Abstract
The oxidation of secondary alcohols into corresponding ketones has been described using heterogeneous nanocrystalline TiO2 as catalyst at a moderate temperature with quantitative yields. Use of H2O2 instead of O2 (g) is the main feature of our methodology since maintenance of PO2 (g) at elevated temperature is not easy. Besides, this catalyst could be recovered and reused for further reactions with consistent activity. Hence, our present protocol is economical and throughout clean consisting of green reagent, solvent, and catalyst.
Introduction
The oxidation of alcohols to carbonyl compounds has great importance in synthetic organic chemistry due to their utility in variety of products, such as drugs, agro-chemicals, and fragrances Citation1 Citation2. Alcohols have conventionally been oxidized by noncatalytic methods using stoichiometric amount of hazardous oxidants such as chromium and manganese compounds in the presence of strong mineral acids, which generate enormous amounts of poisonous metal salts as waste Citation3–5 .
In view of increasing environmental concerns, many efforts have been made to develop the environmentally benign oxidation systems Citation6 . In addition to this, various heterogeneously catalyzed protocols were reported, including the use of silver nanoparticles supported on hydrotalcites Citation7, metallic iron nanoparticles on MCM-41 Citation8, and highly expensive gold and palladium nanoparticles supported on metal oxides Citation9–12. However, most of the systems could be applicable for the oxidations of only activated substrates by using molecular O2 (g) Citation13, or large quantities of additives such as bases and electron transfer mediators are required Citation14 Citation15. Besides this, lethal V2O5 and ruthenium catalyst have also been used as catalysts in the toxic solvents such as toluene Citation16 Citation17.
Some of the methods could be considered green but these methods are not throughout clean because sometimes these protocols utilize green catalyst but neither solvent nor reagent is green; sometimes green solvents are used but catalysts and reagents are not under the periphery of greener reactions and vice versa. So there is a strong need to develop such a catalytic system which comprises all, that is, green catalyst, solvent, and reagent.
Industry favors catalytic processes induced by heterogeneous catalysts over homogeneous processes in view of the ease of handling, simple work-up, and recyclability. Titanium dioxide is a green heterogeneous prominent material for various kinds of industrial applications, for example, in the selective reduction of NOx in stationary sources, organic synthesis, photovoltaic devices, and sensors Citation18–22. Despite this, researchers are not able to explore catalytic activity of sole nanocrystalline titanium (IV) oxide for oxidation reactions.
We chose polyethylene glycol (PEG) as solvent because PEG and its derivatives are commonly known to be inexpensive, thermally stable, environmentally benign media for chemical reactions and moreover having an almost negligible vapor pressure Citation23–25.
In continuation of our progressive program toward the development of green and sustainable synthetic methods Citation26–28 for organic synthesis and the role of transition metal catalysts in such syntheses Citation29 Citation30, we would like to report a novel and efficient method for the oxidation of secondary alcohols to ketones using green catalytic system which involves TiO2-np as heterogeneous catalyst, H2O2 as reagent, and PEG as green solvent.
Results and discussion
To validate our hypothesis, we initially examined the oxidation of diphenylcarbinol in absence of nanocrystalline TiO2. Reaction was performed in PEG-400 using equivalent amount of diphenylcarbinol and aqueous H2O2. This reaction mixture was continuously stirred at 70–75°C in oil bath for 6 hours ().
Under these reaction conditions diphenylcarbinol was converted into benzophenone with only 27% yield. Then the conversion was further improved by introducing 10 mol% of nanocrystalline TiO2 and surprisingly yield reached up to 92%. Furthermore, the reaction temperature had a great influence on this transformation. The reaction gave only 40% conversion at 40–50°C and in case of diphenylcarbinol, traces of product were obtained.
Different nanoparticles were used to catalyze the same oxidation reaction. But nano TiO2 was found to be the most effective among all the catalysts used ().
Table 1. Oxidation of alcohols using different nanocrystalline metal oxides.a
The increased catalytic activity of nano-TiO2 over the commercially available bulk TiO2 and other nano oxides may be attributed to the higher surface area for nano-TiO2. This is thought to be due to morphological differences; whereas larger crystallites have only a small percentage of reactive sites on the surface, smaller crystallites will possess a much higher surface concentration of such sites (shown in powder XRD pattern in ) crystal corners, edges or ion vacancies Citation31.
Next, we investigated the effect of different solvents. Solvents having low boiling point did not give good results because reaction was performed at 70–75°C. Due to environment point of view, we chose PEG as a solvent then we have screened our reaction with PEG having different molecular weights. We found that the conversion was gradually decreased with increase in the molecular weight of the PEG from 400 to 800, being presumably ascribed to the increasing mass limitation of dispersion of TiO2 nano powder in the liquid phase which decreased the activity of the catalyst ().
Table 2. Screening of solvent for oxidation reaction.
Different oxidizing agents were used to oxidize the diphenylcarbinol under similar reaction conditions. Hydrogen peroxide was found to be an excellent oxidizing agent. The reason is that active oxygen availability in case of hydrogen peroxide is higher in comparison to other oxidants Citation15.
It was important to optimize the concentration of H2O2 to get maximum yield of product. For this, the same amount of alcohol was treated with different concentrations of hydrogen peroxide as depicted in . We found that equivalent amount of hydrogen peroxide gave maximum yield of product without giving other side products. Notably, reaction could not be performed without aqueous H2O2 as the oxidant.
Figure 2. Optimization of concentration of H2O2. Reaction conditions: diphenylcarbinol (1 mmol), hydrogen peroxide (1 mmol) and 10 mol% nano TiO2 (50±2) nm; PEG-400; temperature 70–75°C. Isolated yields.
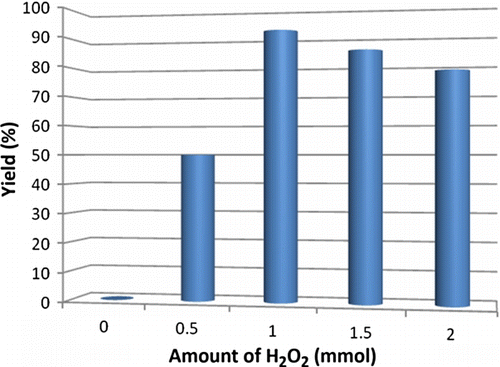
Table 3. Oxygen availability in various oxidants.
To test the generality and scope of nano TiO2 catalyzed reaction, an array of structurally divergent alcohols were tested. As shown in , both aliphatic and aromatic secondary alcohols can be easily oxidized into the corresponding carbonyl compounds in fair to high yield. In general, substrate having electron donating group showed higher activity than those having electron withdrawing groups. Moreover, groups such as –OCH3, –Cl, and –CH3 were ineffective during course of reaction.
Table 4. Nanocrystalline titanium (IV) oxide catalyzed oxidation of alcohol into ketones.a
The literature suggested that superoxide species O2 – over metal oxide and zeolitic system usually act as active species in radical reactions Citation32 Citation33. This highly active species may react with alcohol and form its free radical which could be easily converted into product as shown in .
The formation of products was confirmed by disappearance of peak between 3200 and 3500 cm–1 due to H–O bond of unsaturated alcohols and appearance of characteristic stretching bands of –C = O– at 1650–1730 cm–1 for ketones in the IR spectra. In the 1H NMR spectra disappearance of broad band of “alcoholic” proton confirmed the formation of products.
For practical applications of heterogeneous systems, the lifetime of the catalyst and its level of reusability are very important factors. To clarify this issue, we established a set of experiments for the oxidation of diphenylcarbinol using the recycled nano-TiO2 catalyst. After completion of the first reaction to afford the corresponding ketones, the catalyst was recovered by dissolving the product in chloroform followed by filtration.
TiO2 remained as residue which was then washed with ethyl acetate and finally dried at 100°C for 5 hours. A new reaction was performed with obtained nano TiO2 and fresh reactant and H2O2, under similar reaction conditions. The TiO2-nanoparticles could be reused at least four times with change in slight activity, as shown in .
Figure 3. Recycling studies of nano-TiO2 oxidation reaction. aReaction conditions: diphenylcarbinol (1 mmol), hydrogen peroxide (1 mmol) and 10 mol% nano TiO2 (50±2) nm; PEG-400; temperature 70–75°C. Isolated yields.
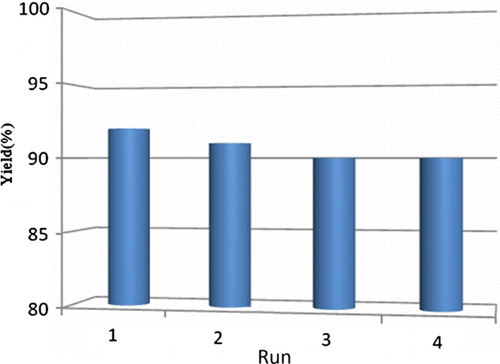
It was further confirmed by Transmission electron microscopy (TEM) technique. Both fresh and recovered catalysts were observed under TEM to understand the shape and size of the particles. a shows the fresh nano-particles having size range 20–50 nm, with well-defined shapes. b shows the TEM image of the used catalyst after four recycles. Interestingly, it was observed that the shape and size of the particles remain unchanged even after recycling.
This supports that the morphology of the catalyst remains the same even after recycling. Besides this, powder diffraction (XRD) patterns of the fresh and used nano TiO2 do not differ in the range of 2θ = 0°–70°. This also confirms the fact that the structure and morphology of the catalyst remain the same during the course of the reaction.
Experimental
General
The materials procured from Sigma-Aldrich and Merck were used without further purification. Melting points are determined on Buchi 530 (Buchi laborfeghnik AG, CH-9230, Falawil). Mass spectra were recorded in a TOF-mass spectrometer model no. KC455. 1H NMR and 13C NMR spectra were recorded on a Bruker spectrospin 400 and 100 MHz, respectively. All NMR samples were run in CDCl3, and chemical shifts are expressed as ppm relative to internal Me4Si.
Procedure for oxidation of alcohols
A measure of 10 mol% of nanocrystalline titanium (IV) oxide was added to the reaction mixture of diphenylcarbinol (1 mmol) and 30% aqueous H2O2 (1 mmol) in PEG-400 (3 ml). This reaction mixture was continuously stirred at 70–75°C in the oil bath for 6 hours. The progress of reaction was continuously monitored by Thin layer chromatography (TLC). After accomplishment of the reaction, excess of water was added and the reaction mixture was further stirred at room temperature for 2 hours. In case of solid product (Benzophenone), reaction mixture was filtered to get the solid product. This solid product was dissolved in ethyl acetate followed by centrifuge to recover the TiO2-nanoparticles. But in case of liquid, products were extracted with diethyl ether and dried over anhydrous sodium sulphate. Solvent was recovered in vacuo. Subsequently, the crude products were purified by column chromatography using 80:20 (hexane:chloroform) as an eluent.
Spectral data of some compounds
Benzophenone (Table 4, entry 1)
(White solid) IRv max(KBr) 1664 cm–1 (CO). 1H NMR (400 MHz, TMS, CDCl3): δ 7.44–7.51 (m, 4H), 7.62–7.75 (2H, m), 7.80. (4H, m). 13C NMR (100 MHz, TMS, CDCl3): δ 129.90, 132.30, 137.54, 197.21. m/z (GC-MS, HR-MS): 182.07 (M+).
Fluoren-9-one (Table 4, entry 4)
(White solid) IR v max(KBr) 1721 cm–1 (CO).1H NMR (400 MHz, TMS, CDCl3): δ 7.16–7.20 (2H, m), 7.36–7.42 (m, 4H), 7.56 (2H, J = 8Hz, d). 13C NMR (100 MHz, TMS, CDCl3): δ 120.25, 124.28, 129.00, 134.10, 134.63, 144.39, 193.87. m/z (GC-MS, HR-MS): 179.92 (M+).
1-Naphthalen-2-yl-ethanone (Table 4, entry 6)
(Brownish solid) IRv max(KBr)1675 cm–1 (CO). 1H NMR (400 MHz, TMS, CDCl3): δ 2.62 (3H, s), 7.42–7.54 (2H, 2m), 7.76–7.82 (2H, m), 7.87 (1H, J = 8 Hz, d), 7.92–7.96 (1H, Jab=8 Hz, Jac=4 Hz, dd), 8.37 (1H, s). 13C NMR (100 MHz, TMS, CDCl3): δ 26.67, 123.82, 126.74, 127.77, 128.37, 130.16, 132.44, 134.40, 135.54, 198.04. m/z (GC-MS, HR-MS): 168.96 (M+).
Conclusion
We have developed an economical and facile method for the oxidation of secondary alcohols into corresponding ketones. Simple work-up, mild reaction conditions, and only water as a side product make this present protocol eco-friendly. In addition to this recyclability of nano TiO2, use of nontoxic solvent makes this protocol imperative in the field of organic synthesis.
Acknowledgements
Saurav Bhardwaj and Arti Jain thank CSIR and UGC, respectively, New Delhi, for their fellowships.
References
- Anastas , P.T. Bartlett , L.B. Kirchhoff , M.M. Williamson , T.C. Catal. Today 2000 , 55 , 11 .
- R. Sheldon , J.K. Kochi . Metal Catalyzed Oxidations of Organic Compounds , Academic Press , New York , 1981 .
- Matsumoto , T. ; Ueno , M. ; Wang , N. ; Kobayashi , S. Chem. Asian J . 2008 , 3 , 196 .
- Mallat , T. ; Baiker , A. Chem. Rev . 2004 , 104 , 3037 .
- Hackett , S.F.J. ; Brydson , R.M. ; Gass , M.H. ; Harvey , I. ; Newman , A.D. ; Wilson , K. ; Lee , A.F. Angew. Chem. Int. Ed . 2007 , 46 , 8593 .
- Jamwal , N. ; Gupta , M. ; Paul , S. Green Chem . 2008 , 10 , 999 .
- Mitsodume , T. ; Mikami , Y. ; Funai , H. ; Mizugaki , T. ; Jitsukawa , K. ; Kaneda , K. Angew. Chem. Int. Ed . 2008 , 47 , 138 .
- Gonzalez-Arellano , C. ; Campelo , J.M. ; Macquarrie , D.J. ; Marinas , J.M. ; Romero , A.A. ; Luque , R. ChemSusChem 2008 , 1 , 746 .
- Zheng , N. ; Stucky , G.D. J. Am. Chem. Soc . 2006 , 128 , 14278 .
- Mori , K. ; Hara , T. ; Mizugaki , T. ; Ebitani , K. ; Kaneda , K. J. Am. Chem. Soc . 2004 , 126 , 10657 .
- Chen , J. ; Zhang , Q. ; Wang , Y. ; Wan , H. Adv. Synth. Catal . 2008 , 350 , 453 .
- Enache , D.I. ; Edwards , J.K. ; Landon , P. ; Solsona-Espriu , B. ; Carley , A.F. ; Herzing , A.A. ; Watanabe , M. ; Kiely , C.J. ; Knight , D.W. ; Hutchings , G.J. Science 2006 , 311 , 362 .
- Lu , A.-H. ; Li , W.-C. ; Hou , Z. ; Schuth , F. Chem. Commun . 2007 , 1038 .
- Kotani , M. ; Koike , T. ; Yamaguchi , K. ; Mizuno , N. Green Chem . 2006 , 8 , 735 .
- Karimi , B. ; Abedi , S. ; Clark , J.H. ; Budarin , V. Angew. Chem. Int. Ed . 2006 , 45 , 4776 .
- Ebitani , K. ; Motokura , K. ; Mizugaki , T. ; Kaneda K. Angew. Chem. Int. Ed . 2005 , 44 , 3423 .
- Rout , L. ; Nath , P. ; Punniyamurthy T. Adv. Synth. Catal . 2007 , 349 , 846 – 848 .
- Bosh , H. ; Janssen , F. Catal. Today 1988 , 2 , 369 .
- Forzatti , P. Catal. Today 2000 , 62 , 51 .
- Hoffman , M.R. ; Martin , S.T. ; Choi , W. ; Wahneman , D.W. Chem. Rev . 1995 , 95 , 69 .
- Maldoti , A. ; Molinari , A. ; Amadeni , R. Chem. Rev . 2002 , 102 , 3811 .
- Kantam , M.L. ; Laha , S. ; Yadav , J. ; Sreedar , B. Tetrahedran Lett . 2006 , 47 , 6213 – 6216 .
- Chen , J. ; Spear , S.K. ; Huddleston , J.G. ; Rogers , R.D. Green Chem . 2005 , 7 , 64 .
- Du , Y. ; Wu , Y. ; Liu , A.-H. ; He , L.-N. J. Org. Chem . 2008 , 73 , 4709 .
- Wang , J.-Q. ; Cai , F. ; Wang , E. ; He L.-N. Green. Chem . 2007 , 9 , 882 .
- Kidwai , M. ; Poddar , R. ; Diwaniyan , S. ; Kuhad , R.C. Adv. Synth. Catal . 2009 , 351 , 589 .
- Kidwai , M. ; Poddar , R. Catal Lett . 2008 , 124 , 311 .
- Kidwai , M. ; Bhardwaj , S. ; Poddar , R. Beil. J. Org. Chem . 2010 , 6 , 35 .
- Kidwai , M. ; Bhardwaj , S. ; Mishra , N.K. ; Bansal , V. ; Kumar , A. ; Mozumdar , S. Catal. Commun . 2009 , 10 , 1514 .
- Kidwai , M. ; Bhardwaj , S. Appl. catal. Gen. A 2007 , 9 , 742 .
- Sheldon , R.A. Chem. Commun . 2008 , 3352 – 3365 .
- Lunsford , J.H. Adv. Catal . 1972 , 22 , 265 .
- Zhao , Q. ; Bao , X.-H. ; Wang , Y. ; Lin , L.-W. ; Guo , X.-W. ; Wang X.-S. , Jal of Molecular Catalysis A: Chemical , 2000 , 157 , 265 – 268 .
Supporting Information
A green oxidation protocol for the conversion of secondary alcohols into ketones using heterogeneous nanocrystalline titanium (IV) oxide in PEG.
Contents:
1: General experimental details.
2: Experimental characterization data for all compounds.
3: Copies of product 1H NMR, IR and 13C NMR. (1, 4, 5, 6)
Mazaahir Kidwai* and Saurav Bhardwaj and Arti jain
Green Chemistry Research Laboratory, Department of Chemistry, University of Delhi, Delhi-110007, India
General
The materials procured from Sigma-Aldrich and Merck, were used without further purification. Melting points are determined on Buchi 530 (Buchi laborfeghnik AG, CH-9230, Falawil). Mass spectra were recorded in a TOF-mass spectrometer model no. KC455. 1H NMR and 13C NMR spectra were recorded on a Bruker spectrospin 400 and 100 MHz respectively. All NMR samples were run in CDCl3 and chemical shifts are expressed as ppm relative to internal Me4Si.
Experimental
10 mol% nanocrystalline titanium (IV) oxide were added to the reaction mixture of diphenylcarbinol (1mmol) and H2O2 (1mmol) in PEG-400 (3 ml). This reaction mixture was continuously stirred at 70–75 oC in the oil bath for 6 hrs. The progress of reaction was continuously monitored by T.L.C. After completion of the reaction, excess of water was added and the reaction mixture was further stirred at room temperature for 2 hrs. In case of solid product, reaction mixture was filtered to get the solid product. This solid product was dissolved in ethyl acetate followed by centrifuge to recover the nano TiO2. But in case of liquid, product was extracted with diethyl ether and dried over sodium sulphate. Solvent was recovered in vacuum. Subsequently the crude products were purified by column chromatography using 80:20 (hexane: chloroform) as an eluent.
1. Benzophenone (White solid) IRv max(KBr) 1664 cm−1 (CO). 1H NMR (400 MHz, TMS, CDCl3): δ 7.44- 7.51 (m, 4H), 7.62-7.75 (2H, m), 7.80. (4H, m). 13C NMR (100 MHz, TMS, CDCl3): δ 129.90, 132.30, 137.54, 197.21. m/z (GC-MS, HR-MS): 182.07 (M+).
2. 3,4-Dihydro-2H-naphthalen-1-one (Yellowish liquids) IRv max(KBr) 1681 cm−1 (CO). 1H NMR (400 MHz, TMS, CDCl3): δ 2.56 (t, J = 12 Hz, 2H), 2.68-2.80 (m, 2H), 2.91 (t, J = 12 Hz, 2H), 7.23–7.43 (m, 3H), 8.00 (1H, J = 6 Hz, d). 13C NMR (100 MHz, TMS, CDCl3): δ 23.01, 29.13, 38.83, 126.30, 127.67, 128.11, 132.27, 133.66, 144.07, 198.35. m/z (GC-MS, HRMS): 145.85 (M+).
3. 2-Methylbenzophenone (White solid) IRv max(KBr) 1665 cm−1 (CO). 1H NMR (400 MHz, TMS, CDCl3): δ 2.23 (s, 3H), 7.15–7.78 (m, 9H), 13C NMR (100 MHz, TMS, CDCl3): δ 14.20, 28.19, 127.11, 130.11, 138.41, 139.50, 187.10. m/z (GC-MS, HRMS): 196.01 (M+).
4. Fluoren-9-one (White solid) IR v max(KBr) 1721 cm−1 (CO).1H NMR (400 MHz, TMS, CDCl3): δ 7.16–7.20 (2H, m), 7.36–7.42 (m, 4H), 7.56 (2H, J = 8Hz, d). 13C NMR (100 MHz, TMS, CDCl3): δ 120.25, 124.28, 129.00, 134.10, 134.63, 144.39, 193.87. m/z (GC-MS, HR-MS): 179.92 (M+).
5. 4,4'-Dimethoxybenzophenone (White solid) IRv max(KBr) 1638 cm−1 (CO). 1H NMR (400 MHz, TMS, CDCl3): δ 3.72 (6H, s), 6.76 (2H, J = 8 Hz, d), 7.85 (2H, J = 8 Hz, d) 13C NMR (100 MHz, TMS, CDCl3): δ 55.56-55.67 (1C, J = 32, q) 112.97, 130.91, 132.38, 162.99, 194.64. m/z (GC-MS, HR-MS): 242.04 (M+).
6. 1-Naphthalen-2-yl-ethanone (brownish solid) IRv max(KBr)1675 cm−1 (CO). 1H NMR (400 MHz, TMS, CDCl3): δ 2.62 (3H, s), 7.42-7.54 (2H, 2m), 7.76–7.82 (2H, m), 7.87 (1H, J = 8 Hz, d), 7.92–7.96 (1H, Jab=8 Hz, Jac=4 Hz, dd), 8.37 (1H, s). 13C NMR (100 MHz, TMS, CDCl3): δ 26.67, 123.82, 126.74, 127.77, 128.37, 130.16, 132.44, 134.40, 135.54, 198.04. m/z (GC-MS, HR-MS): 168.96 (M+).
7. 4-Chlorobenzophenone (White solid) v max(KBr)IR 1657 cm−11H NMR (400 MHz, TMS, CDCl3): δ 7.45-7.52 (m, 4H), 7.58–7.63 (m, 1H), 7.75–7.79 (m, 4H),.13C NMR (100 MHz, TMS, CDCl3): δ 128.41, 128.70, 130.0, 131.54, 132.77, 135.91, 137.23, 138.92, 195.54,. m/z (GC-MS, HR-MS): 215.76 (M+).
8. Acetophenone (Colorlessliquid) IRv max(KBr) 1678 cm−1 (CO). 1H NMR (400 MHz, TMS, CDCl3): δ 2.55 (3H, s), 7.32-7.68 (3H, m), 7.94 (2H, J = 8 Hz, d),. 13C NMR (100 MHz, TMS, CDCl3): δ 26.47, 128.26, 128.56, 133.00, 136.95, 197.90. m/z (GC-MS, HR-MS): 121.06 (M+).
9. Cyclohexanone (Colorless liquid) IRv max(KBr) 1710 cm−1 (CO).1H NMR (400 MHz, TMS, CDCl3): δ 1.50-2.10 (6H, m), 2.35 (4H, J = 11.86 Hz, t). 13C NMR (100 MHz, TMS, CDCl3): δ 25.24, 27.10, 42.02, 211.55.m/z (GC-MS, HRMS): 97.56 (M+).
10. Cyclopentanone (Colorless liquid) IRv max(KBr) 1745 cm −1 (CO).1H NMR (400 MHz, TMS, CDCl3): δ 1.88 (4H, t), 2.33 (4H, t). 13C NMR (400 MHz, TMS, CDCl3): δ 23.30, 38.28, 220.14. m/z (GC-MS, HR-MS): 82.89 (M+).
References
(1) Y. Nishihara, Y. Inoue, M. Fujisawa, K. Takagi, Syn. Lett., 2005, 15, 2309.
(2) D. Wang, Z. Zhang, Org. Lett., 2003, 5, 4645.
(3) D. Taber, M. Sethuraman, J. Org. Chem., 2000, 65, 254.
(4) A. Echavarren, J. Stille, J. Am. Chem. Soc., 1988, 5, 1557.
(5) C. Edward, G. Pan, Org. Lett. 2003, 5, 4979.