ABSTRACT
Wood, a fundamental material in construction, confronts durability and weathering challenges, notably UV-induced degradation leading to colour changes. This study investigated a novel treatment using citric acid and urea to enhance the UV stability of wood. The reaction between these compounds generates water-soluble fluorescent species and insoluble particles upon thermal treatment which may provide wood with UV protection. Specimens were treated with two different treatment methods and then exposed to 2016 h of accelerated weathering, during which colour was measured regularly. Citric acid and urea were either pressure impregnated into the wood and thermally reacted in situ during heat treatment or pre-reacted in the absence of wood with subsequent implementation into melamine formaldehyde (MF) and water-based surface coatings. The results showed that water-soluble fluorophore compounds were formed with both treatment methods. Accelerated weathering tests revealed significant colour changes over time, where specimens coated with a mixture of MF and fluorescent particles from the reaction between citric acid and urea, exhibiting the least alteration. The lowest colour change ΔE of 5.9 was observed for specimens coated with a MF-based coating containing 1 wt% of citric acid and urea thermally pre-reacted at a temperature of 180 °C, showcasing potential wood protection applications.
1. Introduction
Wood remains a popular choice in construction despite challenges such as durability, stability, fire resistance, and weathering. Among these, weathering draws attention due to its visual impact. Weathering can be defined as the slow degradation of the wood surface that occurs through the combined effect of sunlight, water, oxygen, temperature, and atmospheric pollution (Feist Citation1983). This degradation process initiates complex chemical reactions, notably triggered by ultraviolet (UV) radiation, leading to the breakdown of polymeric molecules, particularly lignin. It has been shown that lignin is the best absorber of UV light with chromophore functional groups capable of absorbing a broad spectrum of UV light in the wavelength range 250–400 nm (Sadeghifar and Ragauskas Citation2020). The breakdown of lignin leads to the wood losing its original colour, ultimately developing a silver-grey patina on the wood surface. To combat UV degradation, various methods have been employed, including hindered amine light stabilizers (HALS), often based on 2,6-piperidine systems, UV-absorbing compounds like benzophenones and triazines, and nanoparticulate materials such as nanotitania and nanoceria (Jirouš-Rajkovíc and Miklečíc Citation2021). These methods are often applied in conjunction with semi-transparent coatings to preserve the natural aesthetic of wood. Recent research has explored alternative approaches, such as utilising low-cost compounds like citric acid and urea shown by Strauss et al. (Citation2020), who reacted citric acid and urea in a 1:3 molar ratio at temperatures ranging from 100 to 290 °C. At 180 °C and above, a dramatic increase in product yield was observed, indicating a complete reaction with no remaining educts. These compounds can form initial eutectic mixtures and subsequently fluorophore compounds upon heating (). While wood modification with citric acid on its own does not improve the weathering durability of wood (Miklečić and Jirouš-Rajković Citation2011), fluorophore compounds may provide wood with UV protection by, e.g. absorbing detrimental UV radiation and re-emitting it as visible light, dissipating the absorbed energy as heat or lower-energy light, and maintaining photostability to ensure long-term effectiveness, thereby offering low-cost alternatives to compounds such as hindered amine light stabilisers (HALS) (Gijsman Citation2017).
Figure 1. Overview of the thermal reaction between citric acid and urea (Strauss et al. Citation2020).
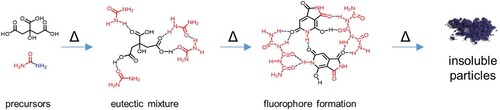
The objective of this study was to produce fluorophore compounds in situ within the cellular structure of wood and generating insoluble particles for application in semi-transparent coatings on wood surfaces to improve the weathering resistance. Initial investigations included examining the fixation of treatments and conducting weathering tests using UV exposure.
2. Materials and methods
Defect-free and straight-grained Scots pine (Pinus sylvestris L.) sapwood specimens with dimensions of 10×93×73 mm (radial x tangential x longitudinal) were prepared. Analytical-grade citric acid (C6H8O7), analytical-grade urea (CH4N2O) (VWR International AB, Stockholm, Sweden) and melamine formaldehyde (MF) powder (Dynea AS, Lillestrøm, Norway) were used.
Three different types of treatments to incorporate thermally-reacted citric acid and urea particles in wood were applied. Namely: (1) impregnation and heat treatment, (2) MF-based surface coating, and (3) water-based surface coating. For each of the treatment types, two chemical concentrations and two reaction temperatures affecting the reaction products were tested. This resulted in 12 different treatment groups, which were extended by four control groups ().
Table 1. Treatment type and process parameter for the preparation of specimens for the accelerated weathering test. MF = melamine-formaldehyde, CU = citric acid and urea, CUL = citric acid and urea pre-reacted at 180 °C, and CUH = citric acid and urea pre-reacted at 220 °C.
Specimens for impregnation and heat treatment were fully submerged in an aqueous solution of 10 wt% or 40 wt% citric acid and urea in a 1:3 molar ratio. The full cell process (Bethell method) was utilized for pressure impregnation. Afterwards, the specimens were dried to 0% moisture content, wrapped in aluminium foil, and placed in an oven for heat treatment at 180 °C or 220 °C for 1 h. 180 °C was chosen based on the results of Strauss et al. (Citation2020) and 220 °C to avoid excessive thermal degradation of the wood.
For the preparation of surface coatings, citric acid and urea were pre-reacted in the absence of wood, potentially undergoing reactions as illustrated in . Two solutions, each containing 50 g of citric acid and urea in a 1:3 molar ratio underwent evaporation at 103 °C to fully remove the water and form eutectic mixtures. Afterwards, the mixtures were placed in an oven at either 180 °C or 220 °C for 8 h, resulting in a mass yield of 45.2% and 36.4% of the initial solid weight, respectively. The resulting particles ((a)) were ground and mixed at concentrations of 1% or 4% () with either a MF solution (50 wt% solid concentration) or water to form the two types of coatings. For coating with MF-based coating, the tangential bark side of each specimen was coated by first applying a thin layer of acetic acid, letting the specimens air-dry at room temperature for 3 h and then applying 200 g/m2 of the MF-based coating, whilst the water-based coating was applied without acetic acid pre-coating. After coating, all specimens were oven-dried at 103 °C for 16 h.
Figure 2. (a) Material from the pre-reaction between citric acid and urea. Under UV-light: water running over the surface of a specimen pressure impregnated and heat-treated (b), treated with MF-based coating (c) and water-based coating (d), both coatings containing pre-reacted citric acid and urea.
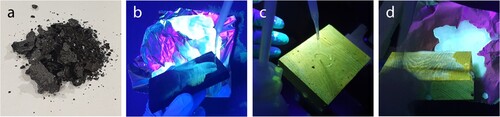
Additional specimens were prepared and exposed to water, to determine if fluorophore compounds were present and if they were fixated by the impregnation and heat-treatment or surface coating. This was done by visual assessment by applying deionized water to the treated surface while the specimens were exposed to UV-light. Additionally, leachates of pressure impregnated, and heat-treaded specimens were collected and analysed with a Hitachi Spectrophotometer U-1500 (Hitachi Ltd. Corporation, Tokyo, Japan).
Accelerated weathering was tested by exposing specimens in a QUV-spray for 2016 h (Q-Lab Europe, Ltd., Bolton, UK) according to the EN 927-6 standard (CEN 2006). 12 exposure cycles were done, each cycle beginning with 24 h condensation followed by 48 subcycles (2.5 h UVA + 0.5 h spray). The colour of the exposed surface was measured at increasing time intervals with a Konica Minolta spectrophotometer CR-410 (Konica Minolta Inc., Osaka, Japan) with the results presented in the CIELCh colour space. Image scans were done after 0, 825 and 2016 h of accelerated weathering exposure.
3. Results and discussion
(b) demonstrates that water-soluble fluorophore compounds were formed in situ during heat-treatment of the impregnated specimens. (d) shows visually similar fluorescence in the specimens coated with the water-based pre-reacted citric acid and urea particles. The same particles were used in the MF-based coating but as seen in (c), no fluorescence was visible under UV-light. This indicates that the water-soluble fluorophore compounds were present in MF-based specimens but fixated in the coating medium. Depending on the process parameter, the water-soluble compounds fluoresced within the blue to green UV spectrum. UV-visible analysis revealed absorption peaks at wavelengths of 325 and 410 nm. These peaks are in a similar range as the UV-absorption spectrum of lignin (250–400 nm), potentially leading to the fluorophore compounds absorbing the UV-light instead of the lignin, providing the wood with UV-resistance.[open-strick]
shows the initial appearance of the treated specimens and the result of the accelerated weathering test after 825 h and 2016 h of exposure.
Figure 3. Surface appearance before and after 825 h and 2016 h of accelerated weathering. C = chroma value at 2016 h and ΔE = colour change from 0 h to 2016 h.
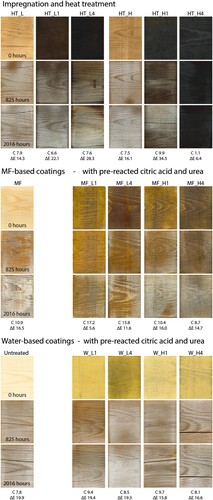
Initially, different treatment methods yielded distinct appearances of the wood surface. Impregnated and heat-treated specimens acquired a deep black tone reminiscent of the treatment solution particles, indicating a parallel reaction within the wood. Compared to the untreated control, specimens treated with MF-based coating displayed a deeper brown hue, while water-based-coated specimens showed a slight green tint and less browning. After 2016 h of exposure, significant changes in surface appearance were observed. The chroma value (C), reflecting surface greyness, was highest in the MF-based coated specimens, indicating richer, more saturated colouration. Water-based-coated and solely heat-treated specimens did not effectively prevent greying, with C-values similar to untreated wood. Although impregnated and heat-treated specimens showed lower initial saturation, their C-values were even lower, likely due to their initial low saturation. The colour change or wood greying over time, quantified by ΔE, varied depending on the initial colour. Solely MF-coated specimens exhibited increased browning and greying of the coating, accompanied by the first signs of coating layer brittleness at 825 h, worsening with further exposure. This can be attributed to crosslink scission of melamine resin which reduced the mechanical flexibility of the coating (English and Spinelli Citation1984, Bauer Citation1987). ΔE was lowest in group MF_L1 and HT_H4. Additionally, no brittleness was observed in the coating of MF_L1 suggesting that the pre-reacted citric acid and urea may have enhanced the UV-stability of MF, while MF prevented leaching of soluble particles. In HT_H4, leaching was not prevented, but dark insoluble particles remained on the surface, unaffected in appearance by weathering exposure. These particles likely acted as a solar screen, protecting the underlying wood from UV-degradation similar to other pigments (Bulian and Graystone Citation2009). When repeating the procedure shown in (b), the group HT_H4 still released fluorescent water-soluble compounds. The images in show uneven, partly spotty discolouration, with more stable colour in the earlywood section of the wood. An improved surface preparation and more controlled application of the surface coating would likely provide more even weathering resistance.
4. Conclusion
The reaction between citric acid and urea is known to produce fluorescent compounds, which upon further thermal treatment, yield insoluble nanoparticulate material. This study has demonstrated the potential of utilising such methods for treating wood within the temperature range of 180 °C to220 °C, either reacting citric acid and urea in the wood or pre-reacting before integration into the wood. Despite observed leaching of fluorescent compounds on pressure impregnated and heat-treated, as well as samples coated with a water-based coating, the incorporation of MF coating, seemed to aid in retaining the fluorophores. The results of the accelerated weathering test showed that the fluorophores not only reduced the greying of the wood surface but they also indicated that the fluorophores increase the UV stability of the MF coating. Incorporating the insoluble polymeric material into coatings may also offer possible routes to protect wood against weathering. Future work aims to further asses the compatibility of citric acid-urea systems with various coatings.
Disclosure statement
No potential conflict of interest was reported by the author(s).
Additional information
Funding
References
- Bauer, D.R., 1987. Photo-physics of melamine-formaldehyde resins: fluorescence and fluorescence lifetimes. Polymer Degradation and Stability, 19 (2), 97–112. doi:10.1016/0141-3910(87)90069-3.
- Bulian, F., and Graystone, J., 2009. Wood coatings: theory and practice. 1st ed. Boston: Elsevier.
- English, A.D., Spinelli, H.J. (1984). Degradation chemistry of primary cross-links in high-solids enamel finishes. In: Labana, S. S., and Dickie, R. A. eds., Characterization of highly cross-linked polymers. Washington, DC: American Chemical Society. 257–269.
- Feist, W.C., 1983. Weathering and protection of wood. Kansas City: American Wood-Preservers Association, 79, pp. 195–205
- Gijsman, P., 2017. A review on the mechanism of action and applicability of Hindered Amine Stabilizers. Polymer Degradation and Stability, 145, 2–10. doi:10.1016/j.polymdegradstab.2017.05.012.
- Jirouš-Rajkovíc, V., and Miklečíc, J., 2021. Enhancing weathering resistance of wood—A review. Polymers, 13, 1980. doi:10.3390/polym13121980.
- Miklečić, J., and Jirouš-Rajković, V., 2011. Accelerated weathering of coated and uncoated beech wood modified with citric acid. Drvna Industrija, 62(4), 277–282. doi:10.5552/drind.2011.1116.
- Sadeghifar, H., and Ragauskas, A., 2020. Lignin as a UV light blocker—A review. Polymers, 12, 1134.
- Strauss, V., et al., 2020. Carbon nanodots revised: the thermal citric acid/urea reaction. Chemical Science, 11, 8256.