ABSTRACT
Sageretia thea (Osbeck.) was used as an effective chelating agent for the biosynthesis of iron oxide nanoparticles (IONP's) and extensively characterized through XRD, FTIR, Raman spectroscopy, Energy Dispersive Spectroscopy, HR-SEM/TEM and SAED. Antibacterial assays against five human pathogenic bacterial strains were carried out and minimum inhibitory concentrations were calculated. Pseudomonas aeruginosa (MIC: 7.4 µg/mL) was the most susceptible strain to biosynthesized IONPs. All of the fungal strains showed susceptibility to the IONPs. MTT cytotoxic assay was carried out against the promastigote and amastigote cultures of Leishmania tropica and their IC50 values were calculated as 17.2 and 16.75 µg/mL. The cytotoxic potential was further assessed using brine shrimps, and the IC50 was calculated as 16.46 µg/mL. Moderate antioxidant activities were reported. Human RBCs and macrophages were found to be biocompatible with biogenic IONPs (IC50 > 200 µg/mL).
GRAPHICAL ABSTRACT
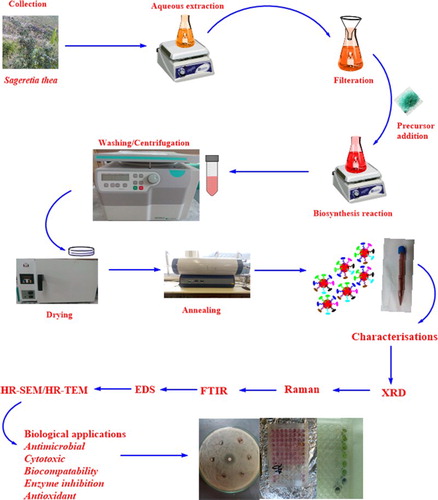
1. Introduction
The interface of green chemistry and nanotechnology presents an exciting horizon for synthesizing green and clean multifunctional metal oxide nanoparticles. Besides silver and gold (Citation1), biogenic synthesis of nanoparticles has been extended to various metal oxides that exhibit interesting properties (Citation2–9). Multifunctional iron oxide nanoparticles (IONPs) have emerged as a promising material for a wide range of applications, such as biomedicine, cosmetics, bioremediation, diagnostics and materials engineering (Citation10–14). However, applications of IONPs in biomedicine are growing exponentially. The ability of IONPs in the controlled release of drugs for tissue repair, MRI and treatment of solid tumors has previously been demonstrated (Citation15). The wide range of applications can be attributed to its ideal properties such as higher surface area, small band gap and stability.
Numerous chemical and physical means are used for the synthesis of iron nanoparticles. Chemical-based methods used in the synthesis of IONPs include thermal decomposition hydrothermal synthesis, reverse micelles, co-precipitation, micro-emulsion technology, sol–gel synthesis, sonochemical reactions, thermolysis and hydrolysis of precursors, electrospray synthesis, flow injection synthesis and colloidal chemistry-based methods (Citation16). In certain methods such as hydrothermal reaction, co-precipitation and sol–gel process, the dispersion and uniformity of size and particle distribution were poor (Citation17). Apart from these disadvantages, IONPs have been synthesized using solvents such as sodium borohydride, hydrazine and sodium dodecyl sulfate (Citation18,Citation19). Such chemical synthesis methods can generate toxic waste streams that are harmful for the environment and human health (Citation20). These chemical routes provide more chances of agglomeration of nanoparticles (Citation21). Likewise, physical means of synthesis are often costly because of intense energy requirements (Citation2). Hitherto, these physical/chemical methods are effective in synthesis; there is a paradigm shift toward cheap, sustainable, green and eco-friendly methods (Citation22), which can be used for the synthesis of IONPs. Numerous biological resources such as algae, fungi, bacteria and plants have emerged as cost-effective and eco-friendly platforms for the synthesis of nanoparticles. The interface of medicinal plants and biogenic nanoparticles has attracted numerous researchers to fabricate nanomaterials with diverse applications. Medicinal plants have been widely employed and preferred for the synthesis of nanoparticles because of their rich phytochemistry and bioactive components (Citation23).
Bioinspired synthesis of IONPs has already been demonstrated in some recent reports. Bioactive components of the plant extracts are used as reducing agents in the synthesis process. Highly crystalline IONPs were reported using the aqueous leaf extracts of Azadirachta indica (Citation24). Camellia- sinensis-mediated IONPs indicated four times larger surface area relative to the commercial ones (Citation25). Lawsonia inermis, Amaranthus dubius, Rosmarinus officinalis, Melaleuca nesophila, Padina pavonica, etc., are some of the other medicinal plants being effectively used in the biosynthesis of IONPs (Citation15). Furthermore, these green IONPs were found to be nontoxic to human beings when compared to their chemically produced counterparts and also indicated higher biocompatibility and impressive antimicrobial properties (Citation26–28).
This contribution reports on the bioinspired synthesis of nano-scaled single-phase pure maghemite Fe2O3 nanoparticles, bio-engineered for the first time via complete green process using the aqueous leaf extracts of Sageretia thea as an effective reducing as well as capping agent without addition of any further chemical components (acids/base).
2. Materials and methods
2.1. Plant material
2.1.1. Plant material processing
The plant sample collected from Islamabad was taxonomically verified as S. thea (Osbeck.) in the Department of Plant Sciences, while the herbarium specimen (MOSEL-343) was deposited in the herbarium of Molecular Systematics and Applied Ethnobotany Lab (MoSAEL), Department of Biotechnology, Quaid-i-Azam University, Islamabad. Fresh and healthy leaves were excised, washed with distilled water, dried and ground to fine powder in a Willy mill. Powdered plant material was stored and used further for aqueous extraction.
2.2. Biosynthesis of IONPs
The standard procedure as established by previous authors for the biosynthesis of metal oxide nanoparticles was used with minor modifications (Citation22). Thirty grams of plant material was added to 200 mL of deionized water and heated at 80°C for 1 h on a magnetic stirrer hotplate for the extraction of bioactive components. Solid residues were removed by filtering the resultant solution three times using Whattman filter paper. To 100 mL of filtered solution (pH:5.7), 6.0 g of iron sulfate hepta hydrate (Alfa Aesar) was added as a precursor salt and heated at 85°C for 2 h. The color of the solution changed from brownish dye to violet color, and the pH was recorded as 4.3. Reaction mixture was cooled and centrifuged at 10,000 rpm for 10 min to collect the precipitate assumed as IONPs. The obtained precipitate was washed three times with distilled water, dried and annealed at 500°C to obtain highly crystalline IONPs. Annealing was performed to ensure full crystallization of the nanoparticles as well as decomposition of any extra compound from the natural extracts, not involved in the biosynthesis reaction.
2.3. Physical characterizations
To confirm the pure phase of maghemite IONPs, the annealed IONPs were subjected to intensive physical characterization techniques such as X-ray diffraction, Fourier Transformed Infrared Spectroscopy, Raman spectroscopy, Energy Dispersive Spectroscopy (EDS), Selected Area Electron Diffraction and High resolution Scanning/Transmission electron microscopy. XRD analysis was carried using X-ray diffractometer (Model: Bruker AXS D8 Advance) with irradiation line Kα of copper (λ = 1.5406 A°) while their corresponding size was calculated using Debye–Scherer equation (<Øsize> = Kλ/Δθ1/2 cosθ). Vibrational properties of IONPs were studied using Raman spectra recorded over the spectral range (0–2000 cm–1) using laser line of 473 nm with average excitation power of 2.48 mW. To investigate the major functional groups in the aqueous extracts of S. thea, Attenuated Total Reflectance (4000–600 cm–1) was carried out for the powdered aqueous extracts using Thermo Scientific Nicolet iS10 Spectrometer (Thermo Scientific, Waltham, MA) equipped with a Smart iTR ATR accessory, with a diamond crystal. FTIR analysis for the bioinspired IONPs was carried out over the spectral range of 400–4000 cm–1. Elemental composition was investigated using EDS while for morphological and shape observations HR-SEM and HR-TEM were used.
2.4. Antimicrobial activities
2.4.1. Antibacterial activity
Disc diffusion assay (Citation29) was used to investigate the antibacterial activities against five pathogenic bacterial strains (Escherichia coli, Bacillus subtilis, Staphylococcus epidermidis, Klebsiella pneumoniae and P. aeruginosa), while their minimum inhibitory concentrations were investigated using broth dilution assay. Fresh cultures were standardized to the optical density of 0.5, which corresponds to 1 × 108 CFU/mL. Uniform microbial lawns were prepared using sterilized bent glass rod after dispensing 100 µL of already standardized culture. Filter discs (6 mm) loaded with 10 µL IONPs (1000–31.25 µg/mL) final concentrations were carefully placed on the microbial lawns while gentamycin disc (10 µg) was used as positive control. Zones of inhibitions were recorded in millimeters on a vernier caliper. MICs were calculated using broth dilution assay by applying further lower concentrations of bioinspired IONPs.
2.4.2. Antifungal activity
A previously described protocol for the linear mycelial growth inhibition was employed to investigate the antifungal properties (Citation30) of bioinspired IONPs against Aspergillus niger, Aspergillus flavus, Aspergillus fumigatus, Mucor racemosus and Rhizopus Solanai. Sabouraud Dextrose Liquid medium (Oxoid CMO147) supplemented with 1.5% agar technical (Oxoid LP0012) was used to prepare fungal growth media. Autoclaved media (4 mL) was poured in sterilized test tubes and allowed to cool to ∼50°C. About 66.6 µL of test IONP samples (2000–500 µg/mL) was added and media were solidified in slanting position. Fungal spores from the freshly cultured strains were inoculated to the base of test tubes carefully using sterilized nichrome wire loop. Amphotericin B was used as positive control, while slants without any sample were used as negative control. After incubation for 5 days, at 30°C, linear growth inhibition was measured using the below equation:
2.5. Cytotoxic activities
2.5.1. Antileishmanial activity (promastigotes and amastigotes)
MTT cytotoxic assay as previously described (Citation31) was performed against axenic L. tropica KMH23 promastigotes and amastigotes cultures. Leishmania can exist in two forms: promastigotes (outside the human body) and amastigotes (inside the human body). Promastigotes are flagellated while amastigotes are ovoid and non-flagellated (Citation32). MI99 medium (GIBCO) added with 10% heat-inactivated fetal bovine serum was used for culturing the parasite. Cultures were grown to a density of 1 × 106 cells/mL. IONPs with final concentration of 200–1 µg/mL were used. The assay was performed in 96-well plate. The seeded plates with IONPs and culture media were incubated at 24°C for 72 h to a density of 1 × 106 cells/well in humified incubator with 5% CO2, and readings were taken at 540 nm. The survived promastigotes were counted with hemocytometer under inverted microscope, while their IC50 values were calculated using table curve software. Percent inhibition was calculated as:
2.5.2 Brine shrimp cytotoxicity
Artemia salina larvae were used to further investigate the cytotoxic potential of bioinspired IONPs in 96-well plates (Citation31–33). A. salina eggs (Ocean star, U.S.A.) were incubated for 24–48 h under light at 30°C in sea water (38 g/L supplemented with 6 mg/L dried yeast). Ten mature phototropic nauplii were harvested using Pasteur pipette and transferred to each well of the plate. Test concentrations of IONPs were added to each well. DMSO was used as negative control and doxorubicin as positive control. Percent of dead shrimps was calculated in each well after incubation at 24 h. IC50 values were calculated using table curve software ().
Table 1. IC50 calculation.
2.6. Biocompatibility assays
2.6.1. Human RBCs
Hemolytic assay was carried out to study the compatibility of bioinspired IONPs using fresh isolated RBCs from healthy blood donor (Citation34). One milliliter of the freshly collected blood was centrifuged at 14,000 rpm for 5 min for isolation of erythrocytes. Erythrocytes suspension was prepared by adding PBS (pH 7.2) to 200 µL of isolated erythrocytes. Hundred microliters of different test concentrations of IONPs were added to 100 µL of erythrocytes suspension and incubated for 1 h at 35°C in 96-well plate. After incubation, samples were centrifuged at 10,000 rpm for 10 min. Absorbance of the collected supernatant was monitored at 530 nm to find the percent hemoglobin release. DMSO and Triton X-100 were used as negative and positive controls. Percent hemolysis was found out using the following equation:
2.6.2. Human macrophages
Cytotoxicity of bioinspired IONPs was also determined against freshly isolated human macrophages. Macrophages were isolated using the blood of a healthy donor using the previously described standard procedure based on ficoll-gastrografin density gradient (density = 1.070 g/mL) (Citation35). A typical procedure comprises slow dissolution of ficoll (5.7 g in 95 mL distilled water to which 5 mL of gastrografin has been previously added). Hank’s buffer salt solution was used for dilution of blood, which was layered gently on the ficoll-gastrografin, followed by centrifugation at 400 g for 30 min and purification with percoll gradient (density 1.064 g/mL) adjusted with sterilized deionized water. The white-layered macrophages isolated were cultured in RPMI medium enriched with heat-inactivated FBS (10%), Hepes (25 mM) and antibiotics (Streptomycin: 0.1 mg/mL; Penicillin: 100 U/mL). Cells were cultured to a density of 1 × 105 cells/well in a humified incubator with 5% CO2. Percent inhibition was calculated using the below formula:
2.7. Protein kinase inhibition
Streptomyces 85E strain cultured in ISP4 minimal media was used to investigate the protein kinase inhibition activity of bioinspired IONPs. Filter paper discs loaded with 10 µL of serially diluted IONPs were placed after on the culture plates after making uniform lawns. Surfactin was used as positive control. Zone of inhibition was measured in millimeters after 72 h incubation at 30°C (Citation29).
2.8. Alpha amylase inhibition
The microplate method was applied to study the alpha amylase enzyme inhibition potential of the bioinspired IONPs (Citation31). Reaction mixture is composed of 15 µL PBS and 25 µL α-amylase enzyme, 10 µL of test samples and 40 µL of starch solution. Positive and negative controls were composed of acarbose and deionized water respectively. Percent enzyme inhibition was calculated as:where “ODS,” “ODN” and “ODB” correspond to the optical densities of sample, negative control and blank.
2.9. Antioxidant activities
2.9.1. DPPH-free radical scavenging
2,2-Diphenyl 1-picrylhydrazyl (DPPH) radical scavenging ability was investigated using different test concentrations (200–1 µg/mL) by the spectrophotometric method (Citation31). Reagent solution was prepared by adding 2.4 mg of DPPH to 25 mL of methanol. Ascorbic acid was considered positive control, while DMSO was used as negative control. 200 µL of reaction mixture is composed of 180 µL of reagent solution and 20 µL of test sample. After incubation for 1 h, readings were recorded at 517 nm to investigate the percent scavenging of DPPH, which was calculated using the following formula:where “ABS” and “ABC” correspond to absorbance of sample and absorbance of control.
2.9.2. Total antioxidant capacity
Total antioxidant capacity (TAC) was investigated using phosphomolybdenum method (Citation36). In a typical procedure, 20 µL of test samples are added to 180 µL of reagent mixture. Reagent mixture is composed of 0.6 M H2SO4, 28 mM NaH2PO4, 4 mM (NH4) 6Mo7O24.4H2O. The reaction mix was incubated at 95°C for 90 min. Readings were recorded at 695 nm. Results are expressed as number of microgram equivalents of ascorbic acid per milligram of the sample, that is, µg AAE/mg.
2.9.3. Total reducing power
The K3Fe (CN)6 (potassium ferricyanide)-based method was used to investigate total reducing power of bioinspired IONPs (Citation37). To 50 µL of PBS, 40 µL of test sample was added, followed by incubation at 50°C for 20 min, and then 50 µL of (10%) tri-chloro acetic acid was added. The reaction mix was centrifuged at 3000 rpm for 10 min. To the 166.6 µL of the collected supernatant, 33.3 µL of (0.1%) FeCl3 was added in a 96-well plate. DMSO and ascorbic acid were used as negative and positive controls. Absorbance was recorded at 630 nm and the results were expressed as ascorbic acid equivalents per milligram.
3. Results and discussion
3.1. Biosynthesis of IONPs
Chemical and physical nanoparticle synthesis procedures are either laborious, time consuming or costly. Sometimes even unwanted toxic chemicals can remain adsorbed to the surface of nanoparticles and eventually hinder its widespread therapeutic applications (Citation38,Citation39). A green, cheap, rapid and simple method for the biosynthesis IONPs has been successfully demonstrated for the first time using aqueous leaf extracts of S. thea. Biomimetic synthesis is more acceptable because it has no disadvantages relative to the physical/chemical methods and hence can be effectively applied for biological applications. S. thea has already been used in traditional medicines and in making tea in parts of Korea and China. Bioactive compounds such as Taraxerol, Quercetin, Syringic acid, Myricetrin, Kaempferol and Daucosterol have previously been reported from S. thea (Citation40–42), which has an intended role in reducing, capping and stabilizing of IONPs. The valency of Fe in sulfate hepta hydrate is 2+ at room temperature, which changes to Fe+3 during the reaction process because of electron-donating nature of iron at higher temperature. The oxidation process here is governed by temperature (85°C). At higher temperature, the reduced Fe+2 changes to Fe+3, which then combines with the oxygen present in the system or released by the phenolic-rich components in the plant extracts, resulting in the formation of Fe2O3 ().
3.2. Room temperature physical characterizations
X-ray diffraction spectra of the biosynthesized IONPs annealed at 500°C in open air furnace were recorded ((A)). The observed Bragg peaks were found consistent with single pure-phase (Fe2O3) tetragonal maghemite (JCP pattern: 00-025-1402). Lattice constants were deduced as <aexp.> = 8.34000 A° and <cexp.> = 25.02000 A°. Crystallographic reflections of (209, 203, 205, 206, 216, 105 and 1115) indicate a highly crystalline IONPs. It is worth mentioning that magnetite and maghemite are isostructural, which means that the XRD technique cannot clearly differentiate between them, especially in the nanophase state where the characteristic reflections are broad and appear almost at the same 2θ positions (Citation43,Citation44). The position of the peaks was compared on the 2θ scale with earlier reports and their positions were found in close resemblance with the earlier reports (Citation44–48). Debye–Scherer equation was employed to calculate the size of average nanoparticles, which was found to be ∼29 nm ((B)). No other components were found up to the level of XRD analysis that underlines the purity of bioinspired IONPs. HR-SEM was carried out to identify the shape of bioinspired IONPs ((E)), which confirms the formation of tetragonal crystalline shape, in line with the XRD data. The size of the as-synthesized nanoparticles was found in agreement to the size calculated using XRD data by Scherrer approximation. Moreover, a large concentration of the particles was of the size of ∼30 nm, which aligns with the data collected through XRD and digitization of via image J software. Particle distribution was studied through HR-TEM and the results perfectly simulated the data generated from XRD analysis ((A and B)). Spotty pattern in the SAED analysis also confirms the crystallinity of IONPs ((C)).
Figure 3. Morphological investigations: (A) HR-TEM at 50 nm; (B) particle distribution; (C) SAED pattern; (D) HR-TEM image; and (E) HR-SEM.
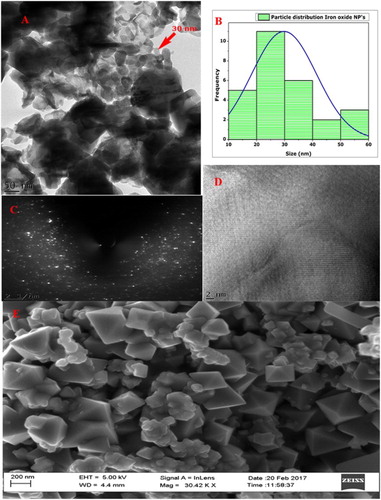
Vibrational features of the biogenic IONPs were studied using FTIR. FTIR spectrum was recorded over the spectral range (4000–400 cm–1). Characteristics of IR absorption were studied ((A)). An FTIR absorption peak of ∼500 cm–1 corresponds to the characteristic Fe–O vibration mode as indicated in the earlier studies (Citation49,Citation50). Broad –OH stretching can be observed at ∼3400 cm–1, which is usually attributed to the presence of phenolic compounds. Other IR absorption peaks can be observed at ∼1100–1200, 1600 and 2200 cm–1, which can be attributed to the –C–O, –C=O and –CN functional groups, respectively. These IR absorptions are attributed to the phytochemical components that are used in the reduction, capping as well as stabilizing of the IONPs. These bioactive components remained adhered to the biogenically synthesized IONP even after being subjected to a high thermal annealing temperature of 500°C. (B) indicates Raman spectra recorded at using Argon laser green excitation λexc = 514 nm. Spectrum indicates Raman shift at ∼388, ∼500 and ∼772 cm–1, which corresponds to T2g, Eg and A1g Raman active phonon modes specific to maghemite. Maghemite generally has three Raman active phonon modes (Citation51). Pronounced peak A1g is enough to assign maghemite phase to the biosynthesized IONPs, which is in line with the data obtained from XRD and in agreement with earlier reports. Here, it is noteworthy to mention that positioning of the peaks varies with the method used in synthesis and distribution vacancies within the unit cell (Citation52). To the best of our knowledge, maghemite-phase IONPs have never been reported via green chemistry. To further investigate the elemental composition of the bioinspired IONPs, EDS was employed ((C)). No other elements except carbon have been indicated by the EDS analysis. Peak of carbon is attributed to the grid support.
3.3. Antimicrobial properties of bioinspired IONPs
Antibacterial properties of bioinspired IONPs were investigated against five pathogenic strains (E. coli, B. subtilis, S. epidermidis, K. pneumoniae and P. aeruginosa) across different concentrations (1000–31.25 µg/mL) using disc diffusion assay and their corresponding MICs were investigated at concentrations <31.25 µg/mL to the bioinspired IONPs. In general, all bacterial strains were susceptible to the biogenic IONPs, while S. epidermidis and P. aeruginosa were found most susceptible with MICs of 7.8 µg/mL each. It is interesting to note that earlier reports indicated no activity for chemically synthesized IONPs against P. aeruginosa at very high concentrations such as 50 mg/mL (Citation53). However, as indicated in our results (), P. aeruginosa was significantly inhibited. Similarly, moderate antibacterial activities were reported for IONPs synthesized with Balanites aegyptiaca oil by the co-precipitation method (Citation54). Medicinal plants with antimicrobial potential have rarely been used for biosynthesis of IONPs. Our results suggest significant antibacterial activities against human pathogenic bacterial strains.
Antifungal activities against five pathogenic strains were demonstrated () using linear growth inhibition. Antifungal activity was evaluated against M. racemosus, A. niger, A. flavus, A. fumigatus and R. solanai. Linear growth inhibition was recorded at all of the tested concentrations (i.e. 2–0.5 mg/mL). Percent inhibition was found to be highest against R. solanai (79.03% ± 2.90), A. fumigatus (74.58% ± 3.15) and M. racemosus (74% ± 3.20) and at 2 mg/mL. However, none of the tested sample gave percent inhibition greater then positive control Amp B. It is noteworthy to mention that the antifungal activities of the biogenically synthesized IONPs against the selected bacterial strains using linear mycelial growth inhibition assay have never been performed. We further conclude a dose-dependent response toward bioinspired IONPs.
Several researchers have tried to explain the antimicrobial effects of IONPs. Many researchers have considered generation of reactive oxygen species leading to cellular oxidative damage as a mechanism for the nanomaterial based microbial toxicity. A part from ROS generations, other non-oxidant factors such as membrane damage because of sorption of nanoparticles to the surface can lead to cellular injury. Similarly, surface defects in the nanoparticle symmetry can also contribute to the enhancement of antibacterial properties by causing injury to the cells (Citation55). In addition, we further propose the role of bioactive functional groups such as phenols adhered from the aqueous leaf extracts of S. thea used in capping-stabilizing IONPs have a significant contribution to antimicrobial potency.
3.4. Cytotoxic activities
3.4.1. MTT cytotoxicity against axenic L. tropica cultures
Leishamniasis has an annual incidence rate of ∼1.2 million, while the parasite has been endemic in 100 countries. Previous treatment of Leishmania included antimonial compounds, which are not preferred anymore because of drug resistance and associated side effects (Citation56). Metal-oxide-nanoparticle (oxides of zinc, titanium, magnesium)-based treatments have gained popularity because of their impressive cytotoxicity against Leishmania (Citation56); however, biosynthesized IONPs have never been explored for their toxicity against L. tropica (KMH23). Biogenically synthesized IONPs in the range of 200–1 µg/mL were investigated via MTT cytotoxic assay against the promastigote and amastigote axenic cultures of L. tropica (). Bioinspired IONPs were found to have significant inhibition against Leishmania parasite in promastigote as well as amastigote form. IC50 values were calculated as 17.2 µg/mL and 16.75 µg/mL for promastigote and amastigote, concluding the futuristic possibilities of the use of bioinspired IONPs in antileishmanial therapies.
3.4.2. Brine shrimp cytotoxicity
To further confirm the cytotoxicity of bioinspired IONPs, they were further screened against brine shrimps to investigate the percent mortality. Brine shrimps (Artemia salina) are used as a standard to screen the cytotoxic compounds, which can be further studied for their anticancer and antimicrobial properties. Cytotoxicity testing of brine shrimps further confirmed the potential cytotoxic behavior of bioinspired IONPs, as indicated in . Bioinspired IONPs were found to be highly active at 200 µg/mL with 100% indicated by the 100% mortality of brine shrimps. At lower concentrations, there is a gradual decrease in the cytotoxic behavior. Median lethal concentration (IC50) was calculated as 16.46 µg/mL. These results confirm the cytotoxic behavior of biosynthesized IONPs.
3.4.3. Biocompatibility against human macrophages and RBCs
MTT cytotoxic assay was performed against freshly isolated human macrophages through the ficoll method. Macrophages are the important cells responsible for the immunity of a person against infections. Results of the MTT cytotoxicity are indicated in . Percent inhibition of macrophages at 200 µg/mL was investigated to be 47 ± 2.34%; when a least concentration applied, the percent inhibition was reported as 3.6 ± 1.1%. IC50 values calculated using table curve was found to be significantly higher (i.e. >200 µg/mL). also indicates the percent hemolysis induced by bioinspired IONPs. Hemolytic activity is based on the release of hemoglobin, which is induced by the test sample. If a given sample is hemolytic, it will cause rupturing of RBCs, which results in hemoglobin release. This hemoglobin release, which has been resulted after incubation of erythrocyte suspension with the test sample (IONPs), can be quantified with the help of a spectrophotometer; hence the lysis of RBCs is quantified. Hemolytic assay was carried out at different concentrations. Median lethal concentration was investigated at >200 µg/mL. According to “American Society for Testing and Materials Designation,” substances with >5% hemolysis are hemolytic, 2–5% slightly hemolytic while <2% non-hemolytic (Citation57). From our results, it can be inferred that bioinspired IONPs are non-hemolytic at low concentrations.
In the results presented in , it is evident that bioinspired IONPs are more toxic to all cells except human cells as indicated by their biocompatibility. Generally, the human cells such as macrophages have an inherent capacity to deal with the ROS generated from any source. Therefore, the reactive oxygen species generated at low concentrations are not cytotoxic to human cells such as RBCs and macrophages unless the concentration is increased beyond limit (Citation58). A detailed proposed mechanism for the IONPs-based cytotoxicity has been indicated in .
Figure 8. Different proposed mechanisms of IONPs-mediated toxicity. (A) Extracellular ROS generations (which can easily penetrate inside the cell) followed by their interference with nuclear material, leading to genotoxicity. (B) IONPs are internalized by receptor-mediated endocytosis, followed by their entry into the lysosomes, where Fe2+ dissolution occurs. (D) The generated ions not only interfere with other proteins, but also make their way into the mitochondria, where they disrupt its function by creating further ROS. (C) Surface defects in IONP’s can result in rupturing of cellular membranes. The mechanism have been developed through the work of earlier researchers.
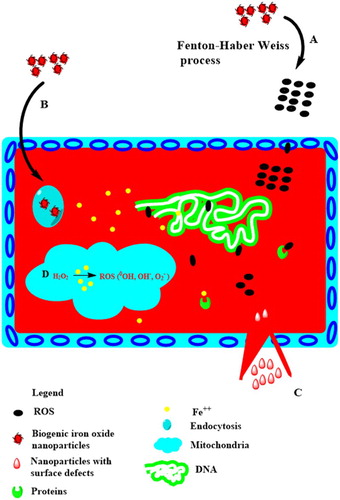
3.5. Enzyme inhibition assays
Protein kinase inhibitors represent a fascinating area of research with respect to the treatment therapies for cancer (Citation59). These enzymes are responsible for the phosphorylation of serine-threonine and tyrosine residues that play a pivotal role in cellular division, differentiation, apoptosis and other metabolic processes. Deregulated phosphorylations at serine-threonine and tyrosine residues can lead to the progression of tumor. Hence, chemical entities having a capacity to inhibit PK enzymes are extremely valuable. In the Streptomyces 85E strain, protein kinases play a pivotal role in the formation of hyphae. Same strain was employed to investigate the protein kinase inhibition potential (Citation60) of bioinspired IONPs. Following disc diffusion assay, significant zones of inhibitions were observed (i.e. 39–17 mm) from highly concentrated to the least concentrated samples (2000–62.5 µg/mL), which relates to the significant PK inhibition activity of the biogenically synthesized IONPs. Therefore, a potent signal transductor inhibitor is identified in the form of bioinspired IONPs. Results are summarized in , while a detailed mechanism of protein kinase inhibition is indicated in . In addition, the bioinspired IONPs indicated insignificant alpha amylase inhibition (IC50 > 200 µg/mL). The alpha amylase enzyme is involved in the metabolism of carbohydrates by converting them into glucose and therefore it is linked to the postprandial blood glucose excursion in a person suffering from diabetes. Hence, the conversion to glucose from starch can be blocked by inhibition of alpha amylase enzyme and therefore it represents a key area of diabetes research (Citation61, Citation62)
Figure 10. Proposed role of biosynthesized IONPs as protein kinase inhibitor for tumor growth prevention: (A) the activity of protein kinase in response to growth stimuli, which is followed by activation of growth factors by phosphorylation activity of protein kinase; and (B) by interfering with the protein kinase enzymes, protein kinases could not initiate the signaling cascade responsible for the proliferation of the cell, hence division is inhibited.
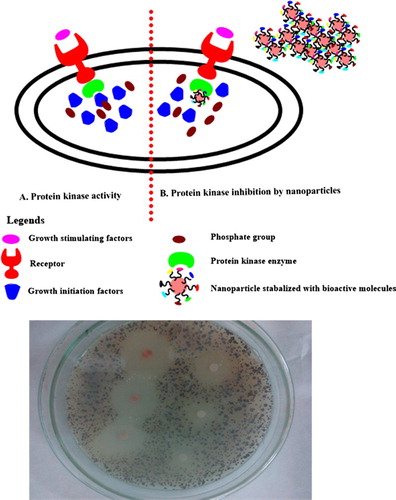
3.6. Antioxidant activities
indicates the antioxidant activities performed on the biosynthesized IONPs engineered via the aqueous leaf extracts of S. thea. Free radical scavenging activity was performed using DPPH. Moderate percent radical scavenging was observed at higher concentrations of 200 µg/mL (i.e. 54.08 ± 2.3%). Free radical scavenging was reported for all of the applied concentrations (200–1 µg/mL). IC50 for the DPPH activity was calculated as 339 µg/mL. DPPH is based on the formation of yellowish diphenyl picrylhydrazine molecule when DPPH moiety is reduced by accepting a hydrogen or electron from a donor. TAC is based on the conversion of Mo(VI) to Mo(V) and a subsequent formation of greenish Mo(V)-phosphate complex having maximum absorption at 695 nm (Citation36). Maximum antioxidants in terms of ascorbic acid equivalents/mg were found as 14.3 ± 1.01 for the tested concentration of 200 µg/mL. TAC indicates the ability of a test compound to quench ROS. Reducing power assay was performed and highest reducing power (15.24 ± 1.45) was investigated for the test concentration of 200 µg/mL. Reducing power assay includes the investigation of reductones that are related with anti-oxidation potential by donating H-atom that results in the breakage of free radical chain (Citation63). Since aqueous leaf extracts of S. thea were used as a reducing, capping as well stabilizing agent, it can be inferred that some bioactive components which are retained as capping agents have contributed to the antioxidant activities of the biogenic IONPs.
4. Conclusion
Successful biosynthesis of highly crystalline, pure iron oxide maghemite (Fe2O3) phase nanoparticles has been demonstrated using an eco-friendly, economical, simple and fast method based on green chemistry approach. A comprehensive study on their characterizations and pharmacognostic properties has been carried out. Our results indicate impressive biological properties of the biosynthesized IONPs. Biosynthesized IONPs have been found as more cytotoxic to the axenic cultures of L. tropica (promastigotes / amastigotes) and brine shrimps while relatively nontoxic to the human RBCs and macrophages at low concentrations, indicating their safe nature and futuristic role in antimicrobial and antileishmanial therapies. In addition, significant inhibition of protein kinase enzyme suggests that biosynthesized IONPs can be extremely effective for treatment of cancer via inhibition of protein kinase enzymes. However, to harvest the true benefits of these biologically synthesized nanomaterials, further in vitro and in vivo studies are suggested by involving animal models that can help in fabrication of iron oxide nanomaterials with highest degree of biocompatibility. To further enhance the nanoparticle biocompatibility, research should be undertaken that involves their coating with several different types of biocompatible polymers such as PEG, Chitosan, Dextran etc. They will not only serve as a vehicle for drug delivery but also will improve their compatibility.
Acknowledgments
The authors are indebted to the staff at Material Research Department (iThemba Labs) and Molecular systematics and applied ethnobotany lab (Department of Biotechnology, Quaid-i-Azam University) for their continuous support and cooperation. Mr Ali Talha Khalil is also affiliated with the Department of Biotechnology, Quaid-i-Azam University, Islamabad, Pakistan. The authors are also indebted to the kind support of Prof. NM Butt. ATK, ZKS and MM conceived the idea. ATK, MO, MA and IU performed the experiments with the assistance of ZKS and MM. ATK and MA drafted the manuscript. MM and ZKS critically reviewed and improved the manuscript.
Disclosure statement
No potential conflict of interest was reported by the authors.
Notes on contributors
Mr. Ali Talha Khalil is a PhD fellow at the Department of Biotechnology, Quaid-i-Azam University, Islamabad, Pakistan. His research work is based on the applications of metal and metal oxide nanoparticles. He is also a fellow of NANOAFNET and UNESCO-UNISA Africa Chair in nanoscience and nanotechnology.
Mr. Muhammad Ovais is a fresh MPhil graduate with research interest in green chemistry and nanosciences.
Mr. Ikram Ullah is a PhD fellow at the Department of Biotechnology, Quaid-i-Azam University, Islamabad, with research interest in using bioinspired nanoparticles for anticancer research.
Dr. Muhammad Ali is an energetic young scientist and assistant professor at the Department of Biotechnology, Quaid-i-Azam University, Islamabad. His research is based on novel treatment strategies using nanoparticles against different diseases.
Dr. Zabta Khan Shinwari is a tenured professor at the Department of Biotechnology, Quaid-i-Azam University, Islamabad, with diverse research interests. He is very well known for his work on the medicinal flora of Pakistan and his work has been cited frequently in peer-reviewed journals.
Dr. Malik Maaza is a professor at the University of South Africa and a well-known scientist in green chemistry. His research work is based on the application of nanoparticles in diverse fields such as medicine and energy storage. His work has been highly cited in international and peer-reviewed journals. He is also a fellow of some of the prestigious societies and associations.
Additional information
Funding
References
- Venugopal, K.; Rather, H.; Rajagopal, K.; Shanthi, M.; Sheriff, K.; Illiyas, M.; Rather, R.; Manikandan, E.; Uvarajan, S.; Bhaskar, M. Synthesis of silver nanoparticles (Ag NPs) for anticancer activities (MCF 7 breast and A549 lung cell lines) of the crude extract of Syzygium aromaticum. J. Photochem. Photobiol. B: Biology. 2017, 167, 282–289.
- Diallo, A.; Ngom, B.; Park, E.; Maaza, M. Green synthesis of ZnO nanoparticles by Aspalathus linearis: structural & optical properties. J. Alloys. Compd. 2015, 646, 425–430.
- Sone, B.; Manikandan, E.; Gurib-Fakim, A.; Maaza, M. Sm2O3 nanoparticles green synthesis via Callistemon viminalis’ extract. J. Alloys. Compd. 2015, 650, 357–362.
- Thovhogi, N.; Park, E.; Manikandan, E.; Maaza, M.; Gurib-Fakim, A. Physical properties of CdO nanoparticles synthesized by green chemistry via Hibiscus sabdariffa flower extract. J. Alloys. Compd. 2016, 655, 314–320.
- Diallo, A.; Beye, A.; Doyle, T.; Park, E.; Maaza, M. Green synthesis of Co3O4 nanoparticles via Aspalathus linearis: physical properties. Green Chem. Lett. Rev. 2015, 8 (3–4), 30–36.
- Khenfouch, M.; Minnis Ndimba, R.; Diallo, A.; KhamLich, S.; Hamzah, M.; Dhlamini, M.; Mothudi, B.; Baitoul, M.; Srinivasu, V.; Maaza, M. Artemisia herba-alba Asso eco-friendly reduced few-layered graphene oxide nanosheets: structural investigations and physical properties. Green. Chem. Lett. Rev. 2016, 9 (2), 122–131.
- Nyangiwe, N.N.; Khenfouch, M.; Thema, F.T.; Nukwa, K.; Kotsedi, L.; Maaza, M. Free-green synthesis and dynamics of reduced graphene sheets via sun light irradiation. Graphene. 2015, 4 (3), 54–61.
- Sone, B.; Diallo, A.; Fuku, X.; Gurib-Fakim, A.; Maaza, M. Biosynthesized CuO nano-platelets: physical properties & enhanced thermal conductivity nanofluidics. Arab. J. Chem. in press. doi:10.1016/j.arabjc.2017.03.004.
- Fuku, X.; Kaviyarasu, K.; Matinise, N.; Maaza, M. Punicalagin green functionalized Cu/Cu2O/ZnO/CuO nanocomposite for potential electrochemical transducer and catalyst. Nanoscale Res. Lett. 2016, 11 (1), 2229.
- Braunschweig, J.; Bosch, J.; Meckenstock, R.U. IONPs in geomicrobiology: from biogeochemistry to bioremediation. N. Biotechnol. 2013, 30 (6), 793–802.
- Gupta, A.K.; Gupta, M. Synthesis and surface engineering of IONPs for biomedical applications. Biomaterials. 2005, 26 (18), 3995–4021.
- Lee, J.H.; Schneider, B.; Jordan, E.K.; Liu, W.; Frank, J.A. Synthesis of complexable fluorescent superparamagnetic IONPs (FL SPIONs) and cell labeling for clinical application. Adv. Mater. 2008, 20 (13), 2512–2516.
- Li, X.-Q.; Elliott, D.W.; Zhang, W.-X. Zero-valent iron nanoparticles for abatement of environmental pollutants: materials and engineering aspects. Crit. Rev. Solid State. 2006, 31 (4), 111–122.
- López-Serrano, A.; Olivas, R.M.; Landaluze, J.S.; Cámara, C. Nanoparticles: a global vision. Characterization, separation, and quantification methods. Potential environmental and health impact. Anal. Methods. 2014, 6 (1), 38–56.
- Siddiqi, K.S.; Ur Rahman, A.; Husen, A. Biogenic fabrication of iron/IONPs and their application. Nanoscale Res. Lett. 2016, 11 (1), 229.
- Rasheed, R.; Meera, V. Synthesis of IONPs coated sand by biological method and chemical method. Procedia Technol. 2016, 24, 210–216.
- Nidhin, M.; Indumathy, R.; Sreeram, K.; Nair, B.U. Synthesis of IONPs of narrow size distribution on polysaccharide templates. Bull. Mater. Sci. 2008, 31 (1), 93–96.
- Mahdavi, M.; Namvar, F.; Ahmad, M.B.; Mohamad, R. Green biosynthesis and characterization of magnetic iron oxide (Fe3O4) nanoparticles using seaweed (Sargassum muticum) aqueous extract. Molecules. 2013, 18 (5), 5954–5964.
- Shahwan, T.; Sirriah, S.A.; Nairat, M.; Boyacı, E.; Eroğlu, A.E.; Scott, T.B.; Hallam, K.R. Green synthesis of iron nanoparticles and their application as a Fenton-like catalyst for the degradation of aqueous cationic and anionic dyes. Chem. Eng. J. 2011, 172 (1), 258–266.
- Smuleac, V.; Varma, R.; Sikdar, S.; Bhattacharyya, D. Green synthesis of Fe and Fe/Pd bimetallic nanoparticles in membranes for reductive degradation of chlorinated organics. J. Membrane. Sci. 2011, 379 (1), 131–137.
- Kharissova, O.V.; Dias, H.R.; Kharisov, B.I.; Pérez, B.O.; Pérez, V.M.J. The greener synthesis of nanoparticles. Trends. Biotechnol. 2013, 31 (4), 240–248.
- Thema, F.; Beukes, P.; Gurib-Fakim, A.; Maaza, M. Green synthesis of monteponite CdO nanoparticles by Agathosma betulina natural extract. J. Alloys. Compd. 2015, 646, 1043–1048.
- Ovais, M.; Khalil, A.T.; Raza, A.; Khan, M.A.; Ahmad, I.; Islam, N.U.; Saravanan, M.; Ubaid, M.F.; Ali, M.; Shinwari, Z.K. Green synthesis of silver nanoparticles via plant extracts: beginning a new era in cancer theranostics. Nanomedicine. 2016, 11 (23), 3157–3177.
- Sharma, J.K.; Srivastava, P.; Akhtar, M.S.; Singh, G.; Ameen, S. α-Fe2O3 hexagonal cones synthesized from the leaf extract of Azadirachta indica and its thermal catalytic activity. New J. Chem. 2015, 39 (9), 7105–7111.
- Ahmmad, B.; Leonard, K.; Islam, M.S.; Kurawaki, J.; Muruganandham, M.; Ohkubo, T.; Kuroda, Y. Green synthesis of mesoporous hematite (α-Fe2O3) nanoparticles and their photocatalytic activity. Adv. Powder. Technol. 2013, 24 (1), 160–167.
- Hoag, G.E.; Collins, J.B.; Holcomb, J.L.; Hoag, J.R.; Nadagouda, M.N.; Varma, R.S. Degradation of bromothymol blue by “greener” nano-scale zero-valent iron synthesized using tea polyphenols. J. Mater. Chem. 2009, 19 (45), 8671–8677.
- Naseem, T.; Farrukh, M.A. Antibacterial activity of green synthesis of iron nanoparticles using Lawsonia inermis and Gardenia jasminoides leaves extract. J. Mater. Chem. 2015, 1–7. doi:10.1155/2015/912342.
- Huang, L.; Weng, X.; Chen, Z.; Megharaj, M.; Naidu, R. Synthesis of iron-based nanoparticles using oolong tea extract for the degradation of malachite green. Spectrochim. Acta Mol. Biomol. Spectrosc. 2014, 117, 801–804.
- Fatima, H.; Khan, K.; Zia, M.; Ur-Rehman, T.; Mirza, B.; Haq, I.-U. Extraction optimization of medicinally important metabolites from Datura innoxia Mill.: an in vitro biological and phytochemical investigation. BMC. Complement. Altern. Med. 2015, 15 (1), 1–18. doi:10.1186/s12906-015-0891-1.
- Ahmad, K.; Khalil, A.T.; Yusra ;Somayya, R. Antifungal, phytotoxic and hemagglutination activity of methano-lic extracts of Ocimum basilicum. J. Tradit. Chin. Med. 2016, 36 (6), 794–798.
- Ali, A.; Ambreen, S.; Javed, R.; Tabassum, S.; Ul Haq, I.; Zia, M. ZnO nanostructure fabrication in different solvents transforms physio-chemical, biological and photodegradable properties. Mater. Sci. Eng. C. 2017, 74, 137–145.
- Chtita, S.; Ghamali, M.; Hmamouchi, R.; Elidrissi, B.; Bourass, M.; Larif, M.; Bouachrine, M.; Lakhlifi, T. Investigation of antileishmanial activities of acridines derivatives against promastigotes and amastigotes form of parasites using quantitative structure activity relationship analysis. Adv. Phys. Chem. 2016. http://dx.doi.org/10.1155/2016/5137289
- Khan, I.; Ahmad, K.; Khalil, A.T.; Khan, J.; Khan, Y.A.; Saqib, M.S.; Umar, M.N.; Ahmad, H. Evaluation of antileishmanial, antibacterial and brine shrimp cytotoxic potential of crude methanolic extract of herb Ocimum basilicum (Lamiacea). J. Tradit. Chin. Med. 2015, 35 (3), 316–322.
- Malagoli, D. A full-length protocol to test hemolytic activity of palytoxin on human erythrocytes. Invertebr. Surviv. J. 2007, 4 (2), 92–94.
- de Almeida, M.C.; Silva, A.C.; Barral, A.; Barral Netto, M. A simple method for human peripheral blood monocyte isolation. Memorias do Instituto Oswaldo Cruz. 2000, 95 (2), 221–223.
- Jafri, L.; Saleem, S.; Ullah, N.; Mirza, B. In vitro assessment of antioxidant potential and determination of polyphenolic compounds of Hedera nepalensis K. Koch. Arab. J. Chem. 2014, 5, 002. doi.org/10.1016/j.arabjc.2014.05.002
- Javed, R.; Usman, M.; Tabassum, S.; Zia, M. Effect of capping agents: structural, optical and biological properties of ZnO nanoparticles. Appl. Surf. Sci. 2016, 386, 319–326.
- Zak, A.K.; Razali, R.; Majid, W.; Darroudi, M. Synthesis and characterization of a narrow size distribution of zinc oxide nanoparticles. Int. J. Nanomed. 2011, 6, 1399–1403.
- Darroudi, M.; Sabouri, Z.; Oskuee, R.K.; Zak, A.K.; Kargar, H.; Hamid, M.H.N.A. Green chemistry approach for the synthesis of ZnO nanopowders and their cytotoxic effects. Ceram. Int. 2014, 40, (3), 4827–4831.
- Shen, C.J.; Chen, C.K.; Lee, S.S. Polar constituents from Sageretia thea leaf characterized by HPLC-SPE-NMR assisted approaches. J. Chin. Chem. Soc. 2009, 56 (5), 1002–1009.
- Chung, S.-K.; Kim, Y.-C.; Takaya, Y.; Terashima, K.; Niwa, M. Novel flavonol glycoside, 7-O-methyl mearnsitrin, from Sageretia theezans and its antioxidant effect. J. Agric. Food Chem. 2004, 52 (15), 4664–4668.
- Xu, L.; Yang, X.; Li, B. Chemical constituents of Sageretia theezans Brongn. Zhongguo Zhong yao za zhi = Zhongguo zhongyao zazhi = China Journal of Chinese Materia Medica. 1994, 19 (11), 675–702.
- Zhao, B.; Wang, Y.; Guo, H.; Wang, J.; He, Y.; Jiao, Z.; Wu, M. Iron oxide (III) nanoparticles fabricated by electron beam irradiation method. Mater. Sci. Polan. 2007, 25 (4), 1143–1148.
- Islam, M.S.; Kusumoto, Y.; Abdulla-Al-Mamun, M. Novel rose-type magnetic (Fe3O4, γ-Fe2O3 and α-Fe2O3) nanoplates synthesized by simple hydrothermal decomposition. Mater. Lett. 2012, 66 (1), 165–167.
- Ruíz-Baltazar, A.; Esparza, R.; Rosas, G.; Pérez, R. Effect of the surfactant on the growth and oxidation of iron nanoparticles. J. Nanomater. 2015, 16 (1), 202.
- Brown, G. The X-ray identification and crystal structures of clay minerals;Mincerology Society London: London, 1961.
- Zhang, W.; Zeng, Y.; Xiao, N.; Hng, H.H.; Yan, Q. One-step electrochemical preparation of graphene-based heterostructures for Li storage. J. Mater. Chem. 2012, 22 (17), 8455–8461.
- Benelmekki, M.; Martinez, L.M. Magnetophoresis of IONPs: a tool for synthesis monitoring and biomagnetic applications. Nanotechnology, 2013, 7. Diagnostics and Therapeutics. Stadium press LLC, USA.
- Maiti, D.; Manju, U.; Velaga, S.; Devi, P.S. Phase evolution and growth of IONPs: effect of hydrazine addition during sonication. Crystl Growth. Desi. 2013, 13 (8), 3637–3644.
- Khayatian, G.; Hassanpoor, S.; Azar, A.R.; Mohebbi, S. Spectrophotometric determination of trace amounts of uranium (VI) using modified magnetic IONPs in environmental and biological samples. J. Braz. Chem. Soc. 2013, 24 (11), 1808–1817.
- Jubb, A.M.; Allen, H.C. Vibrational spectroscopic characterization of hematite, maghemite, and magnetite thin films produced by vapor deposition. ACS Appl. Mater. Interfaces. 2010, 2 (10), 2804–2812.
- De Faria, D.; Venâncio Silva, S.; De Oliveira, M. Raman microspectroscopy of some iron oxides and oxyhydroxides. J. Raman Spectrosc. 1997, 28 (11), 873–878.
- Behera, S.S.; Patra, J.K.; Pramanik, K.; Panda, N.; Thatoi, H. Characterization and evaluation of antibacterial activities of chemically synthesized IONPs. World J. Nano Sci. Eng. 2012, 2 (4), 196–200.
- Gasmalla, H.B.; Idris, A.M.; Shinger, M.I.; Qin, D.; Shan, D.; Lu, X. Balanites aegyptiaca oil synthesized IONPs: characterization and antibacterial activity. J. Biomater Nanobiotechnol. 2016, 7 (3), 154–165.
- Li, Y.; Zhang, W.; Niu, J.; Chen, Y. Mechanism of photogenerated reactive oxygen species and correlation with the antibacterial properties of engineered metal-oxide nanoparticles. Acs Nano. 2012, 6 (6), 5164–5173.
- Jebali, A.; Kazemi, B. Nano-based antileishmanial agents: a toxicological study on nanoparticles for future treatment of cutaneous leishmaniasis. Toxicol. In Vitro. 2013, 27 (6), 1896–1904.
- Aula, S.; Lakkireddy, S.; Swamy, A.; Kapley, A.; Jamil, K.; Tata, N.R.; Hembram, K. Biological interactions in vitro of zinc oxide nanoparticles of different characteristics. Mater. Res. Express. 2014, 1 (3), 035041.
- Prach, M.; Stone, V.; Proudfoot, L. Zinc oxide nanoparticles and monocytes: impact of size, charge and solubility on activation status. Toxicol. Appl. Pharmacol. 2013, 266 (1), 19–26.
- Yao, G.; Sebisubi, F.M.; Voo, L.Y.C.; Ho, C.C.; Tan, G.T.; Chang, L.C. Citrinin derivatives from the soil filamentous fungus penicillium sp. H9318. J. Braz. Chem. Soc. 2011, 22 (6), 1125–1129.
- Waters, B.; Saxena, G.; Wanggui, Y.; Kau, D.; Wrigley, S.; Stokes, R.; Davies, J. Identifying protein kinase inhibitors using an assay based on inhibition of aerial hyphae formation in streptomyces. J. Antibiot. 2002, 55 (4), 407–416.
- Ali, S.; Stone, M.; Peters, J.; Davies, M.; Khunti, K. The prevalence of co-morbid depression in adults with Type 2 diabetes: a systematic review and meta-analysis. Diabetic. Med. 2006, 23 (11), 1165–1173.
- Dineshkumar, B.; Mitra, A.; Mahadevappa, M. Antidiabetic and hypolipidemic effects of mahanimbine (carbazole alkaloid) from Murraya koenigii (rutaceae) leaves. Int. J. Phytomed. 2010, 2 (1), 22–30.
- Abdel-Hameed, E.-S.S. Total phenolic contents and free radical scavenging activity of certain Egyptian Ficus species leaf samples. Food Chem 2009, 114 (4), 1271–1277.