Abstract
p-Aminophenol was prepared by hydrogenation of p-nitro phenol over nano-sized nickel catalysts supported on two different supports, SiO2 and Al2O3. Hydrazine hydrate was used as hydrogen source in this reaction. Several loadings of nano-sized Ni were used, thus 20, 5, and 2.5 wt% were prepared. X-ray diffraction (XRD) and electron spin resonance (ESR) were employed to investigate the prepared catalysts. The Ni/Al2O3 was found to be more effective and give high durability. The catalytic activity of the reaction was found to be influenced by both the crystallinity of the nickel and the strain among nano-sized nickel particles. The prepared catalysts showed higher catalytic activity, especially at lower loading. During the reaction, a detectable change of the color was observed from yellow to green and finally to colorless, which enable us to suppose a mechanism of this reaction.
Introduction
Catalysis is considered to be highly valuable in science. This is partly because using the appropriate catalyst will reduce energy and thus reduce the impact on the environment. In addition, catalysis is listed as one of the principles of green chemistry. The most important challenge in catalysis is to synthesize a catalyst with 100% yield and 100% selectivity. With respect to the environment, the catalyst that reduces waste and is economical will be the better catalyst Citation1 Citation2.
p-Aminophenol (PAP) is considered to be one of the most important intermediates in the manufacturing of many analgesic and antipyretic drugs, such as paracetamol, acetanilide, and Phentacin Citation3–9. It can be also utilized as a developer in photography with trade names of activol and azol and also in chemical dye industries Citation10 .
There are many methods used in the preparation of PAP from p-nitrophenol (PNP) such as metal/acid reduction Citation11, catalytic hydrogenation Citation12, electrolytic reduction Citation13, homogeneous catalytic transfer hydrogenation Citation14, and heterogeneous catalytic transfer hydrogenation. Although all of these methods are still used today, each method has its shortcoming. For instance, the metal/acid system is not selective and requires strong acidic medium. The catalytic hydrogenation uses hydrogen gas with drastic conditions at high pressure. The electrolytic reduction needs acidic or alkaline catholite, and the desired product yield is very low. The homogeneous catalytic hydrogenation uses expensive catalysts in the form of complexes. Although the heterogeneous catalytic hydrogenation with supported metals (palladium, platinum, or ruthenium) is very effective, the complexes need special attention while dealing with them, because of their flammable nature in the presence of air.
Among all previously mentioned methods, direct catalytic hydrogenation of PNP to PAP becomes the most important one, because it could be an efficient and greener route for synthesis Citation9. Raney nickel Citation15, nano-sized nickel Citation16, and several noble metal catalysts such as Pd/C9 have been used as catalysts for this reaction. Due to their cost-effectiveness and their higher catalytic activity, supported nickel catalysts are widely used in such reactions Citation17–23. Although the supported and unsupported nickel catalysts have been used for a long time in hydrogenation reactions, only a few recently published manuscripts deal with hydrogenation of PNP with nano nickel metal catalysts Citation24–27.
Most of this recent research used nano supported or unsupported nickel metal catalyst with high pressure of molecular hydrogen as a hydrogen source. Although nano nickel catalyst showed high catalytic activity, it requires high relative temperature and pressure, which is not economically viable. The use of alternative hydrogen sources for hydrogenation of PNP to PAP has been reported very little in the literature. For example, hydrazinum monoformate was used with Raney nickel as an alternative hydrogen source for the hydrogenation of aromatic nitro compounds Citation25. However, the use of hydrazine monoformate has several disadvantages such as the possibility of interaction of nickel with a formate anion to form nickel format.
In this manuscript, we report herein on the utility of hydrazine hydrate as an alternative source of hydrogen with supported nano nickel catalysts as a system for reduction of aromatic nitro compounds using PNP as an example. Only H2 and N2 are the products of the catalytic decomposition of hydrazine hydrate used over nano-sized nickel catalyst in this reduction system. We used SiO2 and Al2O3 as supports for the nano nickel particles comparing them to unsupported commercial Raney nickel catalyst. XRD and ESR techniques were employed to characterize the prepared nano-sized nickel catalysts.
Results
XRD studies
XRD analysis was applied to follow up the change in the crystallinity of Ni metal phase before and after using the catalysts samples in the reaction. Thus, the Ni metal phase ((a)) in 20 wt% Ni/SiO2 catalyst sample exhibits an observable degree of crystallinity. Moreover, after using the catalyst for five successive times with a ratio of 1:5 wt:wt Ni:PNP nearly no crystalline Ni could be observed from XRD diffractograms ((b)).
Figure 1. XRD diffractograms of 20 wt% Ni/SiO2 catalyst (a) freshly prepared and (b) after fifth time of using.
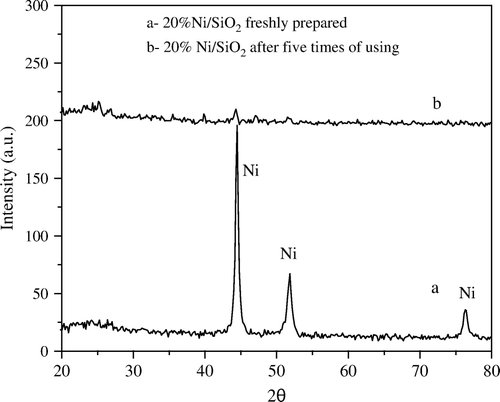
In contrast to the supported SiO2 catalyst, the degree of the crystallinity of Ni on the Al2O3 support remains nearly unchanged after using the catalyst for five times under the same conditions (). This may be explained by the possibility of easier transformation of nickel from crystalline into amorphous phase on SiO2 than that on Al2O3 support. Moreover, this may be related to the difference in metal support interaction between SiO2 and Al2O3.
Figure 2. XRD diffractograms of 20 wt% Ni/Al2O3 catalyst (a) freshly prepared and (b) after fifth time of use.
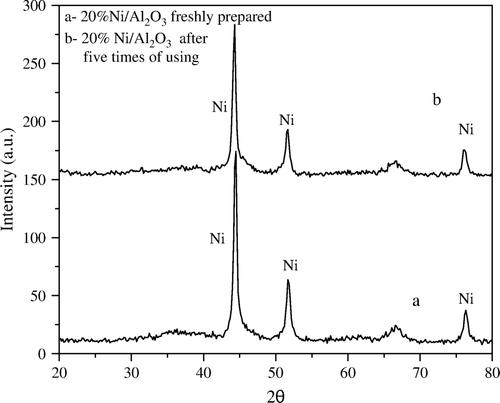
Furthermore, the XRD diffractograms of 5 wt% Ni/SiO2 catalyst before and after using two times with ratio 1:5 wt:wt Ni:PNP (), showed a sharp decrease in the degree of crystallinity of Ni metal after only two times of use.
Figure 3. XRD diffractograms of 5 wt% Ni/SiO2 catalyst (a) freshly prepared and (b) after second time of use.
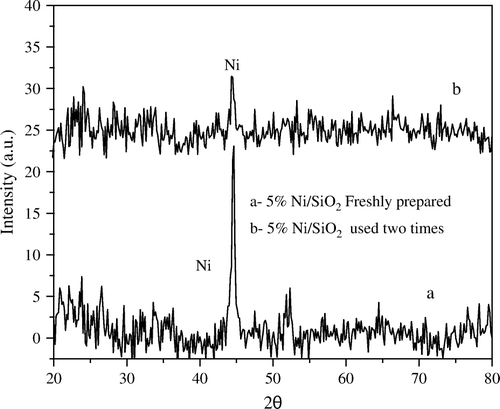
In contrast, the XRD diffractograms of 5 wt% Ni/Al2O3 catalyst before and after using five times with ratio 1:5 wt:wt Ni:PNP (), exhibit only a very small change in the degree of crystallinity of Ni metal.
Figure 4. XRD diffractograms of 5 wt% Ni/Al2O3 catalyst (a) freshly prepared and (b) after fifth time of use.
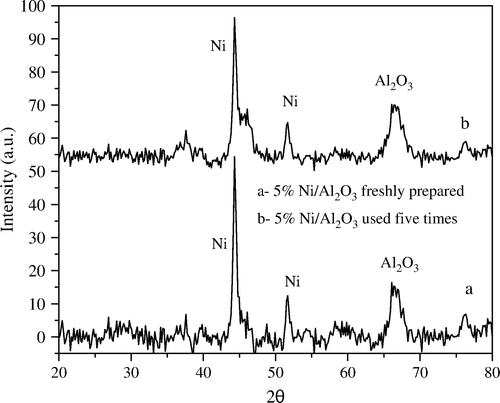
Moreover, the XRD diffractograms of 2.5 wt% Ni/SiO2 and Ni/Al2O3 catalysts showed no crystalline Ni metal at all which attributed to the very small amount of Ni loaded being beyond the detection limit of the XRD technique. The crystallite size of Ni for all samples under investigation was determined according to the Scherrer equation Citation28:
ESR spectra
The ESR technique is employed in this research to investigate the nickel metal on different catalyst samples before and after use in the hydrogenation reaction. The ESR signals were found to be suffering from severe anisotropy (Figures ), especially at higher loading concentrations. In addition, lower magnetic field signals are observed and found to overlap the other signals over the whole range of the magnetic field. This may be explained if we assume a spin–spin interaction to exist. To explain this assumption, it may be better to take a look at the electronic configuration of the nickel metal. Thus, Ni(0) contains two electrons in the 3d orbital; however, these electrons seem to be degenerate and can not result in such severe interactions appearing in the ESR spectra. The only remaining possibility to explain this phenomenon is that there is a spin–spin interaction between nano nickel particles or, in other words, strain between nano nickel particles Citation28–30.
Figure 7. ESR spectra of 20 wt% Ni/SiO2 catalyst, freshly prepared and after fifth time of using at 1:5 wt:wt of Ni:PNP.
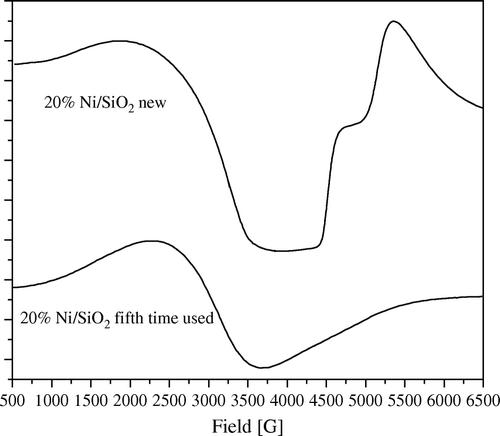
Figure 8. ESR spectra of 20 wt% Ni/Al2O3 catalyst, freshly prepared and after fifth time of using at 1:5 wt:wt of Ni:PNP.
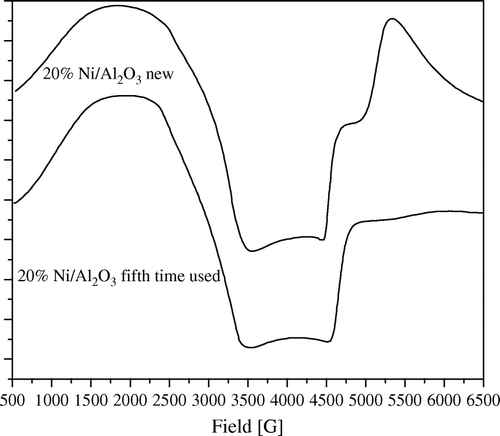
Figure 9. ESR spectra of 5 wt% Ni/SiO2 catalyst, freshly prepared and after fifth time of using at 1:5 wt:wt of Ni:PNP.
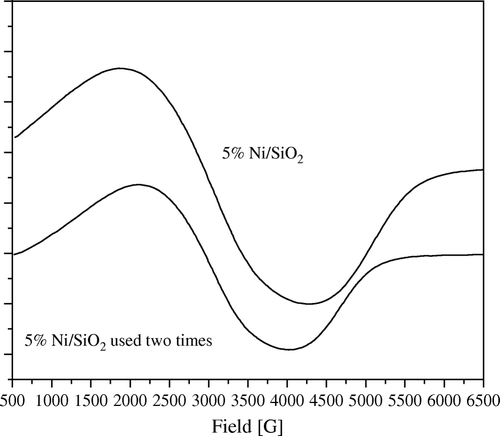
Figure 10. ESR spectra of 5 wt% Ni/Al2O3 catalyst, freshly prepared and after fifth time of using at 1:5 wt:wt of Ni:PNP.
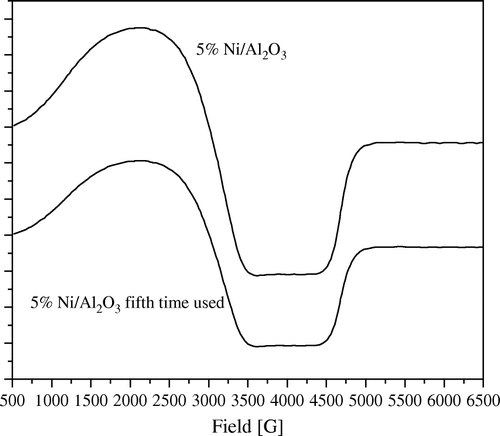
Figure 11. ESR spectra of 20 wt% Ni/SiO2 as it is and after solid–solid dilution with bare SiO2 till anisotropy disappears.
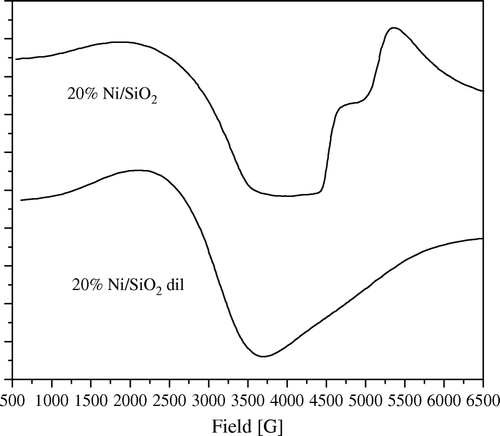
To prove this assumption, we make a successive solid–solid dilution of each sample with its bare support until all anisotropy of the ESR signal disappears (i.e. only one isotropic signal centered at g=2.2 related to nickel metal appeared, e.g. ). From this signal, the particle size of nickel can be estimated. Thus, in 1970 Kawabata Citation31 demonstrated his famous relation hypothesizing that the broadening of ESR signal of nano metal particles is affected by the quantum size effect and can be correlated to the size of the nano metal particle.
Accordingly, direct relationship between the line width ΔHpp (or peak-to-peak width) of the signal of nano particle in ESR spectra and its particle size is given in the following relation:
The proportionality constant “a” for nickel was previously determined as 1.2 Citation32. The particle size of investigated samples was calculated in .
Table 1. Particle size of investigated samples using XRD and ESR.
In addition to the previous phenomenon, much useful information can be extracted by deeply analyzing the ESR spectra of samples under investigations. Thus from and , it can be observed that as the amount of loading of Ni increases, the amount of anisotropy of the ESR signal increases. Also 2.5 wt% Ni loading samples for both SiO2 and Al2O3 showed nearly no signal which can be explained that at this concentration nearly no reduction of Ni can take place at these mild conditions of reduction.
Moreover, it can observed that the amount of anisotropy is proportional to the amount of crystalline Ni metal. Thus, in the fifth use of 20 wt% Ni/SiO2 catalyst showed nearly no anisotropy accompanied with a sharp decrease in crystallinity of Ni as confirmed by XRD diffractograms (). Moreover, anisotropy was still observed in the fifth use of 20 wt% Ni/Al2O3 catalyst where XRD diffractograms showed that it still has a crystalline Ni metal ().
The same observation can be also shown in low loading samples of 5 wt% Ni ( and ).
In addition, the ESR spectra of commercial Raney nickel () showed a severe anisotropy, with low magnetic field overlapping signals. This behavior was also confirmed by similar catalytic activity of high loading catalyst and Raney nickel ().
Discussion
Hydrazine was utilized as the hydrogen donor in the reduction process of PNP over different nano supported nickel catalysts. The reaction was found to be completed within only a few minutes. In addition, the observable change in the color during the reaction from yellow to green to colorless, facilitated the suggestion of the mechanism of this reaction.
Mechanism of hydrogenation
During the hydrogenation process, the change in color was observed in a sequence of: (1) yellow color of PNP; (2) green color (intermediate A); and then (3) discharge of all color (PAP) accompanied with 100% conversion.
The following mechanism showed that H2 (generated from the decomposition of hydrazine over Ni metal) is added first to the nitro group; after which, the elimination of two molecules of water was accompanied by a rearrangement of the molecule to form the green intermediate (A) (). The addition of another H2 molecule to the green intermediate is then accompanied by the formation of PAP.
Catalytic activity
Since the end of the reaction was found to be self indicated by a change in color at 100% conversion, the catalytic activity may be better expressed as the time to reach 100% conversion through this color change. Different ratios of PNP to Ni metal were varied and the catalytic activity of each was measured. shows the time to completion for reactions with different concentrations of catalyst.
Table 2. Effect of concentration of PNP:Ni on the catalytic activity.
The results indicated that a 5 wt% Ni/Al2O3 is the best catalyst concentration, with the lower time to reach 100% conversion at different ratios of Ni:PNP.
Moreover, in order to completely characterize the catalyst we also studied the durability of the catalysts of 1:5 wt:wt Ni:PNP, using the catalyst up to five times as shown in .
Table 3. Durability of the catalysts after five times of using at concentration of 1:5 wt:wt Ni:PNP.
Deeply analyzing the above data, very useful information regarding the catalytic activity of the catalysts prepared can be extracted. Thus, the catalytic activity of 5 wt% Ni supported on either SiO2 or Al2O3 at lower ratios of Ni:PNP (1:5 wt:wt) exhibits a higher activity compared with those of higher loading of Ni (20 wt%). Moreover, at higher ratios of Ni:PNP (1:10 and 1:15 wt:wt) the 5 wt% Ni/Al2O3 showed superior catalytic activity compared to the 5 wt% Ni /SiO2 support. This can be explained by the ease of transformation of crystalline nickel into amorphous phase over SiO2 due to the weak metal support interaction. Moreover, the catalytic activity seems to be proportional to the crystalline nickel only. This behavior is confirmed during the durability test () where a sharp decrease in the catalytic activity of 20 wt% Ni/SiO2 at the fifth time of successive using (40 min) is accompanied with the disappearance of crystalline nickel, as shown by XRD diffractograms (). In the same manner, the 5 wt% Ni/SiO2 at the second time of use also showed the disappearance of crystalline nickel (). Moreover, this behavior is accompanied by decrease in particle size of nickel as evidenced by ESR determination of particle size ().
In addition, the increase of the catalytic activity of low loading Ni on both SiO2 and Al2O3 supports suggests that not all nickel species act as active centers for this reaction. This can be viewed from two aspects, first, the increase of nickel loading will decrease the dispersion of nickel and hence not all nickel can be susceptible to the reactants; the second aspect is that the neighboring nano-sized nickel particles can influence each other as evidenced by ESR. It was also found that, as the amount of the existence of such strain among nano-nickel particles increases, the number of active nickel species decreases. Moreover, the catalytic activity was found to be proportional only to the crystalline nickel and this leads us to believe that the Al2O3 support is more effective than the SiO2 support where, even at lower loading (5 wt%), the catalytic activity and durability are still very high.
In addition to all these observations, all catalyst samples were higher in catalytic activity than commercial Raney nickel. Moreover, the supported catalyst has the advantage of easily handling and the possibility of reuse of the catalyst more than one time, as compared to the impossibility to reuse Raney nickel where almost all nickel is lost during the filtration process at this low concentration of nickel.
Experimental
Materials
NiSO4.7H2O, Al(OH)3, commercial Raney nickel, ydrazine hydrate 80%, PNP, PAP (as a standard materials), and methanol (spectroscopic grade) were purchased from Merck Co., Germany.
Commercial silica was obtained from Brownell limited Co., London. X-ray diffractograms were collected using Bruker D8 advance instrument with CuKα1 target operated at 40 kV and 40 mA. ESR spectra were measured using (Brucker Elexsys. 500) operated at X-band frequency. The following parameters are generalized to all samples otherwise mentioned in the text: microwave frequency, 9.73 GHz; receiver gain, 20; sweep width, 6000 center at 3480 G; and microwave power, 0.00202637 Watt.
Preparation
Nickel was loaded on Al2O3 (obtained by calcination of Al(OH)3 at 550°C for 4 hours) and SiO2 by means of impregnation method to be 20, 5, and 2.5 wt%. Hydrazine hydrate was used to reduce the catalysts in order to obtain the metallic form of nickel. Solid–solid dilution is done by adding bare supports of SiO2 or Al2O3.
Hydrogenation process
The hydrogenation process was performed by dissolving PNP in an appropriate amount of methanol followed by the addition of hydrazine hydrate as the hydrogen source and heating at 80°C. The catalyst was then added to the heated solution and the time to reach 100% conversion (as examined by thin layer chromatography (TLC) and IR spectra) was taken as an expression of the catalytic activity.
The filtrate was then evaporated at reduced pressure and the residue was recrystallized from hot water to give pure product of PAP in almost 100% yield.
The characteristics of the product, PAP, are listed below:
Conclusion
In this study we found that the use of hydrazine as a hydrogen source was very effective for the reduction of PNP into PAP. The use of supported nano-sized nickel was found to be very effective in such reactions. The catalytic activity reached three times higher than the commercial Raney nickel in the case of 5 wt% Ni/Al2O3 catalyst of a much higher concern may be the possibility of reuse the supported catalysts more than one time in the reaction. The catalytic activity of nickel is influenced by the existence of strain among nano nickel particles and is proportional to only the crystalline nickel. Ni/Al2O3 catalyst has proved to be more effective than Ni/SiO2 catalyst, where high durability and high catalytic activity of Ni/Al2O3 catalyst were attained. The mechanism of the hydrogenation reaction is guided according to the change in color during the reaction. The change in color in the reaction makes it a self-indicator of 100% conversion, which decreases the time and effort for following up the reaction.
References
- Trost , B.M. Science 1991 , 254 , 1471 1477 .
- Sheldon , R.A. Green Chem . 2007 , 9 , 1273 1283 .
- Rode , C.V. ; Vaidya , M.J. ; Jaganathan , R. ; Chaudhari , R.V. Chem. Eng. Sci . 2001 , 56 , 1299 1304 .
- Rode , C.V. ; Vaidya , M.J. ; Chaudhari , R.V. Org. Process Res. Dev . 1999 , 3 , 465 470 .
- Komatsu , T. ; Hirose , T. Appl. Catal. A: Gen . 2004 , 276 , 95 102 .
- Sathe , S.S. Process for preparing p-aminophenol in the presence of dimethyldodecylamine sulfate. US Patent 4176138, 1979 .
- Lee , L.T. ; Chen , M.H. ; Yao , C.N. Process for manufacturing p-aminophenol . US Patent 4885389, 1989 .
- Yun , K.S. ; Cho , B.W. Process for preparing paraaminophenol . US Patent 066369, 1991 .
- Vaidya , M.J. ; Kulkami , S.M. ; Chaudhari , R.V. Org. Process Res. Dev . 2003 , 7 , 202 208 .
- Venkatraman , K. The Chemistry of Synthetic Dyes ; Academic Press : New York , 1952 I 184 .
- House , H.O. Modern Synthetic Reactions , 2nd ed. Benjamin : New York , 1977 145 .
- Rylander , P.N. Hydrogenation Methods ; Academic : New York , 1985 365 .
- Popp , F.D. ; Schultz , H.P. Chem. Rev . 1962 , 62 , 19 .
- Harmon , R.E. ; Gupta , S.K. ; Brown , D.J. Chem. Rev . 1972 , 72 , 21 52 .
- Chen , R.Z. ; Du , Y. ; Chen , C.L. ; Xing , W.H. ; Xu , N.P. ; Chen , C.X. ; Zhang , Z.L. J. Chem. Ind. Eng. (China) 2003 , 54 , 704 706 .
- Du , Y. ; Chen , H.L. ; Chen , R.Z. ; Xu , N.P. Synthesis of p-aminophenol from p-nitrophenol over nano-sized nickel catalysts . Appl. Catal. A: Gen . 2004 , 277 , 259 264 .
- Rautanen , P.A. ; Aittamaa , J.R. ; Krause , A.O.I. Chem. Eng. Sci . 2001 , 56 , 1247 1253 .
- Suh , D.J. ; Park , T.J. ; Lee , S.H. ; Kim , K.L. J. Non-Cryst. Solids 2001 , 285 , 309 316 .
- Wu , W.H. ; Xu , J. Catal. Commun . 2004 , 5 , 591 595
- Wu , W.H. ; Xu , J. ; Ohnishi , R. Appl. Catal. B: Environ . 2005 , 60 , 129 137 .
- Kapoor , M.P. ; Matsumura , Y. J. Mol. Catal. A: Chem . 2002 , 178 , 169 172 .
- Toppinen , S. ; Rantakyh , T.K. ; Salmi , T. ; Aittamaa , J. Catal. Today 1997 , 38 , 23 30 .
- Quincoces , C.E. ; Gonzfilez , M.G. Kinetic study on Coz refroming of methane . Chin. J. Chem. Eng . 2001 , 9 , 190 195 .
- Rizhi , C. ; Yan , D.U. ; Weihong , X. ; Nanping , X.U. Chinese J. Chem. Eng . 2006 , 14 , 665 669 .
- Shankare , G. ; Channe , G. Tetrahedron 2002 , 58 , 2211 2213 .
- Chang , Y.C. ; Chen , D.H. J. Hazard Mater . 2009 , 165 , 664 669 .
- Yan , D. ; Hongling , C. ; Rizhi , C. ; Nanping , X. Appl Catal A: Gen . 2004 , 277 , 259 264 .
- Zhang , F. ; Chan , S.W. ; Spanier , J.E. ; Apak , E. ; Jin , Q. ; Robinson , R.D. ; Herman , I.P. Appl. Phys. Lett . 2002 , 80 , 127 129 .
- Sharma , V.K. ; Baiker , A. J. Chem. Phys . 1981 , 75 , 5596 5601 .
- Suran , G. ; Stankoff , A. ; Hofmann , F. Phys. Rev. B . 1973 , 8 , 1109 1118 .
- Kawabata , A. J. Phys. Soc. Jpn . 1970 , 29 , 902 911 .
- Selim , M.M. ; Abd El-Maksoud , I.H. Microporous Mesoporous Mater . 2005 , 85 , 273 278 .