ABSTRACT
A series of complex 2,2,2-trifluoroethyl fatty acid esters were synthesized by the reaction of fatty acids and phenyl(2,2,2-trifluoroethyl)iodonium triflate in the presence of Cs2CO3. The reaction at room temperature without any other additive provided the corresponding 2,2,2-trifluoroethyl esters in up to quantitative yield. The conjugated carbon–carbon double bonds, the unprotected hydroxyl group, and the amido functionalities in the substrates are all tolerated in the reaction. Compared to the known synthetic methods, advantages of this reaction include mild conditions, good functional group tolerance, and without using harsh and toxic condensation agents, which allows for a green, facile, and efficient way to 2,2,2-trifluoroethyl fatty acid esters.
GRAPHICAL ABSTRACT
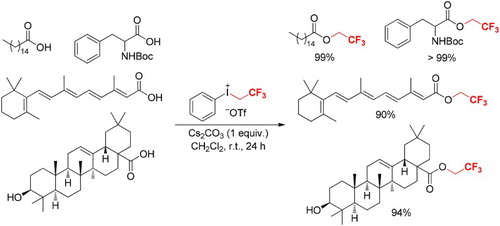
Introduction
Fatty acid esters are in widespread use ranging from fundamental research to industrial applications (Citation1). The chemical and physical properties of fatty acid esters dramatically depend upon the types of fatty acids and alcohols (Citation2–4). Alkyl (in most cases methyl) esters of long-chain fatty acids derived from vegetable oils or animal fats have been explored as biodegradable, nontoxic, and renewable biodiesels (Citation2). Sugar fatty acid esters prepared from carbohydrates (e.g. glucose and sucrose) and fatty acids (e.g. lauric and palmitic acids) have been applied as nonionic green biosurfactants in food, cosmetic, and pharmaceutical industries (Citation3). Polyglycerol, sucrose, and steroid fatty acid esters have shown antioxidative or antibacterial activities (Citation4). 2,2,2-Trifluoroethyl aliphatic carboxylates have been used as versatile acylation reagents in reactions, including transesterification, amidation, and kinetic resolution of aliphatic amines (Citation5). The studies on the Langmuir monolayers of behenic acid 2,2,2-trifluoroethyl ester at air–water interface have demonstrated that the hydrophilic CF3 head of the long-chain fatty acid 2,2,2-trifluoroethyl ester can strikingly change the electrostatic properties of the monolayer (Citation6). Moreover, unsaturated fatty acid 2,2,2-trifluoroethyl esters such as 2,2,2-trifluoroethyl methacrylate, 2,2,2-trifluoroethyl undec-10-enoate, and 2,2,2-trifluoroethyl 4-pentenoate have been exploited as reliable polymerization monomers preparing anion-exchange membranes and drug delivery agents (Citation7). The short- to medium-chain fatty acid 2,2,2-trifluoroethyl esters have been investigated as co-solvents for carbonate-based electrolyte of lithium ion batteries (Citation8a).
Thanks to the usefulness of 2,2,2-trifluoroethyl fatty acid esters, the development of an efficient method for the synthesis of these compounds is of great significance (Citation1,Citation8–11). Although production of non-fluorinated aliphatic carboxylates has been well documented (Citation1), the approaches to the synthesis of 2,2,2-trifluoroethyl esters have far not satisfied the needs of application (Citation8–11). The known means to the preparation of 2,2,2-trifluoroethyl fatty acid esters were mainly focused on the reactions between 2,2,2-trifluoroethanol and carboxylic acids or their derivatives such as carboxylic halides and anhydrides in the presence of appropriate catalysts (Citation8–11). These methods, however, suffered from harsh reaction conditions, narrow functional group tolerance, and poor efficiency, owing to the weak nucleophilicity of 2,2,2-trifluoroethanol and the indispensability of strong condensation agents or catalysts. Furthermore, the diversity of long-chain fatty acid 2,2,2-trifluoroethyl esters is rarely developed compared to the short- and medium-chain analogues (Citation8–11), which might be because of the poorer reactivity of long-chain fatty acids than the short- and medium-chain congeners in acylation reactions. In addition, it should be mentioned that the highly active 2,2,2-trifluorodiazoethane reacted with carboxylic acids could not expectedly form the corresponding 2,2,2-trifluoroethyl esters, making matters worse (Citation12).
On the other hand, phenyl(2,2,2-trifluoroethyl)iodonium triflate ([PhICH2CF3][OTf]), first synthesized by Umemoto et al., has been proved to be an efficient trifluoroethylation reagent for heteroatom and carbon nucleophiles such as amines, peptides, alcohols, phenols, thiols, sulfides, thioglycosides, phosphines, carbanions, and silyl enol ethers. (Citation13). These reactions proceeded smoothly to form the crucial N-CH2CF3, O-CH2CF3, S-CH2CF3, P-CH2CF3, and C-CH2CF3 bonds in a variety of bioactive molecules, showing much more powerful CH2CF3 transfer ability of [PhICH2CF3][OTf] than CF3CH2X (X = I, OTf, OTs). Although the preliminary reaction of benzoic acid with [PhICH2CF3][OTf] has been notified, the elusive interaction between phenyl(2,2,2-trifluoroethyl)iodonium triflate and the long-chain aliphatic fatty acids, especially those containing sensitive functionalities, has never been known (Citation13b). We imagined whether the direct reaction of [PhICH2CF3][OTf] with functionalized fatty acids in the presence of an appropriate base would be a viable and convenient access to the synthesis of complex fatty acid 2,2,2-trifluoroethyl esters under mild conditions.
Results and discussion
Indeed, palmitic acid (or hexadecanoic acid, 1a), one of the most common saturated fatty acid in animals, plants, and microorganisms, reacted with phenyl(2,2,2-trifluoroethyl)iodonium triflate (1.5 equiv.) in CH2Cl2 in the presence of Cs2CO3 at room temperature for 24 h supplied 2a in 71% yield (entry 1, ). If 1a reacted with Cs2CO3 for 5 min followed by treatment with [PhICH2CF3][OTf] (1.5 equiv.) in CH2Cl2 at room temperature for 24 h, 2a was formed in 99% yield (entry 3, ). This implied that the use of base to first deprotonate 1a was beneficial for the reaction. Moreover, either prolonging or shortening the reaction time provided a comparable yield of 2a (entries 2 and 4, ). Reducing the amounts of [PhICH2CF3][OTf] from 1.5 to 1.2 or 1.1 equivalents brought down the yield of 2a (entries 5 and 6, ). K2CO3 and K3PO4 were also suitable bases for the trifluoroethylation, which gave 2a in good yields (entries 7 and 8, ). Reaction of 1a with [PhICH2CF3][OTf] and Na2CO3 or KOH provided 2a in 66% or 67% yield (entries 9 and 10, ). The weak bases such as K2HPO4 and NaHCO3 working with 1a and [PhICH2CF3][OTf] afforded trace or 15% of 2a (entries 11 and 12, ). When pyridine, NEt3, DABCO, and 2,4,6-trimethylpyridine (Citation13b) were employed instead of Cs2CO3 in the same reaction, 2a was formed in 9–38% yields (entries 13–16, ). Varying the reaction time of 1a, [PhICH2CF3][OTf], and 2,4,6-trimethylpyridine (sterically hindered) (Citation13b) from 24 h to 0.5 h led to 86% of 2a, whereas shortening the reaction time in the cases of pyridine, NEt3, and DABCO did not improve the yield of 2a. These results indicated that the highly soluble organic bases might bring about decay of [PhICH2CF3][OTf] and/or the final product (2a) during the reaction. Additionally, reaction of 1a with [PhICH2CF3][OTf] in the absence of base afforded 2a in only 19% yield (entry 17, ).
Table 1. 2,2,2-Trifluoroethylation of palmitic acid (1a) by [PhICH2CF3][OTf]a.
The choice of solvent had a big influence on the reaction. When the reaction was performed in CH3CN or THF under the standard conditions, 2a was obtained in 67% or 44% yield, respectively (entries 18 and 19, ). If the reaction was run in DMSO or DMF, none of the desired product was formed, probably due to the decomposition of [PhICH2CF3][OTf] in these solvents (entries 20 and 21, ). Notably, if 1a was treated with Cs2CO3 and [PhICH2CF3][OTf] in toluene or 1,4-dioxane under the standard conditions, 2a was synthesized in 93% or 89% yield (entries 22 and 23, ). The solvent-free reaction of 1a with Cs2CO3 and [PhICH2CF3][OTf] at room temperature for 24 h furnished 2a in 50% yield (entry 25, ). Mesityl(2,2,2-trifluoroethyl)iodonium triflate is also an available CF3CH2 transfer source in the reaction, which provided 2a in 98% yield under the standard conditions (entry 26, ).
With the optimized reaction conditions (1/Cs2CO3 (1 equiv)/5 min, then [PhICH2CF3][OTf] (1.5 equiv)/r.t./24 h) in hand, a variety of aliphatic carboxylic acids either with or without sensitive functional groups were investigated (). The long-chain saturated fatty acids such as lauric acid (1b), myristic acid (1c), stearic acid (1d), and behenic acid (1e) reacted with [PhICH2CF3][OTf] and Cs2CO3 in CH2Cl2 at room temperature for 24 h to give the corresponding esters (2b–e) in up to 99% yield. Similar reaction of oleic acid (1f) with [PhICH2CF3][OTf] under the standard conditions provided 2,2,2-trifluoroethyl oleate (2f) in 90% yield. Other unsaturated fatty acids such as linolenic acid (1g), (E)-oct-3-enoic acid (1h), 2-(cyclohex-1-en-1-yl)acetic acid (1i), sorbic acid (1j), and vitamin A acid (1k) reacted with [PhICH2CF3][OTf]/Cs2CO3 in CH2Cl2 at room temperature for 24 h afforded the desired products 2g–k in good yields. It seems that the carbon–carbon double bonds in fatty acids are tolerable in the reaction. Since products 2h and 2j are volatile, their 19F NMR yields were given to elucidate the availability of the reaction for these compounds. In addition, reactions of 2-(adamantan-1-yl)acetic acid (1l), 2-([1,1′-biphenyl]-4-yl)acetic acid (1m), and 2-(naphthalen-2-yl)acetic acid (1n) with [PhICH2CF3][OTf] and Cs2CO3 provided the corresponding 2,2,2-trifluoroethyl esters 2l-n in 92% to >99% yields. Treatment of 2-phenylacetic acid (1o) with [PhICH2CF3][OTf]/Cs2CO3 supplied 2o in 95% 19F NMR yield. Succinic acid (1p) reacted with [PhICH2CF3][OTf]/Cs2CO3 under the standard conditions to give the doubly trifluoroethylated ester (2p) in 80% 19F NMR yield. These results indicated that both long-chain and short-chain fatty acids could be smoothly converted in the reaction. Remarkably, (N-tert-butoxycarbonyl)-L-phenylalanine (1q) and N-tosyl-L-proline (1r) were also suitable substrates in the conversion, which furnished 2q in >99% yield and 2r in 89% yield, respectively. Nonetheless, the N-unprotected forms of 1q and 1r reacted with [PhICH2CF3][OTf]/Cs2CO3 did not give the desired corresponding trifluoroethyl esters. Furthermore, reaction of oleanolic acid (1s) with [PhICH2CF3][OTf]/Cs2CO3 under the standard conditions afforded 2s in 94% yields. These data indicated that the free aliphatic OH group is tolerated in the reaction (Citation13v).
Table 2. 2,2,2-Trifluoroethylation of various functionalized fatty acids by [PhICH2CF3][OTf] at room temperaturea.
At last, several traditional approaches were employed to synthesize 2,2,2-trifluoroethyl esters () (Citation14). Fatty acids such as 1a, 1k (with conjugated carbon–carbon double bonds), 1q (with N-Boc protection group), and 1s (with free hydroxyl group) were chosen as the model substrates to compare the efficiency of these methods. At the beginning, reaction of 1a with 2,2,2-trifluoroethanol in CH2Cl2 in the presence of 0.2 or 2 equiv. of concentrated H2SO4 at reflux for 24 h (Method A) provided 2a in 52% or 79% yield, respectively. When 1k, 1q, and 1s were treated with CF3CH2OH under the same conditions (Method A), however, no desired products were obtained. Another approach using DCC/DMAP as the condensation agents (Method B) was applied to esterification of 2,2,2-trifluoroethanol with 1a, 1k, 1q, and 1s, which afforded 2a in 82% yield, 2k in 75% yield, and 2q in 82% yield, and none of 2s was formed in the conversion. Reactions of 1a and 1k with (COCl)2 provided carboxylic chlorides, which without isolation reacted with CF3CH2OH (2 equiv.) in CH2Cl2 in the presence of NEt3 (3 equiv.) at room temperature for 2–3 h (Method C) to form 2a in 46% yield and 2k in 32% yield (over two steps). Nevertheless, substrates 1q and 1s failed to give 2q and 2s in the reaction by Method C. All these results combined indicated that the reaction described in is simpler, milder, more efficient, and showed better functional group tolerance, compared to the known ones.
Table 3. Synthesis of 2,2,2-trifluoroethyl fatty acid esters by the known methodsa.
Conclusions
In conclusion, we have developed a facile method to the construction of 2,2,2-trifluoroethyl esters from complex fatty acids and aryl(2,2,2-trifluoroethyl)iodonium triflate in the presence of Cs2CO3 at room temperature under mild conditions. The reaction provided a series of fatty acid 2,2,2-trifluoroethyl esters in high yields, which are promising building blocks in the preparation of functional materials and potentially bioactive molecules. The conjugated carbon–carbon double bonds, the free hydroxyl group, and the amido groups in the substrates were all tolerated in the reaction. This protocol supplies an efficient and green access to 2,2,2-trifluoroethyl fatty acid esters without using harsh, toxic, and moisture-sensitive condensation agents that are indispensable in the previous methods.
Experimental section
General
All reactions were carried out under ambient atmosphere. Unless otherwise specified, NMR spectra were recorded in CDCl3 on a 500 or 400 MHz (for 1H), 471 or 376 MHz (for 19F), or 126 or 100 MHz (for 13C) spectrometer. All chemical shifts were reported in ppm relative to TMS (0 ppm) for 1H NMR or PhCF3 (–63.5 ppm) for 19F NMR as an internal or external standard. Melting points of new compounds were measured and uncorrected. Phenyl- and mesityl(2,2,2-trifluoroethyl)iodonium triflates were synthesized according to the literature (Citation13). Boc-L-Phenylalanine (1q) was synthesized from L-phenylalanine according to the literature (Citation15a). Tos-L-Proline (1r) was synthesized from L-proline according to the literature (Citation15b). Other fatty acids and reagents were all purchased from commercial sources and used without further purification.
General procedure for trifluoroethylation of fatty acids by phenyl(2,2,2-trifluoroethyl)iodonium triflate.
A sealed tube was charged with fatty acid (1, 0.3 mmol), Cs2CO3 (97.7 mg, 0.30 mmol), and CH2Cl2 (1 mL) with vigorous stirring. After 5 min, [PhICH2CF3][OTf] (196.3 mg, 0.45 mmol) and CH2Cl2 (2 mL) were added. The mixture was reacted at room temperature for another 24 h. The solvent was removed under reduced pressure. The residue was purified by flash column chromatography on silica gel using petroleum ether (to remove iodobenzene) and/or a mixture of petroleum ether and ethyl acetate as eluents to give 2,2,2-trifluoroethyl fatty acid ester.
2,2,2-Trifluoroethyl palmitate (2a) (16a)
White solid; yield: 100.5 mg (99%). Petroleum ether as eluent for flash column chromatography. 1H NMR (400 MHz, CDCl3): δ 4.46 (q, J = 8.5 Hz, 2H), 2.41 (t, J = 7.9 Hz, 2H), 1.65 (m, 2H), 1.25 (m, 24H), 0.88 (t, J = 7.0 Hz, 3H). 19F NMR (376 MHz, CDCl3): δ –73.9 (t, J = 8.4 Hz, 3F). 13C NMR (126 MHz, CDCl3): δ 172.3, 123.2 (q, J = 277.2 Hz), 60.3 (q, J = 36.5 Hz), 33.8, 32.1, 29.8, 29.8, 29.8, 29.7, 29.6, 29.5, 29.3, 29.1, 24.9, 22.8, 14.3.
2,2,2-Trifluoroethyl dodecanoate (2b) (16b)
Light yellow oil; yield: 80.5 mg (95%). Petroleum ether as eluent for flash column chromatography. 1H NMR (500 MHz, CDCl3): δ 4.46 (q, J = 8.2 Hz, 2H), 2.40 (t, J = 7.0 Hz, 2H), 1.65 (m, 2H), 1.26 (m, 16H), 0.88 (t, J = 7.2 Hz, 3H). 19F NMR (471 MHz, CDCl3): δ –73.9 (t, J = 8.4 Hz, 3F). 13C NMR (126 MHz, CDCl3): δ 172.3, 123.2 (q, J = 277.2 Hz), 60.3 (q, J = 36.5 Hz), 33.8, 32.1, 29.7, 29.7, 29.6, 29.5, 29.3, 29.1, 24.9, 22.8, 14.2.
2,2,2-Trifluoroethyl tetradecanoate (2c) (16c)
Yellow solid; yield: 86.9 mg (93%). Petroleum ether as eluent for flash column chromatography. 1H NMR (500 MHz, CDCl3): δ 4.46 (q, J = 8.4 Hz, 2H), 2.41 (t, J = 7.2 Hz, 2H), 1.65 (m, 2H), 1.26 (m, 20H), 0.88 (t, J = 7.3 Hz, 3H). 19F NMR (471 MHz, CDCl3): δ –73.9 (t, J = 8.3 Hz, 3F). 13C NMR (126 MHz, CDCl3): δ 172.3, 123.2 (q, J = 277.1 Hz), 60.3 (q, J = 36.5 Hz), 33.8, 32.1, 29.8, 29.8, 29.8, 29.7, 29.6, 29.5, 29.3, 29.1, 24.9, 22.8, 14.3.
2,2,2-Trifluoroethyl stearate (2d) (16d)
White solid; yield: 108.8 mg (99%). Petroleum ether as eluent for flash column chromatography. 1H NMR (500 MHz, CDCl3): δ4.46 (q, J = 8.3 Hz, 2H), 2.41 (t, J = 7.1 Hz, 2H), 1.65 (m, 2H), 1.26 (m, 28H), 0.88 (t, J = 7.1 Hz, 3H). 19F NMR (471 MHz, CDCl3): δ –73.9 (t, J = 8.4 Hz, 3F). 13C NMR (126 MHz, CDCl3): δ 172.3, 123.2 (q, J = 276.7 Hz), 60.3 (q, J = 36.6 Hz), 33.8, 32.1, 29.8, 29.8, 29.8, 29.7, 29.6, 29.5, 29.3, 29.1, 24.9, 22.8, 14.3.
2,2,2-Trifluoroethyl docosanoate (2e) (6a)
White solid; yield: 125.5 mg (99%). Petroleum ether as eluent for flash column chromatography. 1H NMR (500 MHz, CDCl3): δ 4.46 (q, J = 8.3 Hz, 2H), 2.41 (t, J = 7.0 Hz, 2H), 1.65 (m, 2H), 1.26 (m, 36H), 0.88 (t, J = 7.1 Hz, 3H). 19F NMR (471 MHz, CDCl3): δ –73.9 (t, J = 8.3 Hz, 3F). 13C NMR (126 MHz, CDCl3): δ 172.3, 123.2 (q, J = 277.5 Hz), 60.3 (q, J = 36.3 Hz), 33.8, 32.1, 29.9, 29.8, 29.8, 29.7, 29.6, 29.5, 29.3, 29.1, 24.9, 22.8, 14.3.
2,2,2-Trifluoroethyl oleate (2f) (16e)
Light yellow oil; yield: 98.4 mg (90%). Petroleum ether as eluent for flash column chromatography. 1H NMR (500 MHz, CDCl3): δ 5.34 (s, 2H), 4.46 (q, J = 8.3 Hz, 2H), 2.41 (t, J = 7.2 Hz, 2H), 2.01 (m, 4H), 1.65 (m, 2H), 1.31–1.26 (m, 20H), 0.88 (t, J = 7.1 Hz, 3H). 19F NMR (471 MHz, CDCl3): δ –73.9 (t, J = 8.2 Hz, 3F). 13C NMR (126 MHz, CDCl3): δ 172.3, 130.2, 129.8, 123.2 (q, J = 278.0 Hz), 60.3 (q, J = 36.5 Hz), 33.8, 32.1, 29.9, 29.8, 29.7, 29.5, 29.2, 29.2, 29.1, 27.4, 27.3, 24.8, 22.8, 14.2.
2,2,2-Trifluoroethyl (9Z,12Z,15Z)-octadeca-9,12,15-trienoate (2g) (16f)
Light yellow oil; yield: 97.3 mg (90%). Petroleum ether as eluent for flash column chromatography. 1H NMR (500 MHz, CDCl3): δ 5.37 (m, 6H), 4.46 (q, J = 8.2 Hz, 2H), 2.81 (m, 4H), 2.41 (t, J = 7.4 Hz, 2H), 2.09–2.05 (m, 4H), 1.65 (m, 2H), 1.32–1.26 (m, 8H), 0.98 (t, J = 7.5 Hz, 3H). 19F NMR (471 MHz, CDCl3): δ –73.9 (t, J = 8.5 Hz, 3F). 13C NMR (126 MHz, CDCl3): δ 172.3, 132.1, 130.4, 128.5, 128.4, 127.9, 127.3, 123.2 (q, J = 277.8 Hz), 60.3 (q, J = 36.5 Hz), 33.8, 29.7, 29.2, 29.2, 29.1, 27.3, 25.8, 25.7, 24.8, 20.7, 14.4.
2,2,2-Trifluoroethyl (E)-oct-3-enoate (2h)
Light yellow oil; 92% 19F NMR yield using C6H5CF3 as an internal standard, 46% isolated yield. Petroleum ether as eluent for flash column chromatography. IR (KBr): 2960, 2928, 2873, 2858, 1762, 1657, 1595, 1496, 1457, 1410, 1379, 1353, 1283, 1236, 1170, 1062, 1041, 970, 843, 806,748, 664 cm–1. 1H NMR (500 MHz, CDCl3): δ 5.61 (m, 1H), 5.50 (m, 1H), 4.46 (q, J = 8.5 Hz, 2H), 3.13 (d, J = 6.8 Hz, 2H), 2.04 (q, J = 7.0 Hz, 2H), 1.37–1.28 (m, 4H), 0.89 (t, J = 7.1 Hz, 3H). 19F NMR (471 MHz, CDCl3): δ –73.9 (t, J = 8.6 Hz, 3F). 13C NMR (126 MHz, CDCl3): δ 170.7, 136.1, 123.1 (q, J = 277.2 Hz), 120.3, 60.5 (q, J = 36.8 Hz), 37.5, 32.2, 31.3, 22.3, 14.0. HRMS (EI): m/z [M]+ calcd for C10H15F3O2: 224.1024; found: 224.1026.
2,2,2-Trifluoroethyl 2-(cyclohex-1-en-1-yl)acetate (2i)
Light yellow oil; 90% 19F NMR yield using C6H5CF3 as an internal standard, 49% isolated yield. Petroleum ether and a mixture of petroleum ether and ethyl acetate (40:1, v/v) as eluents for flash column chromatography. IR (KBr): 3394, 3189, 2956, 2923, 2851, 1740, 1647, 1491, 1468, 1420, 1399, 1378, 1362, 1261, 1213, 1195, 1082, 1023, 966, 907, 855, 819, 804, 777, 722, 693, 646 cm–1. 1H NMR (500 MHz, CDCl3): δ 5.60 (m, 1H), 4.47 (q, J = 8.3 Hz, 2H), 3.04 (m, 2H), 2.03–2.00 (m, 4H), 1.65 (m, 2H), 1.57 (m, 2H). 19F NMR (471 MHz, CDCl3): δ –73.8 (t, J = 8.5 Hz, 3F). 13C NMR (126 MHz, CDCl3): δ 170.5, 130.2, 126.8, 123.1 (q, J = 277.6 Hz), 60.4 (q, J = 36.8 Hz), 43.0, 28.5, 25.4, 22.8, 22.0. HRMS (EI): m/z [M]+ calcd for C10H13F3O2: 222.0868; found: 222.0866.
2,2,2-Trifluoroethyl (2E,4E)-hexa-2,4-dienoate (2j) (16g)
96% 19F NMR yield using C6H5CF3 as an internal standard. GC-MS (m/z): 194.0 (M+). 19F NMR (471 MHz, CH2Cl2): δ –74.7 (t, J = 8.3 Hz, 3F).
2,2,2-Trifluoroethyl (2E,4E,6E,8E)-3,7-dimethyl-9-(2,6,6-trimethylcyclohex-1-en-1-yl)nona-2,4,6,8-tetraenoate (2k)
Yellow solid; yield: 103.3 mg (90%); m.p. 57–59°C. Petroleum ether and a mixture of petroleum ether and ethyl acetate (20:1, v/v) as eluents for flash column chromatography. IR (KBr): 3046, 2960, 2928, 2865, 2827, 2722, 1728, 1607, 1581, 1445, 1407, 1357, 1281, 1236, 1169, 1131, 1071, 1027, 967, 923, 888, 873, 842, 826, 791, 767, 724, 663, 644, 628 cm–1. 1H NMR (500 MHz, CDCl3): δ 7.07 (dd, J = 15.0, 11.5 Hz, 1H), 6.33 (d, J = 5.2, 1H), 6.30 (d, J = 4.2 Hz, 1H), 6.17 (s, 1H), 6.14 (d, J = 3.8 Hz, 1H), 5.83 (s, 1H), 4.49 (q, J = 8.6 Hz, 2H), 2.37 (s, 3H), 2.04–2.02 (m, 2H), 2.02 (s, 3H), 1.72 (s, 3H), 1.62 (m, 2H), 1.48–1.46 (m, 2H), 1.03 (s, 6H). 19F NMR (376 MHz, CDCl3): δ –73.7 (t, J = 8.7 Hz, 3F). 13C NMR (126 MHz, CDCl3): δ 165.2, 156.0, 140.8, 137.8, 137.3, 134.6, 132.5, 130.4, 129.4, 129.4, 123.4 (q, J = 278.1 Hz), 116.0, 59.8 (q, J = 36.4 Hz), 39.8, 34.4, 33.3, 29.1, 21.9, 19.4, 14.3, 13.1. HRMS (EI): m/z [M]+ calcd for C22H29F3O2: 382.2120; found: 382.2123.
2,2,2-Trifluoroethyl 2-(adamantan-1-yl)acetate (2l)
Light yellow oil; yield: 76.6 mg (92%). Petroleum ether as eluent for flash column chromatography. IR (KBr): 2906, 2851, 2678, 2661, 1758, 1452, 1409, 1349, 1282, 1255, 1234, 1168, 1132, 1101, 1062, 976, 932, 909, 873, 843, 804, 665, 649, 633, 555 cm–1. 1H NMR (500 MHz, CDCl3): δ 4.45 (q, J = 8.5 Hz, 2H), 2.17 (s, 2H), 1.98 (s, 3H), 1.72–1.60 (m, 12H). 19F NMR (471 MHz, CDCl3): δ –73.6 (t, J = 8.4 Hz, 3F). 13C NMR (126 MHz, CDCl3): δ 170.1, 123.2 (q, J = 277.2 Hz), 60.0 (q, J = 36.5 Hz), 48.3, 42.4, 36.8, 33.1, 28.7. HRMS (EI): m/z [M]+ calcd for C14H19F3O2: 276.1337; found: 276.1344.
2,2,2-Trifluoroethyl 2-([1,1′-biphenyl]-4-yl)acetate (2m)
White solid; yield: 83.0 mg (94%); m.p. 85–87°C. Petroleum ether and a mixture of petroleum ether and ethyl acetate (20:1, v/v) as eluents for flash column chromatography. IR (KBr): 3096, 3080, 3058, 3047, 3033, 2987, 2962, 2923, 1748, 1566, 1488, 1452, 1417, 1358, 1309, 1276, 1227, 1165, 1049, 979, 841, 821, 761, 739, 691, 665 cm–1. 1H NMR (500 MHz, CDCl3): δ 7.58 (m, 4H), 7.44 (t, J = 7.5 Hz, 2H), 7.36 (m, 3H), 4.51 (q, J = 8.2 Hz, 2H), 3.78 (s, 2H). 19F NMR (471 MHz, CDCl3): δ –73.7 (t, J = 8.4 Hz, 3F). 13C NMR (126 MHz, CDCl3): δ 170.2, 140.8, 140.7, 131.9, 129.8, 128.9, 127.6 127.5, 127.2, 123.0 (q, J = 277.8 Hz), 60.8 (q, J = 36.6 Hz), 40.3. HRMS (EI): m/z [M]+ calcd for C16H13F3O2: 294.0868; found: 294.0862.
2,2,2-Trifluoroethyl 2-(naphthalen-2-yl)acetate (2n) (16b)
White solid; yield: 81.3 mg (> 99%). Petroleum ether and a mixture of petroleum ether and ethyl acetate (40:1, v/v) as eluents for flash column chromatography. 1H NMR (500 MHz, CDCl3): δ 7.86–7.83 (m, 3H), 7.76 (s, 1H), 7.51–7.50 (m, 2H), 7.43 (d, J = 8.3 Hz, 1H), 4.53 (q, J = 8.3 Hz, 2H), 3.91 (s, 2H). 19F NMR (471 MHz, CDCl3): δ –73.7 (t, J = 8.3 Hz, 3F). 13C NMR (126 MHz, CDCl3): δ 170.1, 133.6, 132.8, 130.4, 128.6, 128.3, 127.8, 127.8, 127.2, 126.5, 126.2, 123.0 (q, J = 277.0 Hz), 60.8 (q, J = 36.6 Hz), 40.9.
2,2,2-Trifluoroethyl 2-phenylacetate (2o) (16h)
Light yellow oil; 95% 19F NMR yield using C6H5CF3 as an internal standard, 34% isolated yield. Petroleum ether and a mixture of petroleum ether and ethyl acetate (40:1, v/v) as eluents for flash column chromatography. 1H NMR (500 MHz, CDCl3): δ 7.35 (m, 2H), 7.31–7.29 (m, 3H), 4.49 (q, J = 8.3 Hz, 2H), 3.73 (s, 2H). 19F NMR (471 MHz, CDCl3): δ –73.8 (t, J = 8.4 Hz, 3F). 13C NMR (126 MHz, CDCl3): δ 170.1, 133.0, 129.5, 128.9, 127.6, 123.1 (q, J = 277.2 Hz), 60.7 (q, J = 36.6 Hz), 40.7.
Bis(2,2,2-trifluoroethyl) succinate (2p) (16i)
Light yellow oil; 80% 19F NMR yield using C6H5CF3 as an internal standard, 65% isolated yield. Petroleum ether and a mixture of petroleum ether and ethyl acetate (40:1, v/v) as eluents for flash column chromatography. 1H NMR (400 MHz, CDCl3): δ 4.49 (q, J = 8.3 Hz, 4H), 2.79 (s, 4H). 19F NMR (376 MHz, CDCl3): δ –73.9 (t, J = 8.3 Hz, 6F). 13C NMR (126 MHz, CDCl3): δ 170.5, 123.0 (q, J = 277.3 Hz), 60.7 (q, J = 36.8 Hz), 28.5.
(S)-2,2,2-Trifluoroethyl 2-((tert-butoxycarbonyl)amino)-3-phenylpropanoate (2q) (14c)
White solid; yield: 104.2 mg (>99%). Petroleum ether and a mixture of petroleum ether and ethyl acetate (10:1, v/v) as eluents for flash column chromatography. 1H NMR (400 MHz, CDCl3): δ 7.33–7.24 (m, 3H), 7.14 (d, J = 6.9 Hz, 2H), 4.94 (d, J = 7.4 Hz, 1H), 4.67 (m, 1H), 4.48 (m, 2H), 3.11 (m, 2H), 1.41 (s, 9H). 19F NMR (376 MHz, CDCl3): δ –73.6 (t, J = 8.4 Hz, 3F). 13C NMR (126 MHz, CDCl3): δ 170.8, 155.2, 135.5, 129.3, 128.9, 127.4, 122.8 (q, J = 277.0 Hz), 80.5, 61.0 (q, J = 37.0 Hz), 54.4, 38.0, 28.4.
(S)-2,2,2-Trifluoroethyl 1-tosylpyrrolidine-2-carboxylate (2r)
White solid; yield: 93.8 mg (89%); m.p. 54–56°C. Petroleum ether and a mixture of petroleum ether and ethyl acetate (10:1, v/v) as eluents for flash column chromatography. IR (KBr): 3066, 3030, 2980, 2930, 2881, 1774, 1598, 1494, 1450, 1412, 1350, 1305, 1283, 1160, 1095, 1071, 1046, 1008, 979, 921, 843, 817, 782, 751, 708, 666, 594, 549 cm–1. 1H NMR (400 MHz, CDCl3): δ 7.74 (d, J = 8.3 Hz, 2H), 7.32 (d, J = 8.2 Hz, 2H), 4.51 (m, 2H), 4.37 (dd, J = 8.4, 3.7 Hz, 1H), 3.50 (m, 1H), 3.29 (m, 1H), 2.42 (s, 3H), 2.09 (m, 1H), 1.98 (m, 2H), 1.78 (m, 1H). 19F NMR (376 MHz, CDCl3): δ –73.8 (t, J = 8.5 Hz, 3F). 13C NMR (126 MHz, CDCl3): δ 170.8, 144.0, 135.1, 129.9, 127.6, 122.8 (q, J = 277.8 Hz), 60.8 (q, J = 36.7 Hz), 60.1, 48.5, 31.1, 24.7, 21.6. HRMS (EI): m/z [M]+ calcd for C14H16F3NO4S: 351.0752; found: 351.0744.
(4aS,6aS,6bR,8aR,10S,12aR,12bR,14bS)-2,2,2-Trifluoroethyl 10-hydroxy-2,2,6a, 6b,9,9,12a-heptamethyl-1,2, 3,4,4a,5,6,6a,6b,7,8,8a,9,10,11, 12,12a,12b,13,14b-icosahydropicene-4a-carboxylate (2s)
White solid; yield: 151.9 mg (94%); m.p. 76–78°C. Petroleum ether and a mixture of petroleum ether and ethyl acetate (10:1, v/v) as eluents for flash column chromatography. IR (KBr): 3435, 2947, 2868, 1748, 1635, 1464, 1408, 1388, 1365, 1283, 1253, 1234, 1168, 1121, 1083, 1068, 1047, 1030, 994, 976, 919, 827, 748, 655 cm–1. 1H NMR (400 MHz, CDCl3): δ 5.31 (t, J = 3.5 Hz, 1H), 4.41 (m, 2H), 3.21 (m, 1H), 2.86 (dd, J = 14.0, 4.1 Hz, 1H), 2.02 (m, 1H), 1.88 (m, 2H), 1.75–1.30 (m, 20H), 1.14 (s, 3H), 0.98 (s, 3H), 0.92 (s, 3H), 0.90 (s, 6H), 0.78 (s, 3H), 0.71 (s, 3H). 19F NMR (376 MHz, CDCl3): δ –73.6 (t, J = 8.7 Hz, 3F). 13C NMR (126 MHz, CDCl3): δ 176.3, 143.2, 123.2 (q, J = 277.4 Hz), 123.1, 79.2, 60.3 (q, J = 36.4 Hz), 55.4, 47.7, 47.3, 45.9, 41.8, 41.5, 39.4, 38.9, 38.6, 37.2, 33.9, 33.2, 32.9, 32.3, 30.8, 28.3, 27.7, 27.3, 26.0, 23.6, 23.6, 23.1, 18.5, 16.9, 15.7, 15.5. HRMS (ESI): m/z [M + H]+ calcd for C32H50F3O3: 539.3707; found: 539.3705.
Supplement_Material.doc
Download MS Word (2.3 MB)Disclosure statement
No potential conflict of interest was reported by the authors.
Notes on contributors
Qiu-Yan Han is a senior undergraduate in Wuhan University of Technology. Her research interests are the synthesis of aryl(2,2,2-trifluoroethyl)iodonium triflates and their applications in trifluoroethylation reactions.
Cheng-Long Zhao is a first-year master student in the research group of Prof. Cheng-Pan Zhang at Wuhan University of Technology. He obtained his B.S. degree in pharmaceutical engineering from Wuhan University of Technology. His research interests focus on the transitionmetal-free trifluoroethylation reactions.
Jing Yang is a second-year master student in the research group of Prof. Cheng-Pan Zhang at Wuhan University of Technology. She obtained her B.S. degree in pharmaceutical engineering from Wuhan University of Technology. Her research interests include the transition-metal catalyzed trifluoroethylation and the synthesis of 1,1-difluorocyclopropane derivatives.
Cheng-Pan Zhang obtained his B.S. degree in pharmacy from Chongqing Medical University in 2005. He received his Ph.D. degree from Shanghai Institute of Organic Chemistry (SIOC) in 2011. From 2011 to 2012, he was a postdoctoral fellow at University of Hawaii. Then he moved to Texas A&M University and worked there for two years. Since the end of 2014, he joined the faculty of Wuhan University of Technology as a full professor. His current research interests are the synthesis and reactivity of arylsulfonium salts as well as the development of new fluorination and fluoroalkylation reagents and reactions.
Additional information
Funding
References
- (a) Alam, S.; Kalita, H.; Jayasooriya, A.; Samanta, S.; Bahr, J.; Chernykh, A.; Weisz, M.; Chisholm, B.J. Eur. J. Lipid Sci. Technol. 2014, 116, 2–15. (b) Swamy, K.C.K.; Kumar, N.N.B.; Balaraman, E.; Kumar, K.V.P.P. Chem. Rev. 2009, 109, 2551–2651. (c) Lotero, E.; Liu, Y.; Lopez, D.E.; Suwannakarn, K.; Bruce, D.A.; Goodwin, Jr. J.G. Ind. Eng. Chem. Res. 2005, 44, 5353–5363. (d) Tang, S.; Yuan, J.; Liu, C.; Lei, A. Dalton Trans. 2014, 43, 13460–13470. doi: 10.1002/ejlt.201300217
- (a) Knothe, G. Fuel Process. Technol. 2005, 86, 1059–1070. (b) Adamczak, M.; Bornscheuer, U.T.; Bednarski, W. Eur. J. Lipid Sci. Technol. 2009, 111, 808–813. (c) Pratas, M.; Freitas, S.; Oliveira, M.B.; Monteiro, S.C.; Lima, A.S.; Coutinho, J.A.P. J. Chem. Eng. Data 2010, 55, 3983–3990. (d) Akoh, C.C.; Chang, S.-W.; Lee, G.-C.; Shaw, J.-F. J. Agric. Food Chem. 2007, 55, 8995–9005. doi: 10.1016/j.fuproc.2004.11.002
- (a) Yang, Z.; Huang, Z.-L. Catal. Sci. Technol. 2012, 2, 1767–1775. (b) van den Broek, L.A.M.; Boeriu, C.G. Carbohyd. Polym. 2013, 93, 65–72. (c) van Kempen, S.E.H.J.; Schols, H.A.; van der Linden, E.; Sagis, L.M.C. Food Funct. 2014, 5, 111–122. doi: 10.1039/c2cy20109g
- (a) Marshall, D.L.; Bullerman, L.B. Food Sci Technol (Carbohydrate Polyesters as Fat Substitutes). 1994, 62, 149–167. (b) Kabara, J.J.; Marshall, D.L. Food Sci Technol (Antimicrobials in Food, 3rd Edition). 2005, 145, 327–360. (c) Xin, L. J. Chem. Pharm. Res. 2014, 6, 944–946. (d) Vihma, V.; Tikkanen, M.J. J. Steroid Biochem. Mol. Biol. 2011, 124, 65–76.
- (a) Duggan, P.J.; Humphrey, D.G.; McCarl, V. Aust. J. Chem. 2004, 57, 741–745. (b) Caldwell, N.; Jamieson, C.; Simpson, I.; Watson, A.J.B. Chem. Commun. 2015, 51, 9495–9498. (c) Morphy, J.R.; Rankovic, Z.; York, M. Tetrahedron Lett. 2002, 43, 6413–6415. (d) McAllister, L.A.; Bechle, B.M.; Dounay, A.B.; Evrard, E.; Gan, X.; Ghosh, S.; Kim, J.-Y.; Parikh, V.D.; Tuttle, J.B.; Verhoest, P.R. J. Org. Chem. 2011, 76, 3484–3497. (e) Queyroy, S.; Vanthuyne, N.; Gastaldi, S.; Bertrand, M.P.; Gil, G. Adv. Synth. Catal. 2012, 354, 1759–1764. (f) Nechab, M.; Blidi, L.E.; Vanthuyne, N.; Gastaldi, S.; Bertrand, M.P.; Gil, G. Org. Biomol. Chem. 2008, 6, 3917–3920. (g) White, J.D.; Green, N.J.; Fleming, F.F. Tetrahedron Lett. 1993, 34, 3515–3518. doi: 10.1071/CH04040
- (a) Petrov, J.G.; Möhwald, H. J. Phys. Chem. 1996, 100, 18458–18463. (b) Petrov, J.G.; Polymeropoulos, E.E.; Möhwald, H. Langmuir 2007, 23, 2623–2630. doi: 10.1021/jp9613596
- (a) Arafat, A.; Giesbers, M.; Rosso, M.; Sudhölter, E.J.R.; Schroën, K.; White, R.G.; Yang, L.; Linford, M.R.; Zuilhof, H. Langmuir 2007, 23, 6233–6244. (b) Du, W.; Xu, Z.; Nyström, A.M.; Zhang, K.; Leonard, J.R.; Wooley, K.L. Bioconjugate Chem. 2008, 19, 2492–2498. (c) Fang, J.; Zhang, Y.; Wu, Y. Energy Procedia 2012, 14, 133–136. (d) Biesta, W.; van Lagen, B.; Gevaert, V.S.; Marcelis, A.T.M.; Paulusse, J.M.J.; Nielen, M.W.F.; Zuilhof, H. Chem. Mater. 2012, 24, 4311–4318. doi: 10.1021/la7007045
- From RCO2H catalyzed by Brønsted acids: (a) Lu, W.; Xie, K.; Chen, Z.; Pan, Y.; Zheng, C.M. J. Fluorine Chem. 2014, 161, 110–119. (b) Schroeder, G.K.; Wolfenden, R. Biochemistry 2007, 46, 4037–4044. (c) Schmidt-Leithoff, J.; Brückner, R. Helv. Chim. Acta, 2005, 88, 1943–1959. (d) Schmidt-Leithoff, J.; Brückner, R. Synlett. 2006, 2641–2645. From RCO2H catalyzed by DCC, EDC and etc: (e) Miyazawa, T.; Nakajo, S.; Nishikawa, M.; Hamahara, K.; Imagawa, K.; Ensatsu, E.; Yanagihara, R.; Yamada, T. J. Chem. Soc., Perkin Trans. 2001, 1, 82–86. (f) Shelkov, R.; Nahmany, M.; Melman, A. J. Org. Chem. 2002, 67, 8975–8982. doi: 10.1016/j.jfluchem.2014.02.006
- From (RCO)2O:Evenson, S.A.; Fail, C.A.; Badyal, J.P.S. J. Phys. Chem. B 2000, 104, 10608–10611. doi: 10.1021/jp002059y
- Lipase-catalyzed interesterification of RCO2R’:Li, X.-G.; Kanerva, L.T. Org. Lett. 2006, 8, 5593–5596. doi: 10.1021/ol0623163
- From RCOCl: (a) Tsai, S.W.; Cheng, I.C.; Huang, C.M. Chem. Eng. Sci. 2000, 55, 4571–4582. (b) Kohler, N.; Fryxell, G.E.; Zhang, M. J. Am. Chem. Soc. 2004, 126, 7206–7211. (c) Sun, Q.-Y.; de Smet, L.C. P.M.; van Lagen, B.; Giesbers, M.; Thüne, P.C.; van Engelenburg, J.; de Wolf, F.A.; Zuilhof, H.; Sudhölter, E.J.R. J. Am. Chem. Soc. 2005, 127, 2514–2523. (d) Lin, H.-Y.; Lay, E.; Wen, W.-Y.; Dewi, H.; Cheng, Y.-C.; Tsai, S.-W. J. Phys. Org. Chem. 2004, 17, 387–392. (e) Lin, H.-Y.; Tsai, S.-W. J. Mol. Catal. B-Enzym. 2003, 24–25, 111–120. (f) Chen, C.-C.; Tsai, S.-W.; Villeneuve, P. J. Mol. Catal. B-Enzym. 2005, 34, 51–57. (g) Wu, A.-C.; Wang, P.-Y.; Lin, Y.-S.; Kao, M.-F.; Chen, J.-R.; Ciou, J.-F.; Tsai, S.-W. J. Mol. Catal. B-Enzym. 2010, 62, 235–241. (h) Ren, Q.; Zhao, T. Carbohyd. Polym. 2010, 80, 381–386. doi: 10.1016/S0009-2509(00)00093-2
- Meese, C.O. Synthesis 1984, 12, 1041–1042. doi: 10.1055/s-1984-31070
- (a) Umemoto, T.; Gotoh, Y. J. Fluorine Chem. 1985, 28, 235–239. (b) Umemoto, T.; Gotoh, Y. J. Fluorine Chem. 1986, 31, 231–236. (c) Umemoto, T.; Gotoh, Y. Bull. Chem. Soc. Jpn. 1986, 59, 439–445. (d) Umemoto, T.; Gotoh, Y. Bull. Chem. Soc. Jpn. 1987, 60, 3823–3825. (e) Umemoto, T.; Gotoh, Y. Bull. Chem.Soc. Jpn. 1991, 64, 2008–2010. (f) Montanari, V.; Resnati, G. Tetrahedron Lett. 1994, 35, 8015–8018. (g) Zhdankin, V.V.; Kuehl, C.J.; Simonsen, A.J. Tetrahedron Lett. 1995, 36, 2203–2206. (h) Zhdankin, V.V.; Kuehl, C.J.; Simonsen, A.J. J. Org. Chem. 1996, 61, 8272–8276. (i) DesMarteau, D.D.; Montanari, V. Chem. Commun. 1998, 2241–2242. (j) DesMarteau, D.D.; Montanari, V. Chem. Lett. 2000, 29, 1052–1053. (k) Zhang, J.; Martin, G.R.; DesMarteau, D.D. Chem. Commun. 2003, 2334–2335. (l) DesMarteau, D.D.; Lu, C. Tetrahedron Lett. 2006, 47, 561–564. (m) Lu, C.; DesMarteau, D.D. J. Fluorine Chem. 2007, 128, 832–838. (n) DesMarteau, D.D.; Lu, C. J. Fluorine Chem. 2007, 128, 1326–1334. (o) Lu, C.; DesMarteau, D.D. Chem. Commun. 2008, 208–210. (p) Lu, C.; VanDerveer, D.; DesMarteau, D.D. Org. Lett. 2008, 10, 5565–5568. (q) Chu, A.-H.A.; Minciunescu, A.; Montanari, V.; Kumar, K.; Bennett, C.S. Org. Lett. 2014, 16, 1780–1782. (r) Tolnai, G.L.; Székely, A.; Makó, Z.; Gáti, T.; Daru, J.; Bihari, T.; Stirling, A.; Novák, Z. Chem. Commun. 2015, 51, 4488–4491. (s) Umemoto, T.; Gotoh, Y. Bull. Chem. Soc. Jpn. 1987, 60, 3307–3313. (t) Tóth, B.L.; Kovács, S.; Sályi, G.; Novák, Z. Angew. Chem. Int. Ed. 2016, 55, 1988–1992. (u) Yang, J.; Han, Q.-Y.; Zhao, C.-L.; Dong, T.; Hou, Z.-Y.; Qin, H.-L.; Zhang, C.-P. Org. Biomol. Chem. 2016, 14, 7654–7658. (v) Tolnai, G.L.; Nilsson, U.J.; Olofsson, B. Angew. Chem. Int. Ed. 2016, 55, 11226–11230. doi: 10.1016/S0022-1139(00)81146-X
- (a) Sevov, C.S.; Brooner, R.E.M.; Chénard, E.; Assary, R.S.; Moore, J.S.; Rodríguez-López, J.; Sanford, M.S. J. Am. Chem. Soc. 2015, 137, 14465–14472. (b) Fang, X.; Li, J.; Wang, C.-J. Org. Lett. 2013, 15, 3448–3451. (c) Baytas, S.N.; Wang, Q.; Karst, N.A.; Dordick, J.S.; Linhardt, R.J. J. Org. Chem. 2004, 69, 6900–6903. (d) Das, B.C.; Smith, M.E.; Kalpana, G.V. Bioorg. Med. Chem. Lett. 2008, 18, 3805–3808. doi: 10.1021/jacs.5b09572
- (a) Čaplar, V.; Žinić, M.; Pozzo, J.-L.; Fages, F.; Gudrun, M.-G.; Vögtle, F. Eur. J. Org. Chem. 2004, 4048–4059. (b) Franchini, S.; Baraldi, A.; Sorbi, C.; Pellati, F.; Cichero, E.; Battisti, U.M.; Angeli, P.; Cilia, A.; Brasili, L. Med. Chem. Commun. 2015, 6, 677–690. doi: 10.1002/ejoc.200400105
- (a) Lin, G.; Liu, S.-H.; Wu, F.-C.; Jen, W.-J. Synth. Commun. 1993, 23, 2135–2138. (b) Koller, K.L.; Dorn, H.C. Anal. Chem. 1982, 54, 529–533. (c) Monteiro, C.M.; Lourenço, N.M.T.; Afonso, C.A.M. Tetrahedron: Asymmetry. 2010, 21, 952–956. (d) Shibuta, D.; Watanabe, M.; Sato, Y. JP 61012645A, 1986. (e) Prabhakar, S.; Vivès, T.; Ferrières, V.; Benvegnu, T.; Legentil, L.; Lemiègre, L. Green Chem. 2017, 19, 987–995. (f) Zhao, T.; Ren, Q. CN 101608402A, 2009. (g) Hatakenaka, H.; Takahashi, Y.; Matsumoto, A. Polym. J. 2003, 35, 640–651. (h) Vandamme, M.; Bouchard, L.; Gilbert, A.; Keita, M.; Paquin, J.-F. Org. Lett. 2016, 18, 6468–6471. (i) Kunugi, Y.; Chen, P.-C.; Nonaka, T. J. Electrochem. Soc. 1993, 140, 2833–2836. doi: 10.1080/00397919308018607