Abstract
A simple and practical method of recycling homogeneous catalysts in the Suzuki reaction using appropriate work up conditions is reported here. The commonly used and commercially available homogeneous catalysts 1 (Pd-100) dichlorobis(tri-phenylphosphine)palladium(II), 2 (Pd-106) dichloro[1,1′-bis(diphenylphosphino)ferrocene]palladium(II)-dichloromethane adduct, and 3 (Pd-118) 1,1′-bis(di-tert-butylphosphino)ferrocene]dichloropalladium(II) selected for our study and were recycled successfully for three times using simple acidic and basic work up conditions depending on the presence of amino or carboxylic acid functional group in the product.
Introduction
The Green Chemistry is the utilization of a set of principles that enhance the atom economy and reduce or eliminate the use or generation of hazardous substances in the design, manufacture, and application of chemical products. It can be achieved through many ways, for example, design of environmental friendly process, catalysis, and recycling Citation1–3. The application of transition metal mediated homogeneous catalyst for the construction of C–C, C–N, C–O bonds having wide industrial application continued to attract chemists Citation4 Citation5, because of its efficiency to carry out the reaction under mild conditions with better selectivity and yield Citation6–17. However, the stability, cost, and availability of the catalysts (ligands and metal) can limit their applications at the commercial scale Citation18 Citation19. Developing simpler and better ways of recycling of these expensive homogeneous catalysts to overcome these issues would widen their industrial application. The current methods adapted for the recycling of homogeneous catalyst involve anchoring of them on to a heterogeneous support Citation20–22 and on nanoparticles Citation23 Citation24. However, this would further add to the initial cost of the catalyst and moreover many of such anchored catalysts could be under patent protection which would have its own legal and financial issues for their commercial utilization.
Recently successful attempts on recycling of the homogeneous catalyst have been reported in the literature. For example use of membranes Citation25, supercritical liquids Citation26, ionic liquids Citation27, and polyethylene/methanol mixtures Citation28 to separate catalyst from product and further recycling have been reported in the recent literature. Recycling of Pd(OAc)2 in the presence fluorous solvent has been reported in the Heck couplings of iodoarenes and acrylic acids/esters Citation29. Dumrath et al. Citation30 have also reported the recycling of Pd(OAc)2 in the presence of cationic imidazolium-based phosphane ligands for amination aryl halides. However, generality of these techniques and their application at the commercial level needs to be evaluated. Considering the solubility of the homogeneous catalysts in many organic solvents and the insensitivity of many reactions to the presence of water we thought, to explore the possibility of recycling homogeneous Pd catalyst in the Suzuki reaction by separating the catalyst from the product through manipulation of work up conditions depending on the functional group (carboxylic acid, amine) present in the substrate/product. This idea appeared to us a feasible one, simple to adapt at the commercial scale level, and would bring the cost advantage along with an environmental benefit, as the environmental impact of many of the ligands used in the preparation of the homogeneous catalyst is currently not well understood. This article describes a simple method of recycling homogeneous catalysts 1(Pd-100), 2 (Pd-106), and 3 (Pd-118) () used in Suzuki coupling reactions wherein the product has a carboxylic acid or amine functional group. It is known that amount of catalyst required for the Suzuki reaction depends on the nature of functional group present in the reactant and property of the catalyst. After the preliminary evaluation with the selected catalyst and reactants, use of 5 mol% catalyst was found to be optimum for this study.
Results and discussion
Initially, to check the feasibility of the recycling concept of homogeneous catalyst, Suzuki reaction between phenylboronic acid (4) and 4-bromobenzoic acid (7) was considered. Reaction was carried in a mixture of 2-MeTHF and water using potassium carbonate as a base and 1 (Pd-100) catalyst (). The reaction and workup were performed under nitrogen atmosphere and all the solvents including water were degassed before use. The reaction mass after the addition of the catalyst was slowly warmed at 50–60°C and the progress of the reaction was monitored by high-performance liquid chromatography (HPLC). The reaction got completed in 4 h with 94.3% product (9a) formation by HPLC (, Entry 1). The reaction mixture was then cooled to room temperature; the aqueous layer having the product and the organic 2-MeTHF layer having the homogeneous catalyst were separated. The organic layer containing the catalyst 1 (Pd-100) was then taken for the recycling experiment. Molar quantities of reactants and a degassed solution of aqueous potassium carbonate were then added to the above separated organic layer and the reaction was carried out under the same conditions. As expected, the reaction was completed at the same time (4 h) with 96% product (, Entry 2). The layers were separated again and the organic layer containing the Pd catalyst was recycled for the next Suzuki coupling. It was good to see that the second recycle reaction was also complete with 94.5% product formation in 3 h (, Entry 3). The above experimental results indicate that the catalyst 1(Pd-100) retained its catalytic activity under aqueous work up condition even after two cycles. The aqueous layer containing the product was acidified with dilute hydrochloric acid and precipitated product was isolated by filtration, and its identity was confirmed by 1H NMR and mass spectroscopy. A preliminary assessment of catalyst recovery shown to be 70–80% in the organic layer of each cycle (atomic absorption spectrometry) and about 15–20% catalyst leaching to the aqueous layer in each cycle.
Table 1. Recycling of homogeneous catalyst in Suzuki reaction with basic work up ().
The above promising results encouraged us to look for the recycling capabilities of other homogeneous Pd catalysts under similar reaction conditions. We then carried out the recycling experiments with commonly used catalysts 2 (Pd-106) and 3 (Pd-118). The results were similar to 1 (Pd-100) indicating that these catalysts were also stable and retaining the activity under the aqueous basic work up conditions (, Entry 10–12, 19–21). Furthermore, to prove the generality of this recycling concept, Suzuki reactions between substituted phenylboronic acid (5 and 6) and 4-bromobenzoic acid (7) were carried under the above experimental conditions using 2 (Pd-106) and 3 (Pd-118) catalysts to yield 9b and 9c. In all the cases we could easily recycle the catalyst for two times without losing the activity of the catalysts (, Entry 13–18, 22–27). Furthermore, the concept was extended to Suzuki reaction between 2-bromobenzoic (8) acid and phenylboronic acid (4) using 3 (Pd-118) catalysts to yield 9d and it was successfully recycled consecutively for three times (, Entry 28–30). These results confirm the recyclability of 1 (Pd-100), 2 (Pd-106), and 3 (Pd-118) in Suzuki reaction under aqueous basic workup conditions.
Encouraged by the above results we then thought to explore the recyclability of these catalysts under acidic workup conditions. The Suzuki reaction between 4-bromoaniline (10) and 2-fluorophenylboronic acid (5) was then selected for our study. The Suzuki reaction was performed again in 2-MeTHF–water solvent medium using 3 (Pd-118) catalyst under similar conditions. The reaction was completed in 1 h with 98% conversion to product 12a (, Entry 1). After the completion of the reaction the reaction mass was then acidified to pH 1–2 with dilute HCl. The product in the form hydrochloride salt was thus extracted into aqueous solution leaving behind the catalyst in organic layer. The biphasic solution was then separated; 2-MeTHF layer containing the 3 (Pd-118) catalyst was taken for the next Suzuki reaction. Interestingly, the reaction occurred well in 1 h with 98% product (, Entry 2). Employing the similar workup procedure the catalyst was taken for one more cycle. These results clearly indicated that 3 (Pd-118) preserves its catalytic activity even under acidic workup conditions. With this encouraging result, the same transformation was then carried using 1 (Pd-100). Though recycling of the catalyst was successful, only moderate conversion was observed in all the cycles (, Entry 4–6). This could be due to low reactivity of the catalyst for the given conditions. As some un-reacted aniline was always retained in the organic layer, conversion to the product in the subsequent cycles appeared to be lower when comparing to the starting material.
Table 2. Recycling of homogeneous catalyst in Suzuki reaction with acidic work up ()
To test the generality of the recycling of Pd catalyst in Suzuki reaction under acidic work up conditions, Suzuki reactions were carried out between 2-Bromo 5-amino pyrazine (11) and 2-fluorophenylboronic acid (5) using 1 (Pd-100) and 3 (Pd-118) catalyst to give 12b. Moderate to good conversion was obtained in these experiments (, Entry 7–12).
To summarize, we have demonstrated a simple and efficient method for the recycling of homogeneous catalyst in Suzuki coupling reactions. From this study it is very clear that homogeneous catalysts 1 (Pd-100), 2 (Pd-106), and 3 (Pd-118) are quite stable under both acidic and basic workup conditions and can be recycled through simple manipulation of work up conditions. A major advantage of this technique is that, in a single operation, product, and catalyst can be separated easily, and the solution having the catalyst can be recycled without further isolation of the catalyst or loss in their activity. This method could be easily applied in the industry for the commercial scale manufacturing process, which could reduce the cost, operations, and environmental impacts and would increase the efficiency of manufacturing process. This strategy could be extended with varieties of homogeneous catalyst for other chemical transformation. To best of our knowledge, this is the first report where homogeneous catalyst has been recycled successfully in both acidic and basic conditions. Further study on Pd-based homogeneous catalyst and its optimum usage or application on large scale is under way.
Experimental
General procedure for the recycling catalyst in basic work up
A 25 mL three-neck round bottom flask was equipped with condenser, N2-inlet was charged 2-MeTHF (5 mL) and water (5 mL), then degassed through with N2 bubbling for about 5 min. To this, 2-methoxy phenyl boronic acid 6 (0.47 g; 0.0031 mmol), potassium carbonate (1.03 g, 0.0075 mmol), 4-bromobenzoic acid 7 (0.50 g, 0.0025 mmol), and then Pd catalyst (0.0085 g, 5 mol%) were added. The reaction mass was heated on an oil bath at 55–60°C and progress was monitored by HPLC. After the desired conversion, it was cooled to room temperature and biphasic solution was separated. To the organic layer, reactants and degassed solution of aqueous potassium carbonate was added, and then the reaction was carried under same conditions. The same procedure had been utilized for the recycling experiments and all the operations were performed under nitrogen atmosphere. For product isolation, aqueous layer was acidified with dilute HCl and precipitated product was filtered and dried to give the desired product. Identity of the product confirmed by 1H NMR and mass spectroscopy.
General procedure for the recycling of catalyst in acidic work up
A 25 mL three-neck round bottom flask fitted with condenser and N2-inlet was charged 2-MeTHF (5 mL) and water (5 mL) then degassed with N2 bubbling for about 5 min. To this, 4-bromoaniline 10 (0.50 g, 0.0029 mmol), 2-fluorophenyl boronic acid 5 (0.42 g, 0.0031 mmol), potassium carbonate (0.68 g, 0.0043 mmol), and then Pd catalyst (0.0085 g, 5 mol%) were added. Reaction mass was heated to reflux on oil bath and progress was monitored by HPLC. After the desired conversion, it was cooled to room temperature. Reaction mass was acidified with dilute HCl to pH 1–2, stirred for 10–15 min and biphasic solution was separated. To the organic layer, reactants and degassed solution of aqueous potassium carbonate was added, and then the reaction was carried under same conditions. The same procedure was followed for three times and all the operations were performed under nitrogen atmosphere.
Acknowledgements
We thank Senior Management of Pharmaceutical Development for the extensive support on this work. We also thank Bill Moss, Murugan Andiappan for supporting in the manuscript preparation, Jayan R, Alban D, Santosh P for analytical support and Swathi T for initial work support.
References
- Anastas , P. T. ; Warner , J. C. Green Chemistry: Theory and Practice ; Oxford University Press : Oxford , 1998 .
- Anastas , P. T. ; Zimmerman , J. B. Environ. Sci. Technol . 2003 , 37 , 94A .
- Anastas , P. T. ; Beach , E. S. Green Chem. Lett. Rev . 2008 , 1 , 9 .
- de Meijere , A. ; Diederich , F. .; Metal-Catalyzed Cross-Coupling Reactions , 2 ; Wiley-VCH : Weinheim , 2004 .
- Beller , M. ; Bolm , C. Transition Metals for Organic Synthesis , 2nd ed. ; Wiley-VCH : Weinheim , 2004 .
- Littke , A.F. ; Fu , G.C. Angew. Chem. Int. Ed . 2002 , 41 , 4176 .
- Christmann , U. ; Vilar , R. Angew. Chem. Int. Ed . 2005 , 44 , 366 .
- Nicolaou , K.C. ; Bulger , P.G. ; Sarlah , D. Angew. Chem. Int. Ed . 2005 , 44 , 4442 .
- Kantchev , E.A.B. ; Brien , C.J. ; Organ , O.M.G. . Angew. Chem. Int. Ed . 2007 , 46 , 2768 .
- Martin , R. ; Buchwald , S.L. Acc. Chem. Res . 2008 , 41 , 1461 .
- Fu , G.C. Acc. Chem. Res . 2008 , 41 , 1555 .
- Anderson , K.W. ; Buchwald , S.L. Angew. Chem. Int. Ed . 2005 , 44 , 6173
- Saito , B. ; Fu , G.C. J. Amer. Chem. Soc . 2007 , 129 , 9602 .
- Diebolt , O. ; Braunstein , P. ; Nolan , S.P. ; Cazin , C.S. Chem. Commun . 2008 , 3190 .
- Naber J.R. ; Buchwald , S.L. Adv. Synth. Catal . 2008 , 350 , 957 .
- Billingsley , K.L. ; Buchwald , S.L. Angew. Chem. Int. Ed . 2008 , 47 , 4695 .
- Lee , D.-H. ; Jung , J.-Y. ; Lee , I.M. ; Jin , M.-J. Eur. J. Org. Chem . 2008 , 73 , 356 .
- Garrett , C.E. ; Prasad , K. Adv. Synth. Catal . 2004 , 346 , 889 .
- Welch , C.J. ; Albaneze-Walker , J. ; Leonard , W.R. ; Biba , M. ; DaSilva , J. ; Henderson , D. ; Laing , B. ; Mathre , D.J. ; Spencer , S. ; Bu , X. ; Wang , T. Org. Process Res. Dev . 2005 , 9 , 198 .
- Guino , M. ; Hii , K.K.M. Chem. Soc. Rev . 2007 , 36 , 608 .
- Yang , Y.-C. ; Luh , T.-Y. J. Org. Chem . 2003 , 68 , 9870 .
- Parrish , C. A. ; Buchwald , S. L. J. Org. Chem . 2001 , 66 , 3820 .
- Jin , M.-J. ; Lee , D.-H. Angew. Chem . 2010 , 122 , 1137 ; Jin, M.-J.; Lee, D.-H. Angew. Chem. Int. Ed. 2010, 49, 1119 .
- Wittmann , S. ; Schatz , A. ; Stark , R.N.W.J. ; Reiser , O. Angew. Chem. Int. Ed . 2010 , 49 , 1867 .
- Janssen , M. ; Muller , C. ; Vogt , D. Green Chem . 2011 , 13 , 2247 .
- Lu , J. ; Lazzaroni , M.J. ; Hellett , J.P. ; Bommarius , A.S. ; Liotta , C.L. ; Eckert , C.A. Ind. Eng. Chem. Res . 2004 , 43 , 1586 .
- Mathews , C.J. ; Smith , P.J. ; Welton , T. Chem. Commun . 2000 , 14 , 1249 .
- Nobre , S.M. ; Wolke , S.I. ; da Rosa , R.G. ; Monteiro , A.L. Tetrahedron Lett . 2004 , 45 , 6527 .
- Fukuyama , T. ; Arai , M. ; Matsubara , H. ; Ryu , I. J. Org. Chem . 2004 , 69 , 8105 .
- Dumrath , A. ; Lubbe , C. ; Neumann , H. ; Jacksteall , R. ; Beller , M. Chem Eur. J . 2011 , 17 , 9599 .
Supporting information 1H, 13CNMR spectral data, HPLC chromatogram and Mass data for compounds 9a-c are available.
General information
All the reagents and solvents were commercial grade, and used without purification. Reactions were monitored by HPLC,% conversion to the product was quantified, respectively, to starting material. Compounds 9a–c ( ) were isolated as given in general procedure and characterized by 1H, 13C NMR, and mass spectrometer. Compounds 9d, 12a, and 12b were identified by mass using LC–MS. NMR was recorded on a 400 MHz Bruker instrument using d6-DMSO as solvent. Chemical shifts (δ) are expressed in parts per million (ppm) coupling constants (J) are in Hz. The 1 H and 13C NMR chemical shifts are reported relative to the resonance of the residual protons of the solvent was used as internal standard for 1 H (δ=2.50 DMSO-d6) and all deuterium solvent signals for 13C (δ=39.5 DMSO-d6). All measurements were carried out at 298 K. Abbreviations used in the description of NMR data are as follows: br, broad; s, singlet; d, doublet; t, triplet; m, multiplet. Purity of the product was recorded on Agilent 1200 series HPLC. C18 column was used with linear gradient acetonitrile and water system. LC–MS analyses were recorded on a Waters LC–MS equipped with a Waters X-Terra MS, C8-column, (3.5 µm, 100 mm×3.0 mm i.d.). The MS was equipped with an electrospray ion source operated in a negative ion mode. The capillary voltage was 3 kV and the MS was typically scanned between m/z 100 and 700. Palladium content was analyzed using atomic absorption method using Perkin Elmer AAnalyst 700.
1H, 13C, and mass spectral data
Biphenyl-4-carboxylic acid (9a)
1 H NMR (400 MHz, d 6 -DMSO): δ=8.07 (d, J=8.5 Hz, 2H), 7.84 (d, J=8.5 Hz, 2H), 7.78 (d, J=7.6 Hz, 2H), 7.55 (t, J=7.0 Hz, 2H), 7.47 (t, J=7.0 Hz, 1H).
13 C NMR (400 MHz, d 6 -DMSO): 167.1, 144.2, 139.0, 129.9, 129.7, 129.0, 128.2, 126.9, 126.8.
MS (EI): m/z=197(M–1).
2′-Flurobiphenyl-4-carboxylic acid (9b)
1 H NMR (400 MHz, d 6 -DMSO): δ=8.04 (d, J=6.4 Hz, 2H), 7.68 (d, J=8.5 Hz, 2H), 7.59 (t, J=7.5, 1H), 7.47 (t, J=8.5 Hz, 1H), 7.35 (m, 2H).
13 C NMR (400 MHz, d 6 -DMSO): 166.9, 157.8, 139.3, 131.6, 131.2, 130.6, 130.0, 129.5, 129.2, 125.1, 116.3, 116.0.
MS (EI): m/z=215(M–1)
2′-Methoxybiphenyl-4-carboxylic acid (9c)
1 H NMR (400 MHz, d 6 -DMSO): δ=12.97, (s, 1H), 7.97 (d, J=8.5 Hz, 2H), 7.60 (d, J=8.0 Hz, 2H), 7.39 (t, J=7.5 Hz, 1H), 7.34 (d, J = 7.5 Hz, 1H), 7.14 (d, J=8.2 Hz, 1H), 7.06 (t, J=7.0 Hz, 1H) 3.77 (s, 3H).
MS (EI): m/z=227(M–1)
1H, 13C NMR spectra, and HPLC chromatogram for 9a–c
Figure 4. 1H NMR spectra of compound 9b (Note: peak at 1.18 (t), 1.92 (s), and 4.03 (q) ppm corresponds to residual ethylacetate).
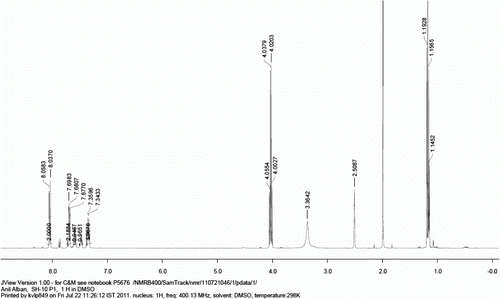
Figure 5. 13C NMR spectrum of compound 9b (Note: peak at 14.0, 20.7, and 170.3 ppm are corresponds to residual ethylacetate).
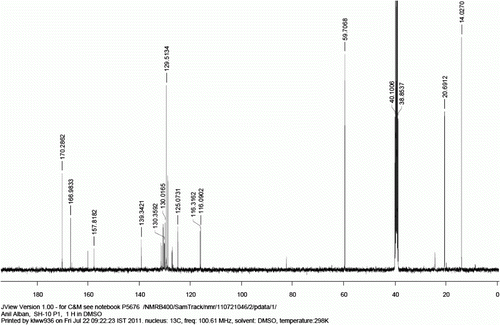