Abstract
Bromination of an alkene is a typical addition reaction covered in an introductory organic chemistry course and laboratory. In this undergraduate organic chemistry laboratory exercise, students studied the bromination of a 4,5-dimethyl-1,4-cyclohexadiene-1,2-dicarboxylic acid. The reaction has an unexpected outcome as bromination yields the aromatic product, 4,5-dimethylphthalic acid. Green chemistry modification involves application of a “bromide/peroxide reaction” with NaBr/H2O2 in an acidic medium as an in situ source of bromine. The experiment was carried out as a two-day exercise and the students integrated molecular modeling, interpretation of mass spectra, knowledge of stability of organic compounds, and knowledge of the reaction mechanisms of addition and elimination to explain the experimental outcome.
Introduction
Bromination of an alkene is an addition reaction covered in an introductory organic chemistry course Citation1–3. Study of bromination reaction introduces physical organic chemistry concepts and reaction mechanisms to the students Citation1–6. Bromination is also suitable as an undergraduate laboratory experiment as it not only illustrates the material covered in the class, but is also a fast reaction that can be completed in a single laboratory period. Furthermore, it usually gives clean stable products in a high yield. Drawbacks of a traditional bromination of an alkene experiment include the use of a highly toxic and corrosive liquid bromine, usually in a chlorinated solvent. Finally, one may consider that a predictable reaction outcome may be a drawback as it may make the experiment a trivial exercise. While we want organic chemistry laboratories to illustrate concepts covered in the class, it is even better if laboratory exercises further students’ understanding as well as help develop their analytical and critical thinking skills. Some of these concerns have been addressed by Doxsee and Hurchison Citation7 Citation8. They have developed a procedure for bromination of trans-stilbene that utilizes a less hazardous HBr/H2O2 as a source of bromine and the students were expected to elucidate the stereochemical outcome of the reaction.
In this laboratory exercise, students studied the bromination of a 4,5-dimethyl-1,4-cyclohexadiene-1,2-dicarboxylic acid (1), which they prepared in the previous laboratory session. The reaction has an unexpected outcome in that the bromine addition is followed by an elimination of hydrogen bromide to give the aromatic product, 4,5-dimethylphthalic acid (2). Thus, the experiment is suitable as an organic chemistry laboratory exercise after both the addition and the elimination reactions have been covered in the lectures. This is a green chemistry experiment with HCl/NaBr/H2O2 as a source of bromine Citation7–10. The experiment was carried out as a two-day exercise. On the first day, students completed molecular modeling of their proposed product and related compounds and set up a Diels–Alder reaction for preparation of 4,5-dimethyl-1,4-cyclohexadiene-1,2-dicarboxylic acid (1). On the second day, they performed the actual bromination reaction, isolated and identified the reaction product.
Results and discussion
Molecular modeling exercise
Most of the first day of the two-day experiment was spent on a molecular modeling exercise. The students also set up a reaction for preparation of the starting material, 4,5-dimethyl-1,4-cyclohexadiene-1,2-dicarboxylic acid (1), which they isolated the following day. Students were supposed to come prepared and, as a part of their preparation, they were expected to predict the reaction product. Most students assumed that the exercise was going to be about the chemoselectivity of bromine addition to the two double bonds and predicted that the bromine was going to add to the dimethyl substituted double bond (). Effects of substituents on reactivity were covered extensively in the class and students proposed that, in an electrophilic addition reaction, the double bond with two electron donating groups (EDGs) will be more reactive compared to a double bond with a two electron withdrawing groups (EWGs).
Figure 1. In an electrophilic addition a double bond with two electron donating groups is more reactive than a double bond with two electron withdrawing groups.
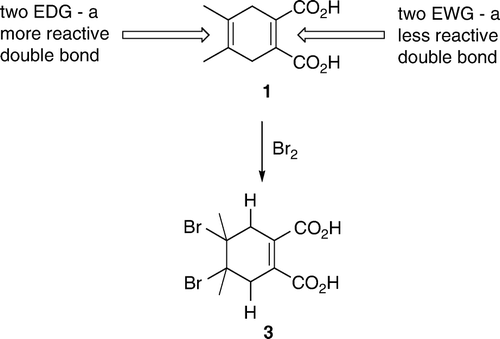
As the first part of the exercise, students used ordinary molecular model sets to make models of their proposed product, 4,5-dibromo-4,5-dimethyl-1-cycloxehene-1,2-dicarboxylic acid (3). It came as no surprise to us that, after examining their models, students came to a conclusion that the conformation with two bromine substituents in equatorial positions was the more stable one. At this point, we explained to the students that molecular model sets show only bond lengths and bond angles relatively accurately, but do not represent steric bulk of the atoms. We asked them to draw conformations represented by the molecular models into their notebooks and suggested that they move on to a computer exercise and then compare the results. Students used ChemSketch to draw structures and 3D Viewer for molecular modeling of the starting material 1, their proposed reaction product 3 and 4,5-dimethylphthalic acid (2). Now, with the aid of computer generated space-filling representations, students found that the conformation of their proposed reaction product 3 with diaxial bromines was likely to be more stable than the diequatorial bromine atoms. In this case, due to the presence of the remaining double bond there are no 1,3-diaxial interactions with bromine substituents. Furthermore, in a diequatorial conformation, the two bulky bromine atoms are closer together. At the end of the exercise, we pointed out to the students that, strictly speaking, those were not axial and equatorial substituents, but rather pseudoaxial and pseudoequatorial, as the ring was cyclohexene not cyclohexane. At the end of the exercise, after students concluded that the diaxial conformation was more stable, they were also told that, if one was to take electrostatic effects into account, the diaxial conformation with the two carbon–bromine dipoles canceling each other, should be even more stable than diequatorial. Students were already used to unexpected results in organic chemistry laboratory exercises. When they realized that the diaxial conformation was the more stable one, there was a sigh of relief as they assumed that this was the unexpected result for this exercise.
Bromination reaction
The bromination procedure was a modification of the experiment developed by Doxsee and Hutchison Citation7 Citation8 with sodium bromide as a source of bromine. In the course of the development of this exercise, we examined HBr, LiBr, NaBr, and MgBr2 as bromide sources in the bromide/peroxide reaction. HBr reacted at a relatively high rate. However, the reaction product was impure. The two bromide salts, LiBr and MgBr2, reacted at a somewhat slower rate and gave the product distribution similar to that of HBr. Interestingly, the use of sodium bromide resulted in the formation of a pure product. However, the reaction was too slow (~4–5 h) to be completed in a single laboratory period. We found that by performing the reaction in 0.1 M HCl the rate increased and the reaction was completed in ~1 h while still yielding a relatively pure product.
Our laboratory sections consist of 12–18 students. Students worked in groups of two and each group was assigned a bromide/peroxide reaction under a different set of conditions. Thus, some groups were assigned a reaction with a catalytic amount of NaBr (1 equivalent), some a stoichiometric amount of NaBr (2 equivalents), and some an excess of NaBr (4 equivalents). At the end of the laboratory period, each group provided a prepared sample for gas chromatography–mass spectrometry (GC–MS) analysis. The analyses were carried out overnight and the students were able to collect the results on the following day. Each student received a package containing the results obtained by the entire section and was expected to write a laboratory report discussing all of the results obtained by the entire section, not just his/her own. Experimental details and student procedures are available as Supplemental Materials.
Although the experimental outcome came as a surprise to the students, they had no difficulty identifying the reaction product from its mass spectrum. They were also able to propose a reasonable reaction mechanism. Most of the students proposed an addition–elimination mechanism shown in . Students did not carry out any mechanistic studies and the proposed mechanism was based on a consideration of electronic effects and known reactivity of the alkenes. Molecular modeling analysis indicated that the conformation of 3 with diaxial bromines was more stable than the diequatorial conformation. Thus, assuming that the dibromide 3 was a reaction intermediate, both the two bromine and the two neighboring hydrogen atoms were already in the correct, antiperiplanar, geometry for elimination. Some students posed a question: “How does the intermediate ‘know’ that the elimination will give the more stable, aromatic, product?” Explanation given to the students involved antibonding C–Br orbitals, and weakening of the allylic C–H bonds by electron delocalization through hyperconjugation Citation15. Delocalization of electrons from the two allylic C–H bonds into the antibonding C–Br orbitals results in weakening of C–H and C–Br bonds. The two delocalized electron pairs, together with the existing C–C π electrons, form a ring of six-delocalized electrons (). Weakening of the C–H and C–Br bonds makes elimination a kinetically favored process, while formation of an aromatic product makes it thermodynamically favored.
Figure 3. (a) Role of anti-bonding orbitals (shown in grey) in the elimination. Bonds that are broken are shown in bold. (b) Electron delocalization from C–H into anti-bonding C–Br bonds results in formation of an aromatic ring of six π-electrons (hydrogen and bromine atoms as well as the substituents have been omitted for clarity).
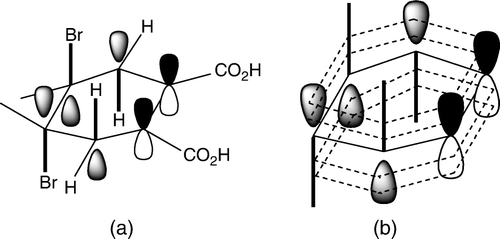
We did not shield the reaction mixture from light and, as the positions 3 and 6 on the cyclohexadiene ring are activated by the two double bonds, there was a possibility of a free radical substitution. Indeed, several students proposed an alternative substitution–elimination mechanism that involved an allylic substitution followed by elimination (). Some additional alternative mechanisms are shown in . All of the proposed mechanisms are based on an assumption that 4,5-double bond was the more reactive one and that it underwent the initial reaction with bromine.
Finally, while the reflux part of bromide/peroxide experiment was in progress, some students carried out calculations of the limiting reagent and realized that, in some experiments, they were using only a catalytic amount of NaBr. They were told that there was no need to check their calculations, that they should calculate reaction yield with respect to the starting diene and that they should wait for the results of the reaction and then consider all the information when explaining the reaction outcome.
Most of the students isolated the crude reaction product in 50–70% yields. The yields were slightly lower (56–60%) when NaBr was used in a catalytic amount (1 equivalent) and somewhat better (~70%) when NaBr was used in either a stoichiometric amount or an excess (2–4 equivalents). Some students performed the reaction on 0.2 g (1.0 mmol), while others were assigned a reaction on 0.4 g (2.0 mmol) of 4,5-dimethyl-1,4-cyclohexadiene-1,2-dicarboxylic acid (1). All the groups obtained similar results regardless of the reaction scale. The main reason for modest yields was a high solubility of 4,5-dimethylphthalic acid (2) in water. Its solubility is even higher in warm water. Thus, if students extracted warm aqueous solution, yields were lower. The product was a yellowish liquid that solidified upon standing. Upon standing for several days the product may get dark brown. However, if purified by rinsing it with hexanes, the reaction product was a pale yellow solid that did not change color upon standing and gave a satisfactory 1H NMR. Some of the students, who isolated the product in “80–98% yields,” actually had a very wet product that did not completely solidify. GC–MS was by far the best method to identify the reaction product. Mass spectra of both the starting material and the reaction product give the M-18 peak of the corresponding anhydrides Citation16. Analysis of either the ethyl acetate extract or the isolated crude product dissolved in ethanol showed only a single peak due to 3,4-dimethylphthalic anhydride (). The two carboxylic groups are in a close proximity to each other and, most likely, the corresponding anhydride was generated in the GC-injector (). The students were given the mass spectrum of the starting material () and were told that they should take the formation of the anhydride into account when calculating the molecular mass of the product. When given the mass spectrum of their product, students were understandably confused and questioned whether it was the correct one. When told that it was indeed the correct spectrum and that the M-18 ion was there, the students quickly realized what the product was and understood why they also modeled 4,5-dimethylphthalic acid (2). At the time, it appeared to them only as a trivial, time-filling, exercise that illustrated higher stability of aromatic compounds compared to corresponding non-aromatic molecules. Some students found it difficult to explain the catalytic role of NaBr. In a post-experiment discussion, students were guided through the process and we ended up with a catalytic cycle represented in (b) that students easily understood. Interestingly, students had difficulty understanding (c), which represents the same catalytic cycle the way it is common in scientific journals.
Figure 7. Both the starting material and the product give mass spectra of the corresponding anhydrides.
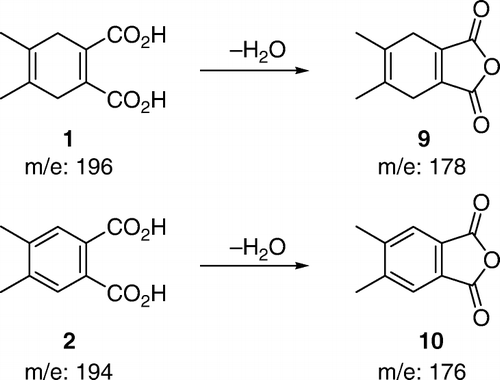
A proton NMR is another method to identify the reaction product (). However, it had to be taken in DMSO-d 6 and it was rather difficult to obtain a good spectrum. The product was very difficult to purify and attempts at recrystallization and column chromatography failed. We were able to get a relatively good proton NMR after thoroughly drying ethyl acetate extract (two dryings over anhydrous magnesium sulfate and a filtration between the dryings), evaporation of ethyl acetate and trituration of the product with hexanes. In the course of an ordinary laboratory session, students may not have enough time to accomplish this. In a view of difficulty of obtaining a pure sample for NMR analysis, students are more likely to obtain a spectrum of an impure compound and misinterpret it. On the other hand, GC–MS, combined with the chemical history of the compound, clearly indicated what the product was.
Analysis by TLC clearly shows that the starting material has been consumed and a new compound formed. However, it does not provide any indication of identity of the product. Beilstein test in conjunction with TLC may be a good idea. However, for a negative Beilstein test the product must be very pure and contain no trace of halogen, which is relatively difficult to accomplish in this experiment.
This reaction sequence also worked very well on the corresponding dimethyl ester. In fact, both the dimethyl 4,5-dimethyl-1,4-cyclohexadien-1,2-dicarboxylate and dimethyl 4,5-dimethylphthalate were easier to purify. However, esters of phthalic acid are potential endocrine disruptors, while phthalic acid itself is a relatively harmless compound Citation17.
Green chemistry aspects of the laboratory exercise
Both the preparation of the starting 4,5-dimethyl-1,4-cyclohexadiene-1,2-dicarboxylic acid (1) and the actual addition–elimination exercise are green chemistry processes. The starting material was prepared in another green chemistry experiment – reaction of 2,3-dimethyl-1,3-butadiene and acetylenedicarboxylic acid in water Citation14.
In the laboratory session following this experiment, we discussed both the reaction mechanism and the green chemistry aspects of this exercise. In their reports students were expected to evaluate how environmentally friendly the process was. In particular, we hoped that they would understand that there is virtually no “perfect” green chemistry reaction and that a design of it will always involve some trade offs. Students were asked to evaluate different procedures with respect to the 12 principles of green chemistry Citation18.
Compared to a traditional bromination experiment, the green chemistry aspects of the described procedure include: prevention of waste (the only waste was a diluted acidic aqueous solution and a small amount of hexanes used in rinsing of the reaction product), design of a less hazardous chemical syntheses (use of highly hazardous bromine had been avoided), use of catalytic reagents (the process was catalytic with respect to hydrogen peroxide) and use of safer solvents (water is the solvent).
Experimental
Molecular modeling was carried out with ACD/ChemSketch and ACD/3D Viewer. The programs are available for a free download Citation11. If available, students can use Spartan Citation12 or Cambridge Software Chem3D Citation13 programs. The starting material, 4,5-dimethyl-1,4-cyclohexadiene-1,2-dicarboxylic acid Citation1–3, was prepared in a Diels–Alder reaction of 2,3-dimethyl-1,3-butadiene and acetylenedicarboxylic acid Citation14. 2,3-Dimethyl-1,3-butadiene has a tendency to dimerize and one should use an excess (1.5 equivalents) of it. GC–MS analyses were performed by means of an Agilent 6890N Gas Chromatograph equipped with an HP-5MS 30 m×0.25 mm column and an Agilent 5973N MSD. 1H NMR spectra were recorded on a Varian 400 MHz spectrometer in DMSO-d 6 solutions.
Hazards
2,3-Dimethyl-1,3-butadiene, ethyl acetate, and ethanol are flammable. Concentrated HCl could cause burns.
Preparation of 4,5-dimethyl-1,4-cyclohexadiene-1,2-dicarboxylic acid
Acetylenedicarboxylic acid (1.14 g) was dissolved in 3.0 mL of water, 2,3-dimethyl-1,3-butadiene (1.23 g) was added and the solution was stirred at a room temperature until the solid product appeared (48–72 h). One can leave it stirring until the next laboratory period. The product was filtered, rinsed with a small amount of cold water and allowed to dry in air. The resulting solid was crushed into a fine powder, rinsed with hexanes and allowed to dry in air. The product was suitable for use in the next step. 1H NMR (DMSO-d 6): δ 2.81 (s, 4H, CH2), 1.62 (s, 6H, CH3).
Bromination of 4,5-dimethyl-1,4-cyclohexadiene-1,2-dicarboxylic acid
A mixture of 4,5-dimethyl-1,4-cyclohexadiene-1,2-dicarboxylic acid (0.200 g), 2.0 mL of 0.1 M aqueous solution of HCl, and sodium bromide (0.103 g) was placed in a 25 mL round bottom flask equipped with a stirring bar and a reflux condenser. The mixture was heated and, at the boiling point, 3.0 mL of 30% aqueous hydrogen peroxide was added through the top of the condenser. Reflux was continued for 60 minutes. The solution was allowed to cool, transferred to a 30 mL separatory funnel and extracted three times with ethyl acetate (3.0 mL of ethyl acetate each time). Ethyl acetate extracts were combined and dried with anhydrous magnesium sulfate. The extract was filtered and the solvent was removed on a rotary evaporator. A small amount of the product was dissolved in ethanol and analyzed by GC–MS. 1H NMR (DMSO-d 6): δ 7.42 (s, 2H, aromatic H), 2.26 (s, 6H, CH3).
Conclusion
Students liked the experiment as the unexpected outcomes were both interesting and presented a challenge they were able to relatively easily meet. While the higher stability of the diaxial conformer and the aromatic reaction product were not what they expected, they had no difficulty understanding and explaining the results of molecular modeling, outcome of the experiment and the reaction mechanism. In the course of a two-day exercise, students had to integrate molecular modeling, interpretation of mass spectra, their knowledge of stability of organic compounds and a detailed knowledge of the mechanisms of addition and elimination reactions to explain the experimental outcome. Finally, students were expected to compare and discuss green chemistry aspects of the experiment and a traditional bromination procedure.
Supplementary Data
Download Zip (2.3 MB)Acknowledgements
We thank Wilkes Honors College of Florida Atlantic University CHM 2205L students who tested this laboratory exercise and Nicole Windmon from Department of Chemistry, Florida Atlantic University, for recording 1H NMR spectra. A partial financial support from the Wilkes Honors College of Florida Atlantic University is gratefully acknowledged.
References
- Bruice , P.J. 2007 . Organic Chemistry , 5th ed , 175 – 178 . Upper Saddle River, NJ : Pearson Education .
- Wade , L.G. Jr. 2006 . Organic Chemistry , 6th edn , 342 – 345 . Upper Saddle River, NJ : Pearson Education .
- Jones , M. Jr. 2005 . Organic Chemistry , 3rd edn , 442 – 449 . New York, NY : W.W. Norton .
- Bosch , E. Chem. Educ . [Online] 2005 , 10 , 333 336 . http://chemeducotor.org, DOI: 10.1333/s00897050949a .
- Amburgey-Peters , J.C. ; Haynes , L.W. J. Chem. Educ . 2005 , 82 , 1051 1052 .
- McGowens , S.I. ; Silversmith , E.F. J. Chem. Educ . 1998 , 75 , 1293 1294 .
- McKenzie , L.C. ; Huffman , L.M. ; Hutchison , J.E. J. Chem. Educ . 2005 , 82 , 306 310 .
- Doxsee , K.M. ; Hutchison , J.E. Green Organic Chemistry ; Brooks/Cole : Florence, KY , 2004 ; 120 128 .
- Rothenberg , G. ; Clark , J.H. Green Chem . 2000 , 2 , 248 251 .
- Ho , T-L. ; Gupta , B.G.B. ; Olah , G.A. Synthesis 1977 , 10 , 676 677 .
- ACD ChemSketch web site http://www.acdlabs.com/download/(accessed May 26, 2009) .
- Spartan Software web site http://www.wavefun.com/products/spartan.html (accessed May 26, 2009) .
- Cambridge Software web site http://www.cambridgesoft.com/software/ChemDraw/(accessed May 26, 2009) .
- Windmon , N. ; Dragojlovic , V. Green Chem. Lett. Rev . 2008 , 1 , 155 163 .
- (a) Pophristic , V. ; Goodman , L. Nature 2001 , 411 , 565 – 568 ; (b) Pophristic , V. ; Goodman , L. J. Phys. Chem. A 2002 , 106 , 1642 – 1646 .
- Karasek , F.W. ; Kim , S.H. Anal. Chem . 1975 , 47 , 1166 1168 .
- Ema , M. ; Miyawaki , E. ; Harazono , A. ; Kawashima , K. Toxicol. Lett . 1997 , 93 , 109 115 .
- Anastas , P.T. and Warner , J.C. 1998 . Green Chemistry: Theory and Practice , Oxford : Oxford University Press .