ABSTRACT
In this research, the ortho C-acylation of some phenol and naphthol derivatives was catalyzed by ZnCl2, supported on multi-walled carbon nanotubes as a new nanocomposite Lewis acid catalyst. The reaction was performed under solvent-free and microwave conditions. In the presence of this heterogeneous catalyst, a variety of phenol and naphthol moieties were converted to C-acylated compounds with full selectivity on the ortho position. Also, this method is an effective approach for acylation of catechol, resorcinol, hydroquinone and their derivatives. In this process, the products were obtained in short reaction times and excellent yields.
GRAPHICAL ABSTRACT
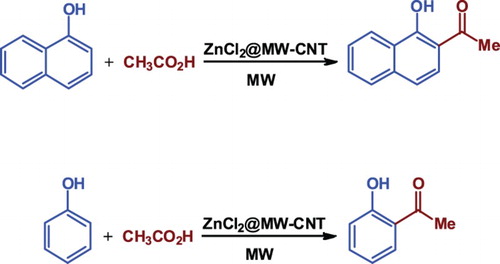
Introduction
The ortho C-acylated phenol and naphthol derivatives are important structural motifs found in a diversity of bioactive molecules, especially in the synthesis of natural compounds. Some examples are shown in . The ortho C-acylated phenols are also important synthons in organic chemistry (Citation1–5). They serve as protecting groups (Citation6), polyfunctional molecules such as nucleosides, carbohydrates and peptides (Citation7), or modified peptides such as isoesters (Citation8) and steroids (Citation9–12).
The three existing major synthetic pathways available for the ortho C-acylation reaction are: (i) the Lewis acid catalyzed Friedel–Crafts acylation (Citation13, Citation14), (ii) ortho-acylation of phenols with aryl aldehydes as precursor and copper as catalyst (Citation15) and (iii) C–H activation of ketone moieties using [bis(trifluoroacetoxy)iodo]benzene as an oxidant (Citation16). The known Friedel–Crafts reaction via Lewis acid catalyst suffers from the formation of undesired p-substituted products, especially when bulky acyl groups need to be introduced. The synthesis of some ketones with sulfur and nitrogen as functional groups is also limited in this method (Citation17, Citation18). The use of new hybrid Lewis acid catalysts in achieving clean reactions with good selectivity and performance under the better reaction conditions has yet to be investigated.
Since carbon nanotubes (CNTs) were discovered in 1991 (Citation19), they have attracted significant interest due to their unique structure and properties. Due to the thermal and chemical stability of multi-walled carbon nanotubes (MWCNTs), it was employed in organic synthesis and science of catalyst as support. The chemical modification of CNTs is a technique for enhancing their applications (Citation20–22).
It was previously found by our research group that ZnCl2 has excellent catalytic activity in the acylation of phenolic compounds by carboxylic acids as substrate (Citation23, Citation24). Our first efforts to heterogenize the catalyst for a greener process led to the support of zinc chloride on the surface of Al2O3 as a solid-phase catalyst (Citation25). Because the alumina has a hydrophilic surface, the stability of the zinc chloride on the surface of this support suffered from faster deactivation. The benefit of MWCNTs as support for zinc chloride is the high surface area and thereupon high activation of the final catalyst. Also, the recyclability of the ZnCl2@MWCNTs is a key advantage of this method. As a continuation of our previous works on the application of MWCNTs in organic reactions (Citation26–28), the ortho-acylation of hydroxyaryl compounds such as phenol and naphthol derivatives was investigated in the presence of carboxylic acids and MWCNTs@ZnCl2 as a new nanocomposite heterogeneous catalyst. The object of the present study is to provide a novel and improved process for the acylation reaction without disadvantages such as low yield, long reaction time, homogeneous catalyst and tedious workup.
Experimental
Materials and methods
The phenol derivatives were purchased from the Merck chemical company with 97–99% purity. The α-naphthol and quinizarin were purchased from the Fluka Chemical Company at 99% purity. The acetic acid and the solvents used in this work were purchased from the Sigma Company at analytical grade with 99% purity. MWCNTs were purchased from Shenzhen Nanotechnology Co., Ltd. (China). The purity of the MWCNTs was 92–95% and their diameters and lengths ranges were between 20–40 nm and 5–15 μm, respectively. The microwave-assisted procedures were carried out in a microwave oven (Milestone LAVIS-1000 MultiQUANT Basic Microwave) operated at 450 W. IR spectra were recorded with a KBr pellet on a Perkin-Elmer 781 Spectrophotometer and an Impact 400 Nicolet FT-IR Spectrophotometer. The 1H NMR and 13C NMR spectra were provided in CDCl3 as a solvent with 400 MHz Bruker Spectrometer. The reactions were carried out in microwave oven specially designed for the organic synthesis (Milestone LAVIS 1000 Basic Microwave). Melting points were recorded with a Yanagimoto micro melting point apparatus. The purity of the substrates and reaction progression were determined by thin-layer chromatography (TLC) on silicagel Polygram SILG/UV 254 plates.
Catalyst preparation
In a typical experiment, a mixture of MWCNTs (1 g) in tetrahydrofuran (THF) (50 mL) was sonicated for 30 min. After dispersion was completed, the solid zinc chloride (0.25 g) was added to the reaction vessel and stirred at room temperature for 24 h. After this time, the reaction mixture was filtered, washed with THF (3 × 20 mL), dried in vacuum and the resulted nanocomposite was used as the catalyst for the acylation reaction.
Typical procedure for the acylation reaction by ZnCl2@MWCNTs nanocomposite
In a typical reaction, in a reaction vessel, hydroquinone (0.1 g, 0.95 mmol), acetic acid (0.05 ml, 1.2 mmol) and 0.02 g of ZnCl2@MWCNTs as catalyst (3.0 mol% of ZnCl2) were combined together and reacted for 70 s under microwave irradiation (450 W). In all of the reactions, during microwave irradiation, the temperature of the reaction mixture reached about 40°C. After cooling the reaction mixture to room temperature, ethyl acetate (15 ml) was added. The catalyst was filtered off and thoroughly washed with ethyl acetate (3 × 10 mL), then dried under vacuum. The organic solvent was washed with aqueous NaHCO3 (20 mL), dried over MgSO4, filtered, and the solvent evaporated to give desired product. The product was purified by column chromatography on silicagel. The products were characterized by spectroscopic and physical techniques by being consistent with previously reported data (Citation29–32).
Results and discussions
In the present study, the performance and regioselectivity of ZnCl2@MWCNTs nanocomposite as a catalyst was evaluated in the acylation reaction. Reactions were carried out in the absence of solvent, to afford the corresponding ortho-acylated hydroxyaryl compounds, in excellent yields. The purpose of these acylation reactions is to (i) improve CNTs interaction with dispersion; (ii) allow the grafting of nanoparticles; (iii) use the high adsorption properties of CNTs and (iv) perform chemical treatments on nanotubes.
Description of the catalyst
CNTs have been used as a support for anchoring some substances in order to give them additional functional properties, such as activity and surface area (Citation33). Interlayer distance in MWCNT is close to interplant graphite distance 3.4 Å and varies in the range of ±0.2 Å (Citation34–37). Thus, two-atomic molecules cannot penetrate into the MWCNTs wall and adsorption takes place at the external surface of MWCNTs.
Zinc (II) complexation of MWCNTs, which involves constructive rehybridization of the external π-system with the vacant zinc dπ orbitals, leads to the formation of advanced nanomaterial based on the catalytic effect of zinc and high surface area of MWCNTs. This π-bond mode of bonding is quite distinct from the modification by conventional covalent σ-bond with a creation of sp3 carbon centers on the surface of CNTs () (Citation38–41).
The ZnCl2@MWCNTs nanocomposite was examined by powder X-ray diffraction (XRD) and Fourier transform infrared spectrometer (FT-IR), and indicated successful bonding of ZnCl2 onto the internal surface of MWCNTs and has the capability for use as a Lewis acid catalyst.
In , the FT-IR spectra for MWCNTs and ZnCl2@MWCNTs are shown as (a) and (b), respectively. Comparison between the FT-IR spectra of the target catalyst with that of the bare MWCNTs reveals the adsorption of zinc dichloride on the surface of the MWCNTs. According to this figure, three bands at 517, 734 and 982 cm−1 were resulted from Zn–Cl and Zn–C bonds that are not included in the spectra of crude MWCNTs. A broad and weak band at 3200–3500 cm−1 was due to the negligible moisture of KBr that included in both of spectra in this figure.
The XRD pattern of both crude MWCNTs and ZnCl2 supported on it, demonstrated typical reflections of 2θ = 25° and 43°. After zinc chloride anchored on MWCNTs, the diffraction peak intensity of modified CNTs was decreased (). Because ZnCl2 dispersed on the surfaces of the MWCNTs, there were no signals in the XRD pattern related to the zinc chloride.
Using salt of ZnCl2 as a catalyst in this reaction has some disadvantages such as aggregation and deactivation of zinc chloride, poisoning of the products with zinc, difficult reaction workup and finally, the catalyst is not recyclable from the reaction media (Citation23). To alleviate the aforementioned problems, Al2O3 was used as a solid and inactive support by our research group (Citation25). The ZnCl2@Al2O3 as a resulted heterogeneous catalyst, kept the zinc chloride from aggregation, and thereupon deactivation of catalyst did not occur.
The SEM image of MWCNTs after the ZnCl2 treatment is shown in . This image illustrates that the MWCNTs were covered by a layer of external matter (zinc chloride), resulting in thickened MWCNTs bundles and a denser network of nanotubes.
The BET analysis for the ZnCl2@MWCNTs was carried out and the obtained result was 262 m2/g for the surface area of catalyst. Moreover, the surface of the heterogeneous catalyst is larger than the crude ones (about 36 m2/g) and indicates the zinc chloride anchored on the surface of the nanotubes.
It is clear that the activity of the resulted heterogeneous catalyst depends on the value of specific surface area of solid support. Therefore, the MWCNTs was used as chemically inactive support having high surface area and being a lipophilic material. In this way, the ZnCl2@MWCNTs catalyst can be easily separated without poisoning of the products and also, the amounts of loaded heterogeneous catalyst can be lower than the amounts in the previously reported method.
Catalytic studies
In this research, we investigated the reactivity of ZnCl2@MWCNTs as a novel catalyst for direct ortho-acylation of hydroquinone (as a phenol derivative) and 1-naphthol as a naphthol derivative with acetic acid under microwave irradiation ().
Figure 6. The C-acylation of phenol and naphthol derivatives with acetic acid under microwave irradiation.
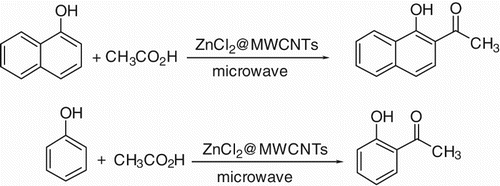
In order to show the advantage of this study, the obtained results were compared with the recently reported results in . The comparison of our results with others was carried out on the basis of reaction conditions, reaction time and the obtained yields percentage. Furthermore, in order to understand the effect of MWCNTs as a support, the reaction was occurred in the presence of same amount of ZnCl2 salt (3 mol%), as a solid catalyst in the optimum reaction conditions. The result of this test is provided in , entry 6, and indicated that the yield of the reaction only reached 32% after 12 min.
Table 1. Comparison of o-hydroxyacetophenone synthesis by ZnCl2@MWCNTs with literature reported methods.
In continuation of this work, ZnCl2@MWCNTs was applied as catalyst for ortho-acylation of various phenol and naphthol derivatives in the presence of acetic acid under microwave conditions (). The obtained results are summarized in .
Table 2. Acylation of phenol and naphthol derivatives in the presence of ZnCl2@MWCNTs.a
As shown in , in some of the reported works, the para-acylated products are also available as a side reaction, while we obtained only the ortho C-acylated product and no para-acylated was obtained. We have now found that, by using lipophilic MWCNTs, deactivation of the catalyst is canceled as expected and the reaction is performed faster than the other-mentioned catalysts.
With attention to entry 13, , when two ortho positions are occupied by methyl groups, no acylated product is obtained. Also, in entries 8–11, the electron-withdrawing groups, reduced the reactivity of the substrates and thus, even at the high power of microwave irradiation, the reaction did not afford any product. In other entries, ortho-acylated compounds were chemoselectively achieved in high yields.
The scope and limitation of the ZnCl2@MWCNTs catalyst in acylation reaction were studied with other organic acids. The nanocomposite was used as Lewis acid catalyst in the acylation of hydroquinone, by propanoic, butanoic and pentanoic acid under modified reaction conditions. These reactions, as expected, produced ortho-acylated compounds in high yields and short reaction times. The obtained results were summarized in . It is notable that the ZnCl2 as the catalytic active sites on the surface can be deactivated and poisoned by a trace of water via coordination with H2O and lastly removed from the surface of the support. However, the resulted water from the reaction process cannot be adsorbed on lipophilic surface of MWCNTs.
Table 3. Ortho-acylation of hydroquinone with various organic acids.a
In order to assess the reliability and validity of this method, the reaction of hydroquinone and acetic acid in the presence of ZnCl2@MWCNTs at optimum reaction conditions was carried out and repeated 10 times. The resulted data were used for calculating the average value and standard deviation where the values of these parameters were 95.8 and 1.762, respectively.
Regioselectivity is essential strategy for this method and is achieved in the key step of zinc chelating to both oxygen moieties from phenol and carboxylic acid substrates and consequently the zinc atom is located at the nearest position of the phenol moiety. Furthermore, nucleophilic addition of phenol ring to the carboxylic acid substrate occurs from the ortho position and phenol joins to the carbonyl group. Finally, the removal of a water molecule produces the desired products.
For assessing the possibility of recovering and recycling of the catalyst, the reaction of hydroquinone and acetic acid in the presence of ZnCl2@MWCNTs catalyst was carried out under the optimized reaction conditions. After completion of the reaction, the mixture was diluted with EtOAc (5 mL) and the solid catalyst was centrifuged to separate from the reaction media. The solid catalyst was dried under reduced pressure and reused seven times without considerable loss of its catalytic activity (from 97% to 85% of product yield). The decreasing of the catalyst performance could occur due to inactivation of ZnCl2 species as active sites or ZnCl2 leakage from solid MWCNTs support.
Conclusion
Zinc chloride was successfully anchored on the surface of MWCNTs and the resulted nanocomposite was characterized by FT-IR, XRD, Brunauer–Emmett–Teller (BET) and scanning electron microscope (SEM) techniques. The prepared ZnCl2@MWCNTs was used as a catalyst in the microwave acylation reaction of phenol and naphthol derivatives toward synthesis of ortho-aryl alkyl ketones under solvent-free conditions. This procedure has some advantages in competition with the previously reported methods, such as high regioselectivity, high yields of desired products, short reaction times, and the elimination of solvents and simple workup.
Disclosure statement
No potential conflict of interest was reported by the authors.
Notes on contributors
Mohsen Moradian received his B.Sc. degree in Chemistry from the University of Yazd, in 2002 and then his M.Sc. degree in Organic chemistry from the same university in 2005. He received his Ph.D. degree in Organic Chemistry from the University of Kashan (Kashan, Iran) in 2013. He joined the Department of Organic Chemistry at the University of Kashan in 2013. His research interest includes the application of new methods and catalysts in organic synthesis, electrosynthesis, investigation of organic compounds for biological, pharmaceutical, and environmental applications.
Atefeh Amini received her B.Sc. degree in Chemistry from the University of Isfahan, in 2008 and then his M.Sc. degree in organic chemistry from the same university in 2012.
Hossein Naeimi was born in 1962 in Khaledabad of Natanz, Iran. He received his M.Sc. degree in 1991 from Shahid Beheshti University and Ph.D. degree in 1998 from Shiraz University. After working at Government College, he became Assistant Professor at University of Kashan and subsequently became Associate Professor in 2004 and Professor in 2008. His research interests include synthesis of Schiff bases, heterocycles and anthraquinones.
Additional information
Funding
References
- Tu, Q.-D.; Li, D.; Sun, Y.; Han, X.-Y.; Yi, F.; Sha, Y.; Ren, Y.-L.; Ding, M.-W.; Feng, L.-L.; Wan, J. Bioorg. Med. Chem. 2013, 21 (11), 2826–2831. doi: 10.1016/j.bmc.2013.04.003
- Shang, Y.; Wang, C.; He, X.; Ju, K.; Zhang, M.; Yu, S.; Wu, J. Tetrahedron. 2010, 66 (50), 9629–9633. doi: 10.1016/j.tet.2010.09.095
- Yang, L.; Lei, H.; Mi, C.-G.; Liu, H.; Zhou, T.; Zhao, Y.-L.; Lai, X.-Y.; Li, Z.-C.; Song, H.; Huang, W.-C. Bioorg. Med. Chem. Lett. 2011, 21 (18), 5389–5392. doi: 10.1016/j.bmcl.2011.07.007
- Madabhushi, S.; Chinthala, N.; Vangipuram, V.S.; Godala, K.R.; Jillella, R.; Mallu, K.K.R.; Beeram, C.R. Tetrahedron Lett. 2011, 52 (46), 6103–6107. doi: 10.1016/j.tetlet.2011.09.019
- Stubbing, L.A.; Li, F.F.; Furkert, D.P.; Caprio, V.E.; Brimble, M.A. Tetrahedron. 2012, 68 (34), 6948–6956. doi: 10.1016/j.tet.2012.05.115
- Wuts, P.G.M.; Greene, T.W. Greene’s Protective Groups in Organic Synthesis, 4th ed.;Wiley-Interscience: New York, 2006.
- Bodanszky, M. Side Reactions in Peptide Synthesis. In Principles of Peptide Synthesis; Springer Verlag: Berlin, 1993, 169–214.
- Magrath, J.; Abeles, R.H. J. Med. Chem. 1992, 35 (23), 4279–4283. doi: 10.1021/jm00101a004
- Ishihara, K.; Ohara, S.; Yamamoto, H. Science. 2000, 290 (5494), 1140–1142. doi: 10.1126/science.290.5494.1140
- Leng, Y.; Wang, J.; Zhu, D.; Ren, X.; Ge, H.; Shen, L. Angew. Chem. Int. Ed. Engl. 2009, 121 (1), 174–177. doi: 10.1002/ange.200803567
- Ohshima, T.; Iwasaki, T.; Maegawa, Y.; Yoshiyama, A.; Mashima, K. J. Am. Chem. Soc. 2008, 130 (10), 2944–2945. doi: 10.1021/ja711349r
- Sakakura, A.; Kawajiri, K.; Ohkubo, T.; Kosugi, Y.; Ishihara, K. J. Am. Chem. Soc. 2007, 129 (47), 14775–14779. doi: 10.1021/ja075824w
- Rappoport, Z. The Chemistry of Phenols; Wiley-Interscience: Chichester, 2003.
- Tyman, J.H.P. Synthetic and Natural Phenols; Elsevier Science: Amsterdam, 1996, Vol. 52.
- Jiang, P.; Zhu, C.N.; Zhang, Z.L. Biomaterials. 2012, 33, 5130–5135. doi: 10.1016/j.biomaterials.2012.03.059
- Mo, F.; Trzepkowski, L.J.; Dong, G. Angew. Chem. Int. Ed. Engl. 2012, 124 (52), 13252–13256. doi: 10.1002/ange.201207479
- Miller, J.A. J. Org. Chem. 1987, 52 (2), 322–323. doi: 10.1021/jo00378a044
- Padró, C.L.; Rey, E.A.; González Peña, L.F.; Apesteguía, C.R. Micropor. Mesopor. Mat. 2011, 143 (1), 236–242. doi: 10.1016/j.micromeso.2011.03.005
- Iijima, S. Nature 1991, 354 (6348), 56–58. doi: 10.1038/354056a0
- Eitan, A.; Jiang, K.; Dukes, D.; Andrews, R.; Schadler, L.S. Chem. Mater. 2003, 15 (16), 3198–3201. doi: 10.1021/cm020975d
- Hill, D.E.; Lin, Y.; Rao, A.M.; Allard, L.F.; Sun, Y.-P. Macromolecules. 2002, 35 (25), 9466–9471. doi: 10.1021/ma020855r
- Lin, Y.; Rao, A.M.; Sadanadan, B.; Kenik, E.A.; Sun, Y.-P. J. Phys. Chem. B. 2002, 106 (6), 1294–1298. doi: 10.1021/jp013501v
- Naeimi, H.; Moradi, L. Catal. Commun. 2006, 7 (12), 1067–1071. doi: 10.1016/j.catcom.2006.04.012
- Varma, R.S.; Namboodiri, V.V. Pure Appl. Chem. 2001, 73 (8), 1309–1313. doi: 10.1351/pac200173081309
- Naeimi, H.; Amini, A.; Moradian, M. Org. Chem. Front. 2014, 1 (4), 415–421. doi: 10.1039/C4QO00031E
- Naeimi, H.; Mohajeri, A.; Moradi, L.; Rashidi, A.M. Appl. Surf. Sci. 2009, 256 (3), 631–635. doi: 10.1016/j.apsusc.2009.08.094
- Naeimi, H.; Mohajeri, A.; Moradi, L.; Rashidi, A. J. Nanosci. Nanotechnol. 2011, 11 (10), 8903–8906. doi: 10.1166/jnn.2011.3454
- Moradi, L.; Mohajeri, A.; Naeimi, H.; Rashidi, A.M. J. Nanosci. Nanotechnol. 2013, 13 (3), 1923–1926. doi: 10.1166/jnn.2013.7104
- Paul, S.; Nanda, P.; Gupta, R.; Loupy, A. Synthesis. 2003, 2003 (18), 2877–2881.
- Stoughton, R.W. J. Am. Chem. Soc. 1935, 57 (1), 202–204. doi: 10.1021/ja01304a056
- Pollock, J.R.A.; Stevens, R. Dictionary of Organic Compounds, 4th ed.;Eyre & Spottiswoode Publishers Ltd.: London. 1965. Eng.
- Gorman, J.J.; Ferguson, B.L.; Nguyen, T.B. Rapid Commun. Mass Spectrom. 1996, 10 (5), 529–536. doi: 10.1002/(SICI)1097-0231(19960331)10:5<529::AID-RCM522>3.0.CO;2-9
- Campidelli, S.; Klumpp, C.; Bianco, A.; Guldi, D.M.; Prato, M. J. Phys. Org. Chem. 2006, 19 (8–9), 531–539. doi: 10.1002/poc.1052
- Salvetat, J.-P.; Bonard, J.-M.; Thomson, N.H.; Kulik, A.J.; Forró, L.; Benoit, W.; Zuppiroli, L. Appl. Phys. A. 1999, 69 (3), 255–260. doi: 10.1007/s003390050999
- Novoselov, K.S.; Geim, A.K.; Morozov, S.V.; Jiang, D.; Zhang, Y.; Dubonos, S.V.; Grigorieva, I.V.; Firsov, A.A. Science. 2004, 306 (5696), 666–669. doi: 10.1126/science.1102896
- Dresselhaus, M.S.; Smalley, R.E.; Dresselhaus, G.; Avouris, P. Carbon Nanotubes: Synthesis, Structure, Properties, and Applications;Springer: Berlin Heidelberg, 2003.
- Banerjee, S.; Hemraj-Benny, T.; Wong, S.S. Adv. Mater. 2005, 17 (1), 17–29. doi: 10.1002/adma.200401340
- Tsai, T.-H.; Yeh, P.-C.; Chen, S.-M.; Ali, M.A.; Al-Hemaid, F.M.A. Electroanalysis. 2014, 26 (5), 971–979. doi: 10.1002/elan.201300625
- Tarlani, A.; Narimani, K.; Mohammadipanah, F.; Hamedi, J.; Tahermansouri, H.; Amini, M.M. Appl. Surf. Sci. 2015, 341,86–91. doi: 10.1016/j.apsusc.2015.02.193
- Omrani, A.; Yen, Y.-C.; Cheng, C.-C.; Chang, F.-C. J. Mol. Struct. 2014, 1056–1057,299–306. doi: 10.1016/j.molstruc.2013.10.053
- Gao, F.; Li, J.; Kang, F.; Zhang, Y.; Wang, X.; Ye, F.; Yang, J. J. Phys. Chem. C. 2011, 115 (23), 11822–11829. doi: 10.1021/jp111831y
- Cao, L.; Zhang, L.; Cui, P. Chem. Heterocycl. Comp. 2004, 40 (5), 635–640. doi: 10.1023/B:COHC.0000037320.27881.27
- Norris, J.F.; Sturgis, B.M. J. Am. Chem. Soc. 1939, 61 (6), 1413–1417. doi: 10.1021/ja01875a027
- Naeimi, H.; Moradi, L. Bull. Chem. Soc. Jpn. 2005, 78 (2), 284–287. doi: 10.1246/bcsj.78.284
- Naeimi, H.; Moradi, L. Russ. J. Org. Chem. 2007, 43 (12), 1757–1759. doi: 10.1134/S1070428007120032
- Corson, B.B.; Heintzelman, W.J.; Schwartzman, L.H.; Tiefenthal, H.E.; Lokken, R.J.; Nickels, J.E.; Atwood, G.R.; Pavlik, F.J. J. Org. Chem. 1958, 23 (4), 544–549. doi: 10.1021/jo01098a012
- Coulthard, C.E.; Marshall, J.; Pyman, F.L. J. Chem. Soc. 1930, 280–291. doi: 10.1039/JR9300000280
- Anjaneyulu, A.S.R.; Mallavadhani, U.V.; Venkateswarlu, Y.; RamaPrasad, A.V. Ind. J. Chem. B. 1987, 26, 823–826.
- Cope, A.C. J. Am. Chem. Soc. 1935, 57 (3), 572–574. doi: 10.1021/ja01306a056
- Thapliyal, P.C.; Aggarwal, L.K. Ind. J. Chem. B. 2000, 39 (9), 706–708.