006899.pub3
- U.S. Food & Drug Administration. 2017 biological device application approvals [Internet]; 2018 [cited 2018 Nov 19]. Available from: https://www.fda.gov/BiologicsBloodVaccines/DevelopmentApprovalProcess/BiologicalApprovalsbyYear/ucm547560.htm
- Wood S, Jayaraman V, Huelsmann EJ, et al. Pro-inflammatory chemokine CCL2 (MCP-1) promotes healing in diabetic wounds by restoring the macrophage response. PloS One. 2014;9:e91574.
- Nakamura Y, Ishikawa H, Kawai K, et al. Enhanced wound healing by topical administration of mesenchymal stem cells transfected with stromal cell-derived factor-1. Biomaterials. 2013;34:9393–9400.
- Miller TJ, Henson K, Aras R, et al. 500. stromal cell-derived factor-1 non-viral DNA therapy accelerates wound healing and decreases scar formation in porcine surgical incisions. Mol Ther. 2013;21:S193.
- Segers VFM, Revin V, Wu W, et al. Protease-resistant stromal cell–derived factor-1 for the treatment of experimental peripheral artery diseaseclinical perspective. Circulation. 2011;123:1306–1315.
- Davis FM, Gallagher K. Time heals all wounds … but wounds heal faster with lactobacillus. Cell Host Microbe. 2018;23:432–434.
- Vågesjö E, Öhnstedt E, Mortier A, et al. Accelerated wound healing in mice by on-site production and delivery of CXCL12 by transformed lactic acid bacteria. Proc Natl Acad Sci. 2018;115:1895–1900.
- Ridiandries A, Bursill C, Tan J. Broad-spectrum inhibition of the CC-chemokine class improves wound healing and wound angiogenesis. Int J Mol Sci. 2017;18(1): 115.
- Kanji S, Das H. Advances of stem cell therapeutics in cutaneous wound healing and regeneration. Mediators Inflamm. 2017;2017:1–14.
- Marfia G, Navone SE, Di Vito C, et al. Mesenchymal stem cells: potential for therapy and treatment of chronic non-healing skin wounds. Organogenesis. 2015;11:183–206.
- Biomendics. TolaSure [Internet]; [ cited 2018 Nov 19]. Available from: http://www.biomendics.com/about-biomendics/
- Gu X, Shen S, Huang C, et al. Effect of activated autologous monocytes/macrophages on wound healing in a rodent model of experimental diabetes. Diabetes Res Clin Pract. 2013;102:53–59.
- Jetten N, Roumans N, Gijbels MJ, et al. Wound administration of M2-polarized macrophages does not improve murine cutaneous healing responses. Brandner JM, editor. PLoS ONE. 2014;9:e102994.
- Zuloff-Shani A, Adunsky A, Even-Zahav A, et al. Hard to heal pressure ulcers (stage III–IV): efficacy of injected activated macrophage suspension (AMS) as compared with standard of care (SOC) treatment controlled trial. Arch Gerontol Geriatr. 2010;51:268–272.
- Mulholland EJ, Dunne N, McCarthy HO. MicroRNA as therapeutic targets for chronic wound healing. Mol Ther Nucleic Acids. 2017;8:46–55.
- Lucas T, Schäfer F, Müller P, et al. Light-inducible antimiR-92a as a therapeutic strategy to promote skin repair in healing-impaired diabetic mice. Nat Commun. 2017;8:15162.
- Gallant-Behm CL, Piper J, Dickinson BA, et al. A synthetic microRNA-92a inhibitor (MRG-110) accelerates angiogenesis and wound healing in diabetic and nondiabetic wounds: MRG-110 accelerates wound healing. Wound Repair Regen. [Internet]. 2018 [cited 2018 Nov 2]. DOI:10.1111/wrr.12660.
- Bowdish D, Davidson D, Hancock R. A re-evaluation of the role of host defence peptides in mammalian immunity. Curr Protein Pept Sci. 2005;6:35–51.
- Scott MG, Davidson DJ, Gold MR, et al. The human antimicrobial peptide LL-37 is a multifunctional modulator of innate immune responses. J Immunol (Baltimore, MD). 1950;2002(169):3883–3891.
- Tomasinsig L, Pizzirani C, Skerlavaj B, et al. The human cathelicidin LL-37 modulates the activities of the P2X 7 receptor in a structure-dependent manner. J Biol Chem. 2008;283:30471–30481.
- Andersson DI, Hughes D, Kubicek-Sutherland JZ. Mechanisms and consequences of bacterial resistance to antimicrobial peptides. Drug Resist Updat. 2016;26:43–57.
- Liu -Y-Y, Wang Y, Walsh TR, et al. Emergence of plasmid-mediated colistin resistance mechanism MCR-1 in animals and human beings in China: a microbiological and molecular biological study. Lancet Infect Dis. 2016;16:161–168.
- Bradshaw JP. Cationic antimicrobial peptides: issues for potential clinical use. BioDrugs. 2003;17:233–240.
- Vaara M. New approaches in peptide antibiotics. Curr Opin Pharmacol. 2009;9:571–576.
- Gomes A, Teixeira C, Ferraz R, et al. Wound-healing peptides for treatment of chronic diabetic foot ulcers and other infected skin injuries. Molecules. 2017;22:1743.
- Agerberth B, Charo J, Werr J, et al. The human antimicrobial and chemotactic peptides LL-37 and alpha-defensins are expressed by specific lymphocyte and monocyte populations. Blood. 2000;96:3086–3093.
- Vermeulen H, van Hattem JM, Storm-Versloot MN, et al. Topical silver for treating infected wounds. Cochrane Wounds Group, editor. Cochrane Database Syst Rev. [Internet]. 2007 [cited 2018 Nov 16]. DOI:10.1002/14651858.CD005486.pub2
- Nherera LM, Trueman P, Roberts CD, et al. A systematic review and meta-analysis of clinical outcomes associated with nanocrystalline silver use compared to alternative silver delivery systems in the management of superficial and deep partial thickness burns. Burns. 2017;43:939–948.
- Ebeling S, Naumann K, Pollok S, et al. From a traditional medicinal plant to a rational drug: understanding the clinically proven wound healing efficacy of birch bark extract. Simon M, editor. PLoS ONE. 2014;9:e86147.
- Barret JP, Podmelle F, Lipový B, et al. Accelerated re-epithelialization of partial-thickness skin wounds by a topical betulin gel: results of a randomized phase III clinical trials program. Burns. 2017;43:1284–1294.
- Gasca-Lozano LE, Lucano-Landeros S, Ruiz-Mercado H, et al. Pirfenidone accelerates wound healing in chronic diabetic foot ulcers: a randomized, double-blind controlled trial. J Diabetes Res. 2017;2017:1–12.
- Janka-Zires M, Almeda-Valdes P, Uribe-Wiechers AC, et al. Topical administration of pirfenidone increases healing of chronic diabetic foot ulcers: a randomized crossover study. J Diabetes Res. 2016;2016:1–7.
- Totsuka Sutto SE, Rodríguez Roldan YI, Cardona Muñoz EG, et al. Efficacy and safety of the combination of isosorbide dinitrate spray and chitosan gel for the treatment of diabetic foot ulcers: A double-blind, randomized, clinical trial. Diab Vasc Dis Res. 2018;15:348–351.
- Nicholas MN, Yeung J. Current status and future of skin substitutes for chronic wound healing. J Cutan Med Surg. 2017;21:23–30.
- Nathoo R, Howe N, Cohen G. Skin substitutes: an overview of the key players in wound management. J Clin Aesthetic Dermatol. 2014;7:44–48.
- Lavery LA, Fulmer J, Shebetka KA, et al. The efficacy and safety of Grafix ® for the treatment of chronic diabetic foot ulcers: results of a multi-centre, controlled, randomised, blinded, clinical trial: efficacy and safety of Grafix ®. Int Wound J. 2014;11:554–560.
- Apelqvist J, Willy C, Fagerdahl A-M, et al. Negative pressure wound therapy - overview, challanges and perspetives. J Wound Care. 2017;26:1–113.
- Liu Z, Dumville JC, Hinchliffe RJ, et al. Negative pressure wound therapy for treating foot wounds in people with diabetes mellitus. Cochrane Wounds Group, editor. Cochrane Database Syst Rev. [Internet]. 2018 [cited 2018 Nov 15]. DOI:10.1002/14651858.CD010318.pub3
- Dumville JC, Land L, Evans D, et al. Negative pressure wound therapy for treating leg ulcers. Cochrane Wounds Group, editor. Cochrane Database Syst Rev. [Internet]. 2015 [cited 2018 Nov 15]. DOI:10.1002/14651858.CD011354.pub2
- Dumville JC, Munson C, Christie J. Negative pressure wound therapy for partial-thickness burns. Cochrane Wounds Group, editor. Cochrane Database Syst Rev. [Internet]. 2014 [cited 2018 Nov 15]. DOI:10.1002/14651858.CD006215.pub4
- Dumville JC, Webster J, Evans D, et al. Negative pressure wound therapy for treating pressure ulcers. Cochrane Wounds Group, editor. Cochrane Database Syst Rev. [Internet]. 2015 [cited 2018 Nov 15]. DOI:10.1002/14651858.CD011334.pub2
- Iheozor-Ejiofor Z, Newton K, Dumville JC, et al. Negative pressure wound therapy for open traumatic wounds. Cochrane Wounds Group, editor. Cochrane Database Syst Rev. [Internet]. 2018 [cited 2018 Nov 15]. DOI:10.1002/14651858.CD012522.pub2
- Kranke P, Bennett MH, Martyn-St James M, et al. Hyperbaric oxygen therapy for chronic wounds. Cochrane Wounds Group, editor. Cochrane Database Syst Rev. [Internet]. 2015 [cited 2018 Nov 16]. DOI:10.1002/14651858.CD004123.pub4
- Demidova-Rice TN, Hamblin MR, Herman IM Acute and Impaired Wound Healing: Pathophysiology and Current Methods for Drug Delivery, Part 2: Role of Growth Factors in Normal and Pathological Wound Healing: Therapeutic Potential and Methods of Delivery.
- Zhu Y, Hoshi R, Chen S, et al. Sustained release of stromal cell derived factor-1 from an antioxidant thermoresponsive hydrogel enhances dermal wound healing in diabetes. J Control Release. 2016;238:114–122.