ABSTRACT
In this research, 2,3-dichloro-5,6-dicyano-1,4-benzoquinone (DDQ) has been used in the condensation reaction between o-phenylenediamine and various arylaldehydes as oxidants under microwave irradiation. In this clean reaction, the benzimidazole derivatives were synthesized as benefit target products. The advantages to this procedure include high yields, easy work-up procedure, no chromatographic separation, and short reaction times. The obtained pure products were characterized by physical and spectral data such as IR, 1H NMR and 13C NMR techniques.
GRAPHICAL ABSTRACT

Introduction
Benzimidazole and its derivatives exhibit a widespread spectrum of pharmacological activities. They have been used for antitumor ( Citation1), antivirus ( Citation2), antiallergic ( Citation3), antimicrobial ( Citation4), antihypertensive ( Citation5), topoisomerase I inhibitor ( Citation6), and angiotensin II inhibitor ( Citation7) applications. Several compounds from this class have been applied as ligands for asymmetric catalysis ( Citation8) and they are very important intermediates in organic reactions ( Citation9).
There are many protocols for benzimidazole synthesis through a general procedure including condensation of o-phenylenediamine with aldehydes under oxidative conditions ( Citation10–17). Various oxidative agents such as 1,4-benzoquinone ( Citation18), MnO2 ( Citation19), H2O2/HCl ( Citation20), benzofuroxane ( Citation21), 2,3-dichloro-5,6-dicyano-1,4-benzoquinone (DDQ) ( Citation22), ceric (IV) ammonium nitrate (CAN) ( Citation23), NaHSO3 ( Citation24), and polymer-supported hypervalent iodine ( Citation25) have been applied. On the other hand, it has been reported that the redox system can be used as the catalyst for the preparation of benzimidazoles. However, many of the reported synthetic protocols suffer from disadvantages such as needing anhydrous conditions, harsh reaction conditions, metals and expensive reagents, prolonged reaction times, low yield, etc. In recent years, the microwave as heating source has been applied for the rapid synthesis of a variety of heterocyclic compounds both in solution phase and solvent-free conditions ( Citation26).
Many articles have been published in the area of microwave-assisted organic synthesis ( Citation27, Citation28). In many cases, controlled microwave heating under sealed vessel conditions has dramatically reduced reaction times, increased product yields, and enhanced product purities by reducing unwanted side reactions observed in conventional synthetic methods ( Citation27, Citation28).
We have reported the synthesis of a series of 2-substituted benzimidazoles using DDQ as the oxidizing agent at room temperature ( Citation29). In order to reduce reaction time and increase product yield, we have been interested in the preparation of benzimidazole derivatives with DDQ under microwave conditions. Herein, we hope to report a simple, chemoselective, one-pot, and high yield synthesis of 2-substituted benzimidazoles directly from o-phenylenediamine and arylaldehydes using DDQ as the oxidant agent under microwave irradiation.
Results and discussion
In order to study the condensation reaction, we accomplished the reaction between o-phenylenediamine and various arylaldehydes using DDQ as the oxidant in acetonitrile solvent under microwave irradiation to synthesize the benzimidazole derivatives (Scheme 1).
First, the effect of various powers of microwave irradiation was investigated for the synthesis of 4-chlorobenzimidazole as a model reaction in order to optimize the best suited reaction conditions. It was observed that the reaction in the presence of DDQ as the oxidant agent and microwave irradiation with power 100 W afforded the best result with 94% isolated product yield during 4.1 min (, entry 2).
Table 1. Survey the microwave power used for the reaction.a.
Next, the amount of oxidative agent was investigated. It was found that 60 mol% of DDQ oxidant was sufficient to give maximum yields of the required product (, entry 4). In the absence of the oxidative agent, the reaction was not accomplished after 25 min under microwave irradiation (, entry 6).
Table 2. Optimizing the value of oxidative agent for the preparation of 2-(4-chlorophenyl)-1H-benzimidazolea.
In addition, to study the effect of solvent on the rate of the reaction, we carried out the model reaction with various solvents such as ethanol, methanol, acetonitrile, ethyl acetate, and dichloromethane. It was found that the solvent has a distinguished influence on the yield of product (). As seen from , the acetonitrile is a convenient solvent with 94% yield for the reaction.
Table 3. Optimization of various solvents for the preparation of 2-(4-chlorophenyl)-1H-benzimidazole.a
A comparison of the present work with different reported works using various catalysts ( Citation10–13, Citation29–31) in terms of reaction times and the yields of respective products is given in . As seen in , our procedure produced the benzimidazole derivatives with shorter reaction times, higher yield percentage of products, and easy work-up (, entry 1 vs. entries 2–9).
Table 4. Comparison of DDQ activity with other reported oxidative agent and catalysts activities.
Although these previously reported works have their own advantages, most suffer from disadvantages such as acidic catalysts, toxic and pollutants metal catalysts, high reaction temperatures, reflux conditions, prolonged reaction times, lower product yields, and tedious work-up procedures. For instance, in one of the mentioned methods, the reaction was performed in the air/dioxane/100°C condition, which is potentially explosive (, entry 2). In contrast, the present method has valuable advantages such as safe methods, mild reaction conditions, simple work-up procedure, no side reactions, available and cheap oxidant, short reaction times, high yields of products, and using metal-free oxidant agent.
A proposed mechanism for the synthesis of 2-substituted benzimidazole derivatives using DDQ as the oxidant agent is represented (Scheme 2). First, condensation of o-phenylenediamine and arylaldehydes led to the formation of unstable intermediate benzimidazoline with the removal of one H2O molecule and the ring closured by attachment of a nitrogen atom lone pair. Then, two hydrogen atoms were removed in the presence of DDQ as the oxidant agent. The DDQ was reduced to 1,4-hydroquinone; finally, 2-substituted benzimidazole derivatives were prepared (Scheme 2).
Scheme 2. Proposed mechanism for synthesis of 2-substituted benzimidazole derivatives using DDQ as oxidant agent.
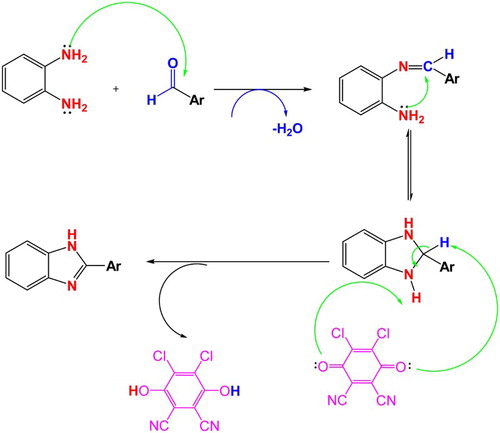
Finally, to determine the effect of various substituents and the limitations of the present reaction, several aromatic aldehydes were reacted with o-phenylenediamine in the presence of DDQ as the oxidant system under microwave irradiation in acetonitrile solvent. The corresponding products including 2-substituted benzimidazole derivatives were obtained and the results are summarized in . The best results were prepared with electron-withdrawing substituents on the aromatic ring. Also, the reaction occurred using propionaldehyde as an aliphatic aldehyde, but was not the observed product under both heating and microwave conditions. The results are reported in , entry 15.
Table 5. Reaction of various aryl aldehydes with o-phenylenediamine.
Using microwave irradiation as the heating source, the rates of reactions involving polar components are usually very fast. Reactions that require hours or even days by conventional heating may often be accomplished in seconds by microwave irradiation, and that is the reason why this technology is widely applied to drug discovery. For this reason, the reaction of the various aldehydes with o-phenylenediamine was speeded up by microwave irradiation in comparison with this reaction under thermal condition as shown in .
The benzimidazoles were prepared in excellent yields and short reaction times under microwave irradiation, while low yields and long reaction times were observed under traditional heating conditions ( Citation29). Thus, this procedure is a practical process compared to previously reported methods for the preparation of 2-substituted benzimidazoles ( Citation10–13).
Experimental
Materials and apparatus
All the materials were of commercial reagent grade. The aromatic aldehydes and o-phenylenediamine were purified by standard procedures and the purity was determined by thin-layer chromatography (TLC). IR spectra were recorded as KBr pellets on a Perkin-Elmer 781 spectrophotometer and an Impact 400 Nicolet FT-IR spectrophotometer. 1H NMR and 13C NMR were recorded in DMSO-d6 and CDCl3 solvents on a Bruker DRX-400 spectrometer with tetramethylsilane as the internal reference. Microwave irradiations were carried out in a microwave oven specially designed for the organic synthesis (Milestone LAVIS 1000 Basic Microwave). Melting points obtained with a Yanagimoto micro melting point apparatus are uncorrected. The purity determination of the substrates and reaction monitoring were accomplished by TLC on silica-gel polygram SILG/UV 254 plates (from Merck Company).
General procedure for the synthesis of benzimidazoles from o-phenylenediamine
A solution of o-phenylenediamine (0.11 g, 1 mmol) and aldehyde (1.0 mmol) in a minimum amount of acetonitrile was prepared. DDQ (0.14 g, 60 mol %) was added into an open Erlenmeyer flask. The mixture was irradiated in a microwave oven. The progress of the reaction was monitored by TLC. After completion of the reaction, the reaction mixture was added drop wise into a mixture of water and ice, and the crude solid was filtered off and washed with water. The pure products were obtained by recrystallization from methanol/H2O (1:1) and were identified by physical and spectroscopic data.
2-(4-Cholorophenyl)-benzimidazole (3j): Yellow solid, m.p. = 290–292°C (lit. 291–293°C) ( Citation37); IR (KBr)/ν (cm−1): 3442, 1598, 1580, 1429; 1H NMR (DMSO-d6, 400 MHz)/δ ppm: 7.2 (m, 2H, ArH), 7.50–7.89 (m, 6H, ArH), 13 (s, 1H, NH); 13C NMR (DMSO-d6, 100 MHz)/δ ppm: 113.5, 123.7, 127.6, 128.3, 129.4, 133.4, 138.9, 151.8.
Conclusion
We have reported an efficient method for the synthesis of 2-substituted benzimidazole derivatives under microwave irradiation. By using the microwave oven as a heating source, reaction times were reduced and product yields were increased compared to thermal conditions. The advantages of the present method include simple procedure, easy work-up, short reaction time, easy purification, mild reaction conditions, and excellent yields.
Supplement_Material.pdf
Download PDF (1.3 MB)Disclosure statement
No potential conflict of interest was reported by the authors.
Notes on contributors
Hossein Naeimi was received his M.Sc. degree in 1991 from Shahid Beheshti University and Ph.D. degree in 1998 from Shiraz University. After working at the Government College, he became Assistant Professor at the University of Kashan and subsequently became Associate Professor in 2004 and Professor in 2008.
Zahra Babaei received her B.Sc. degree in 2013 from Shahid Chamran University of Ahvaz and M.Sc. degree in 2015 from the University of Kashan. Now, she is a Ph.D. student at the Isfahan University of Technology.
Additional information
Funding
References
- Denny, W.A.; Rewcastle, G.W.; Baguley, B.C. J. Med. Chem. 1990, 33, 814–819. doi: 10.1021/jm00164a054
- (a) Porcari, A.R.; Devivar, R.V.; Kucera, L.S.; Drach, J.C.; Townsend, L.B. J. Med. Chem. 1998, 41, 1252–1262. (b) Roth, M.; Morningstar, M.L.; Boyer, P.L.; Hughes, S.H.; Buckheit, R.W., Michejda, C.J. J. Med. Chem. 1997, 40, 4199–4207. doi: 10.1021/jm970559i
- Nakano, H.; Inoue, T.; Kawasaki, N.; Miyataka, H.; Matsumoto, H.; Taguchi, T.; Inagaki, N.; Nagai, H.; Satoh, T. Chem. Pharm. Bull. 1999, 47, 1573–1578. doi: 10.1248/cpb.47.1573
- Fonseca, T.; Gigante, B.; Gilchrist, T.L. Tetrahedron. 2001, 57, 1793–1799. doi: 10.1016/S0040-4020(00)01158-3
- Serafin, B.; Borkowska, G.; Główczyk, J.; Kowalska, I.; Rump, S. Polish J. Pharmacol. Pharm. 1989, 41, 89–96.
- Kim, J.S.; Gatto, B.; Yu, C.; Liu, A.; Liu, L.F.; LaVoie, E. J. Med. Chem. 1996, 39, 992–998. doi: 10.1021/jm950412w
- Kohara, Y.; Kubo, K.; Imamiya, E.; Wada, T.; Inada, Y.; Naka, T. J. Med. Chem. 1996, 39, 5228–5235. doi: 10.1021/jm960547h
- Figge, A.; Altenbach, H.J.; Brauer, D.J.; Tielmann, P. Tetrahedron: Asymmetry. 2002, 13, 137–144. doi: 10.1016/S0957-4166(02)00079-4
- (a) Bai, Y.; Lu, J.; Shi, Z.; Yang, B. Synlett. 2001, 4, 544–546. (b) Hasegawa, E.; Yoneoka, A.; Suzuki, K.; Kato, T.; Kitazume, T.; Yangi, K. Tetrahedron, 1999, 55, 12957–12968. doi: 10.1055/s-2001-12339
- Lin, S.; Yang, L. Tetrahedron Lett. 2005, 46, 4315–4319. doi: 10.1016/j.tetlet.2005.04.101
- Azizian, J.; Torabi, P.; Noei, J. Tetrahedron Lett. 2016, 57, 185–188. doi: 10.1016/j.tetlet.2015.11.092
- Ghosh, P.; Subba, R. Tetrahedron Lett. 2015, 56, 2691–2694. doi: 10.1016/j.tetlet.2015.04.001
- Sharghi, H.; Asemani, O.; Tabaei, S.M.H. J. Heterocycl. Chem. 2008, 45, 1293–1298. doi: 10.1002/jhet.5570450506
- Marri, M.R.; Peraka, S.; Macharla, A.K.; Mameda, N.; Kodumuri, S.; Nama, N. Tetrahedron Lett. 2014, 55, 6520–6525. doi: 10.1016/j.tetlet.2014.09.081
- Sun, Q.; Wang, C.J.; Gong, S.S.; Ai, Y.J.; Sun, H.B. Chin. Chem. Lett. 2015, 26, 297–300. doi: 10.1016/j.cclet.2014.11.014
- Paudel, S.; Cao, Y.; Guo, S.; An, B.; Kim, K.M., Cheon, S.H. Bioorg. Med. Chem. 2015, 23, 6418–6426. doi: 10.1016/j.bmc.2015.08.022
- Chaudhari, C.; Hakim Siddiki, S.M.A.; Shimizu, K.I. Tetrahedron Lett. 2015, 56, 4885–4888. doi: 10.1016/j.tetlet.2015.06.073
- (a) Verner, E.; Katz, B.A.; Spencer, J.R.; Allen, D.; Hataye, J.; Hruzewicz, W.; Hui, H.C.; Kolesnikov, A.; Li, Y.; Luong, C.; Martelli, A.; Radika, K.; Rai, R.; She, M.; Shrader, W.; Sprengeler, P. A.; Trapp, S.; Wang, J.; Young, W.B.; Mackman, R.L. J. Med. Chem. 2001, 44, 2753–2771. (b) Kumar, S.; Kansal, V.; Bhaduri, A. Indian J. Chem. B, 1991, 20, 254–261. doi: 10.1021/jm0100638
- Bhatnagar, I.; George, M.V. Tetrahedron. 1968, 24, 1293–1298. doi: 10.1016/0040-4020(68)88080-9
- Bahrami, K.; Khodaei, M.M.; Kavianinia, I. Synthesis. 2007, 4, 547–550. doi: 10.1055/s-2007-965878
- Patzold, F.; Zeuner, F.; Heyer, T.H.; Niclas, H. J. Synth. Commun. 1992, 22, 281–288. doi: 10.1080/00397919208021304
- Eynde, J.J.V.; Delfosse, F.; Lor, P.; Harverbeke, Y.V. Tetrahedron. 1995, 51, 5813–5818. doi: 10.1016/0040-4020(95)00252-4
- Kumar, R.; Joshi, Y.C. E-J. Chem. 2007, 4, 606–610. doi: 10.1155/2007/756267
- (a) Weidner-Wells, M.A.; Ohemeng, K.A.; Nguyen, V.N.; Fraga-Spano, S.; Macielag, M.J.; Werblood, H.M.; Foleno, B.D.; Webb, G.C.; Barrett, J.F.; Hlasta, D.J. Bioorg. Med. Chem. Lett. 2001, 11, 1545–1548. (b) Austen, S.C.; Kane, J.M. J. Heterocycl. Chem. 2001, 38, 979–980. doi: 10.1016/S0960-894X(01)00024-5
- Kumar, A.; Maurya, R.A.; Ahmad, P. J. Comb. Chem. 2009, 11, 198–201. doi: 10.1021/cc8001876
- Mavandadi, F.; Lidström, P. Curr. Top. Med. Chem. 2004, 4, 773–792. doi: 10.2174/1568026043451078
- (a) Kappe, C.O. Angew. Chem. Int. Ed. 2004, 43, 6250–6284. (b) Hayes, B. L. Aldrichim. Acta 2004, 37, 66–77. doi: 10.1002/anie.200400655
- (a) De La Hoz, A.; Diaz-Ortiz, A.; Moreno, A. Chem. Soc. Rev. 2005, 34, 164–178. (b) Perreux, L.; Loupy, A. Tetrahedron. 2001, 57, 9199–9223. (c) Kuhnert, N. Angew. Chem. Int. Ed. 2002, 41, 1863–1866. (d) Strauss, C.R. Angew. Chem. Int. Ed. 2002, 41, 3589–3590. doi: 10.1039/B411438H
- Naeimi, H.; Babaei, Z. J. Chin. Chem. Soc. 2015, 62, 41–46. doi: 10.1002/jccs.201400293
- Bai, G.; Lan, X.; Liu, X.; Liu, C.; Shi, L.; Chen, Q.; Chen, G. Green Chem. 2014, 16, 3160–3168. doi: 10.1039/C3GC42551G
- Jang, S.S.; Youn, S. W. Org. Biomol. Chem. 2016, 14, 2200–2204. doi: 10.1039/C6OB00074F
- Bachhav, H.M.; Bagat, S.B.; Telvekar, V.N. Tetrahedron Lett. 2011, 52, 5697–5701. doi: 10.1016/j.tetlet.2011.08.105
- Han, X.M.; Ma, H.Q.; Wang, Y.L. Arkivoc, 2007, 13, 150–154.
- Navarrete-Vazquez, G.; Moreno-Diaz, H.; Aguirre-Crespo, F.; Leon-Rivera, I.; Villalobs-Molina, R.; Munoz-Munizd, O.; Estrada-Sotoa, S. Bioorg. Med. Chem. Lett. 2006, 16, 4169–4173. doi: 10.1016/j.bmcl.2006.05.082
- Nagawade, R.R.; Shinde, D.B. Chin. Chem. Lett. 2006, 17, 453–456.
- Chari, M.A.; Shobha, D.; Sasaki, T. Tetrahedron Lett. 2011, 52, 5575–5580. doi: 10.1016/j.tetlet.2011.08.047
- Tavman, A.; Birteksoz, A.S.; Oksuzomer, F.S. Afr. J. Chem. 2012, 65, 150–158.
- Behbahani, F.K.; Ziaei, P. Chem. Heterocycl. Compd. 2012, 48, 1011–1017. doi: 10.1007/s10593-012-1093-0