Abstract
Ceric ammonium nitrate in polyethylene glycol has been used as a sustainable, non-volatile, and ecofriendly catalytic medium for the green synthesis of 2,5-disubstituted 1,3,4-oxadiazoles. This protocol is effective toward various substrates having different functionalities. The easy recyclability of the reaction medium makes the reaction economically and potentially viable for commercial applications.
Introduction
1,3,4-Oxadiazoles are a class of heterocycles which have attracted significant interest in medicinal chemistry and they have a wide range of pharmaceutical and biological activities such as anti-inflammatory Citation1, anticonvulsant Citation2, and antibacterial activities Citation3. They have also shown antimitotic Citation4, antifungal Citation5, and muscle relaxant activity Citation6. In view of the great medical significance, a number of synthetic routes have been developed for 1,3,4-oxadiazoles Citation7–9. Many of these methods suffered from the drawbacks of strong acidic conditions, expensive reagents, long reaction times, harsh experimental conditions, and tedious work up procedures that generate large amounts of toxic waste. Hence the development of a synthetic protocol that is nature friendly, simple, efficient, and cost effective remains an ever challenging objective.
Recently, cerium (IV) ammonium nitrate has emerged as an important reagent for the construction of carbon–carbon and carbon–heteroatom bonds Citation10 Citation11. In addition, many advantages such as excellent solubility in water, inexpensiveness, ecofriendly nature, easy handling, high reactivity, fast conversions, and convenient work up procedures make ceric ammonium nitrate (CAN) a potent catalyst in organic synthesis. Besides this, CAN is able to catalyze various organic transformations not only based on its electron transfer capacity, but also with its Lewis acidic property Citation12–14.
The conventional synthetic procedures invariably use organic solvents as media to provide a homogeneous phase that allows molecular interactions effectively and bring the reaction to completion. Regulatory pressure is increasingly focusing on the use, manufacture, and disposal of organic solvents and thus the development of non-hazardous alternatives (one of the several goals of green chemistry and engineering) is vitally important for the continued and sustainable development of the chemical enterprise. The use of water as a solvent is probably the most desirable approach but this is often not possible due to the hydrophobic nature of reactants. Therefore it was thought worthwhile to use polyethylene glycol (PEG) as the reaction media, as it may stand in comparison to other currently favored systems such as supercritical CO2, near critical water, ionic liquids, and fluorous-based systems. Unlike these “neoteric solvents” whose environmental safety is still debated, complete toxicity profiles are available for a range of PEG molecular weights and indeed many are already approved for internal consumption by US FDA Citation15.
The versatility of CAN and the green nature of PEG encouraged us to couple them together and study their utility for the synthesis of 2,5-disubstituted 1,3,4-oxadiazoles. In continuation of our studies on developing cheap and environmentally benign methodologies for organic reactions Citation16 Citation17, we describe the use of a simple and widely available polymer, PEG as a non-toxic, inexpensive, and non-ionic liquid solvent of low volatility with CAN for the synthesis of 2,5-disubstituted 1,3,4-oxadiazoles (3) ().
Results and discussions
In the beginning, efforts were made toward the catalytic evaluation of CAN toward the synthesis of oxadiazoles. The reaction using 1 equiv. of benzhydrazide (1) and 1 equiv. of carboxylic acid (2a) was performed in traditional organic solvent (EtOH, ). The reaction mixture was stirred for 32 h at 80°C to obtain 48% of oxadiazole 3a. The same reaction was then carried out using PEG as the reaction medium under similar conditions. Surprisingly, a significant improvement was observed and the yield of 3a dramatically increased to 83% after stirring the reaction mixture at 80°C for only 12 h. PEG as a reaction medium markedly catalyzed the reaction ().
Figure 1. Synthesis of 2,5-disubstituted oxadiazoles in various solvents.a
aReaction conditions: carboxylic acid (1 mmol), benzhydrazide (1 mmol); temperature 80°C; solvent.
bIsolated and unoptimized yields.
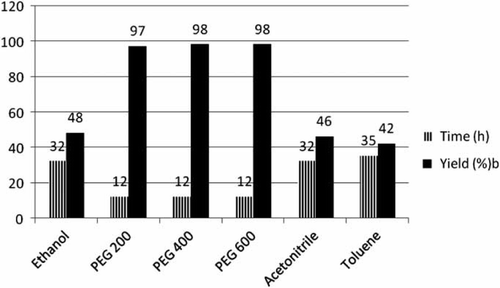
To further improve the yield and to optimize the reaction conditions, the same reaction was carried out in the presence of 2 mol% of CAN under similar conditions. A tremendous improvement was observed and the yield of 3a was dramatically increased up to 94% after stirring the reaction mixture at 80°C only for 7 h. With this optimistic result in hand, we further investigated the best reaction conditions by using different amounts of CAN. An increase in the quantity of CAN from 2 to 5 mol% not only decreased the reaction time from 7 to 5 h, but also increased the product yield slightly from 94 to 98%. This showed that the catalyst concentration plays a major role in optimization of the product yield ().
Figure 2. Catalytic activity evaluation of CAN for the synthesis of 2,5-disubstituted oxadiazoles.a
aReaction conditions: carboxylic acid (1 mmol), benzhydrazide (1 mmol); temperature 80°C; PEG 400.
bIsolated and unoptimized yields.
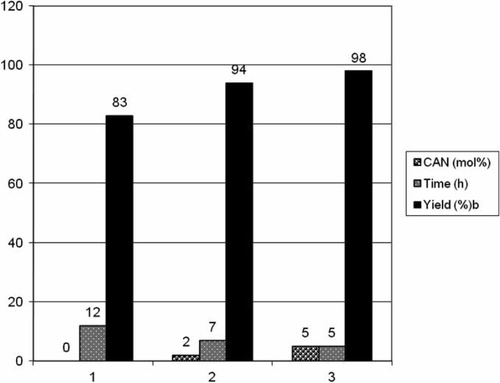
Various other Lewis acids were also tried for the reaction between 1 equiv. of benzhydrazide (1) and 1 equiv. of carboxylic acid (2a), and it was found that CAN acted as the best catalyst for the synthesis of 2,5-disubstituted oxadiazoles ().
Table 1. Screening of various Lewis acids for the synthesis of 2,5-disubstituted oxadiazoles.a
In order to prove that the use of PEG as a solvent is also practical, it must be conveniently recycled with minimal loss and decomposition. Since PEG is immiscible with solvent ether, the desired product may be extracted with it and the retained PEG phase may be reused. The reaction proceeded cleanly with consistent results for four runs, although a weight loss of approximately 5% of PEG was observed from cycle to cycle due to mechanical loss ().
Figure 3. Recycling yields.
Note: Reaction conditions: carboxylic acid (1 mmol), benzhydrazide (1 mmol), CAN (5 mol%); solvent PEG 400; temperature 80°C.
aIsolated and unoptimized yields.
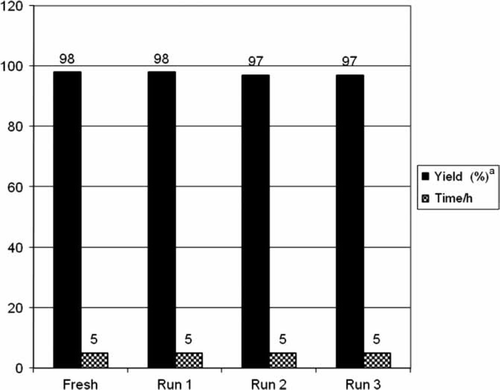
Based on the above observations, we conducted the same reactions using various carboxylic acids (2a–j) under similar conditions and as expected satisfactory results were observed ().
Table 2. Synthesis of various 2,5-disubstituted oxadiazoles.a
Experimental
All chemicals were purchased from Sigma–Aldrich and Lancaster, and were used as such. All reactions and purity of 2,5-disubstituted 1,3,4-oxadiazoles (3) were monitored by thin layer chromatography (TLC) using aluminium plates coated with silica gel (Merck) using 15% ethyl acetate, 5% methanol, and 80% petroleum ether as an eluent. The isolated products were further purified by column chromatography using silica gel (Sigma–Aldrich 24, 217–9,70, 35–70, mesh 40 Ao surface area 675 m2/g) and purified product were recrystallized. IR spectra was recorded on a Perkin–Elmer FTIR-1710 spectrophotometer using Nujol film. 1H NMR spectra was recorded on a Bruker Avance Spectrospin 300 (300 MHz) using TMS as an internal standard and chemical shift, in Δ. GC/MS mass spectra was recorded on a Waters LCT Micromass. The temperature of the reaction mixture was measured through a non-contact infrared thermometer (AZ, Mini Gun type, Model 8868).
General procedure for the synthesis of 2,5-disubstituted 1,3,4-oxadiazoles
In a 50 ml round bottom flask, benzhydrazide (1 mmol) and carboxylic acid (1 mmol) in PEG 400 (0.2 ml) were mixed and stirred at 80°C. To this, CAN was added. The progress of reaction mixture was monitored by TLC. After completion of reaction, the reaction mixture was cooled in a dry ice-acetone bath to precipitate the PEG 400, and extracted with ether (PEG being insoluble in ether). Ether layer was decanted, dried, and concentrated under reduced pressure. The product, though seen as a single compound by TLC, was subjected to further purification by silica gel column chromatography using 15% ethyl acetate, 5% methanol, and 80% petroleum ether as an eluent to yield the product 3a–j. The compounds were recrystallized using ethanol and chloroform by the layering technique. The recovered PEG 400 can be reused for consecutive runs. The structures of all the products were unambiguously established on the basis of their spectral analysis (IR, 1H NMR, and GC/MS mass spectral data). All the products are known compounds.
Spectral data of synthesized compounds
2,5-Diphenyl-1,3,4-oxadiazole (3a, C14H10N2O)
ν max (KBr) cm−1 3052, 2948, 1620, 1560, 1510, 1440, 1270, 1080, 1030, 710, 670; 1H NMR (DMSO-d 6, TMS): ΔH 7.52–7.57 (6H, m), 8.14–8.26 (4H, m); m/z (GC/MS, HRMS): 222.0797 (M+, C14H10N2O required 222.0793). Analysis calculated for C14H10N2O: C, 75.66; H, 4.54; N, 12.60. Found: C, 75.64; H, 4.53; N, 12.62.
2-(2-Aminophenyl)-5-phenyl-1,3,4-oxadiazole (3b, C14H11N3O)
ν max (KBr) cm−1 3450, 3350, 1630, 1590, 1450, 1272, 1120, 1045, 712, 678; 1H NMR (DMSO-d 6, TMS): ΔH 6.8–8.16 (9H, m, Ar), 11.2(2H, s, -NH2); m/z (GC/MS, HRMS): 237.0908 (M+, C14H11N3O required 237.0902). Analysis calculated for C14H11N3O: C, 70.87; H, 4.67; N, 17.71. Found: C, 70.84; H, 4.66; N, 17.73.
2-(2-Hydroxyphenyl)-5-phenyl-1,3,4-oxadiazole (3c, C14H10N2O2)
ν max (KBr) cm−1 3196, 2924, 1630, 1578, 1487, 1308, 1286, 1161, 1072, 1029, 708, 690; 1H NMR (DMSO-d 6, TMS): ΔH 7.12–8.18 (9H, m, Ar), 10.18 (1H, s, OH); m/z (GC/MS, HRMS): 238.0747 (M+, C14H10N2O2 required 238.0742). Analysis calculated for C14H10N2O2: C, 70.58; H, 4.23; N, 11.76. Found: C, 70.59; H, 4.25; N, 11.78.
2-(2-Methylphenyl)-5-phenyl-1,3,4-oxadiazole (3d, C15H12N2O)
ν max (KBr) cm−1 3112, 2870, 1630, 1600, 1560, 1500, 1470, 1420, 1320, 1290, 1200, 1114, 1030, 840, 732, 700; 1H NMR (DMSO-d 6, TMS): ΔH 2.44 (3H, s, CH3), 7.32–7.56 (9H, m, Ar); m/z (GC/MS, HRMS): 236.0952 (M+, C15H12N2O required 236.0950). Analysis calculated for C15H12N2O: C, 76.25; H, 5.12; N, 11.86. Found: C, 76.22; H, 5.14; N, 11.87.
2-(2-Chlorophenyl)-5-phenyl-1,3,4-oxadiazole (3e, C14H9ClN2O)
ν max (KBr) cm−1 3100, 1620, 1570, 1510, 1422, 1321, 1280, 1100, 1082, 1030, 980, 840; 1H NMR (DMSO-d 6, TMS): ΔH 7.12–7.57 (9H, m, Ar); m/z (GC/MS, HRMS): 256.0408 (M+, C14H9ClN2O required 256.0403). Analysis calculated for C14H9ClN2O: C, 65.51; H, 3.53; N, 10.91. Found: C, 65.53; H, 3.51; N, 10.92.
2-(4-Methoxyphenyl)-5-phenyl-1,3,4-oxadiazole (3f, C15H12N2O2)
Mp ν max (KBr) cm−1 3201, 1631, 1534, 1488, 1377, 1290, 1119, 1074, 1031, 931, 870, 706, 687; 1H NMR (DMSO-d 6, TMS): ΔH 3.86 (3H, s, -OCH3), 6.97–7.43 (9H, m, Ar); m/z (GC/MS, HRMS): 252.0893 (M+, C15H12N2O2 required 252.0899). Analysis calculated for C15H12N2O2: C, 71.42; H, 4.79; N, 11.10. Found: C, 71.40; H, 4.76; N, 11.12.
2-(3-Methoxyphenyl)-5-phenyl-1,3,4-oxadiazole (3g, C15H12N2O2)
ν max (KBr) cm−1 3119, 1632, 1532, 1445, 1377, 1288, 1102, 1078, 1030, 934, 878, 707, 687; 1H NMR (DMSO-d 6, TMS): ΔH 3.86 (3H, s, -OCH3), 6.97–7.43 (9H, m, Ar); m/z (GC/MS, HRMS): 252.0896 (M+, C15H12N2O2 required 252.0899). Analysis calculated for C15H12N2O2: C, 71.42; H, 4.79; N, 11.10. Found: C, 71.45; H, 4.78; N, 11.11.
2-(4-Nitrophenyl)-5-phenyl-1,3,4-oxadiazole (3h, C14H9N3O3)
ν max (KBr) cm−1 3118, 2950, 1600, 1425, 1172, 1024, 940; 1H NMR (DMSO-d 6, TMS): ΔH 7.40–7.52 (5H, m), 8.21–8.52 (4H, m); m/z (GC/MS, HRMS): 267.0647 (M+, C14H9N3O3 required 267.0644). Analysis calculated for C14H9N3O3: C, 62.92; H, 3.39; N, 15.72. Found: C, 62.94; H, 3.36; N, 15.76.
2-(2-Furyl)-5-phenyl-1,3,4-oxadiazole (3i, C12H8N2O2)
ν max (KBr) cm−1 3150, 3120, 1630, 1562, 1544, 1500, 1467, 1350, 1180, 1139, 1100, 1080, 1052, 900; 1H NMR (DMSO-d 6, TMS): ΔH 6.64–6.67 (1H, m), 7.24 (1H, d), 7.35–7.55 (3H, m), 7.66–7.68 (1H, m), 8.12–8.14 (2H, m); m/z (GC/MS, HRMS): 212.0583 (M+, C12H8N2O2 required 212.0586). Analysis calculated for C12H8N2O2: C, 65.43; H, 7.32; N, 12.72. Found: C, 65.46; H, 7.33; N, 12.74.
2-(3-Pyridinyl)-5-phenyl-1,3,4-oxadiazole (3j, C13H9N3O)
ν max (KBr)/cm−1 3118, 2950, 1601, 1423, 1172, 1025, 947; 1H NMR (DMSO-d 6, TMS): ΔH 7.51–7.59 (4H, m), 8.14–8.17 (2H, m), 8.45–8.48 (1H, m), 8.81 (1H, d), 9.37 (1H, s); m/z (GC/MS, HRMS): 223.0748 (M+, C13H9N3O required 223.0746). Analysis calculated for C13H9N3O: C, 69.95; H, 4.06; N, 18.82. Found: C, 69.92; H, 4.04; N, 18.81.
Conclusion
In conclusion, we have shown that CAN as a cheap and readily available catalyst is highly efficient for the synthesis of 2,5-disubstituted 1,3,4-oxadiazoles. Moreover, PEG offers a convenient, inexpensive, non-ionic liquid, non-toxic, and recyclable reaction medium for the synthesis of 2,5-disubstituted 1,3,4-oxadiazoles. The recyclability of this catalyst system makes the reaction economically and potentially viable for commercial applications.
Supplementary Material
Download PDF (68.9 KB)Acknowledgements
The authors thank the University Grants Commission for their fellowships.
References
- Palaska E Sahin G Kelicen P Tugba durlu N Altinok G IL Farmaco 2002 57 101 107
- Dogan H.N Duran A Rollas S Sener G Armutak Y Keyer-Uysal M Med. Sci. Res 1998 26 755 758
- Brown P Best D.J Broom N.J.P Cassels R Hanlon P.J.O Mitchell T.J Osborne N.F Wilson J.M J. Med. Chem 1997 40 2563 2570
- Ouyang X Piatnitski E.L Pattaropong V Chen X He H.Y Kiselyov A.S Velankar A Kawakami J Labelle M Smith L Lohman J Lee S.P Malikzay A Flemming K Gerlak J Wang Y Rolser R.L Zhou K Mitelman S Camara M Surguladze D Doody J.F Tuma M.C Bioorg. Med. Chem. Lett 2006 16 1191 1196
- Singh H Yadava L.D.S Chaudharg J.P Acta Chem. Hung 1985 118 11 16
- Yale H.L Losee K J. Med. Chem 1966 9 478 483
- Diana G.D Volkots D.L Nitz T.J Biailly T.R Long M.A Vesico N Aldous A Pevear D.C Dukto F.J J. Med. Chem 1994 37 2421 2436
- Tandon V.K Chhor R.B Synth. Commun 2001 31 1727 1732
- Carlsen H.J Jorgensen K.B J. Heterocycl. Chem 1994 31 805 808
- Nair V Balagopal L Rajan R Mathew J Acc. Chem. Res 2004 37 21 30
- Nair V Deepthi A Chem. Rev 2007 107 1862
- Sridharan V Perumal P.T Avendano C Menendez J.C Tetrahed. Lett 2007 63 4407 4413
- Das B Krishnaiah M Venkateswarlu K Reddy S Tetrahed. Lett 2007 48 81 83
- Sridharan V Menendez J.C Org. Lett 2008 10 4303 4306
- Chen J Spear S.K Huddleston J.G Rogers R.D Green Chem 2005 7 64 82
- Kidwai M Bhatnagar D Mishra N.K Bansal V Catal. Commun 2008 9 2547 2549
- Kidwai M Singhal K Thakur R Lett. Org. Chem 2005 2 419 423
- Park Y-D Kim J-J Chung H-A Kweon D-H Cho S-D Lee S-G Yoon Y-J Synthesis 2003 4 560 564
- Francis J.E Cash W.D Barbaz B.S Bernard P.S Lovell R.A Mazzenga G.C Friedmann R.C Hyun J.L Braunwalder A.F Loo P.S Bennett D.A J. Med. Chem 1991 34 281 287
- Saeed A Chem. Het. Compds 2007 43 1072 1077
- Shang Z Reiner J Chang J Zhao K Tetrahed. Lett 2005 46 2701 2704
- Jacobsen N.W Philippides A.E Aust. J. Chem 1986 39 1911 1914
- Rostamizadeh S . Ghasem Housaini S.A Tetrahed. Lett 2004 45 8753 8756