ABSTRACT
Copper nanoparticles (CuNPs) were synthesized by reducing a copper sulfate solution using the aqueous extract of Ferula macrocolea flowers. The optimum conditions were 0.01 M CuSo4.5H2O volume ratio 1.5, pH 12, temperature 80°C and incubation time 2 h. The ultraviolet–visible absorption spectra confirmed the formation of CuNPs with a characteristic peak at 576 nm. The X-ray diffraction and scanning electron microscope analysis of synthesized CuNPs revealed spherical-shaped nanoparticles with an average size of 22.3 nm ranged between 11 and 33 nm. Energy-dispersive X-ray and X-ray fluorescence spectra showed the peak in the copper region. The fourier transform infrared spectroscopy showed the capping of the CuNPs by compounds contained in F. macrocolea flowers extract. The synthesized CuNPs demonstrated activity against Staphylococcus aureus, followed by Bacillus cereus, Escherichia coli and Klebsiella pneumonia. Cytotoxicity assay indicated no decrease in viability of normal human lymphocytes up to a concentration of 25 µg/mL CuNPs.
GRAPHICAL ABSTRACT
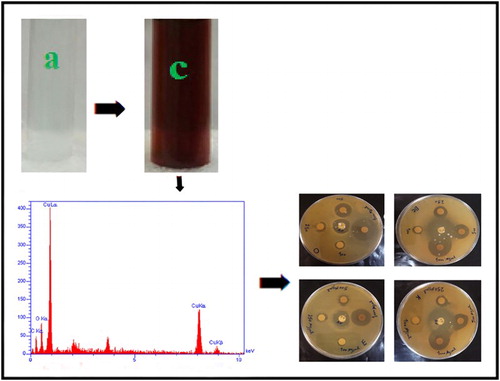
1. Introduction
Nanoparticles (NPs) are often referred to as particles sized between 1 and 100 nanometers that have unique physicochemical properties due to their small size and high surface area to volume ratio. This increased surface area of the smaller NPs enhances their reactivity with the surrounding molecules (Citation1). Metal NPs have been considered in recent years due to a wide variety of potential applications in catalysis (Citation2), plasmonics (Citation3), optoelectronics (Citation4), biology and biomedical fields (Citation5–7). NPs are synthesized by different physical and chemical methods that are expensive, time-consuming and involve the use of harmful and toxic chemicals. Thus, there is an increased need to develop rapid, economical and eco-friendly methods for the synthesis of NPs.
In recent years, the green synthesis of NPs using microorganisms (Citation8) and plants (Citation9) has been achieved. Green synthesis procedures are simple, inexpensive, rapid, nontoxic and a suitable method for large scale production (Citation10). Copper nanoparticles (CuNPs), due to their unique physical and chemical properties and low cost of preparation, have been of great interest (Citation11). They have wide applications as heat transfer systems, antibacterial materials, super strong materials, sensors and catalysts (Citation12–14). Various plant extracts such as Magnolia kobus, Ocimum sanctum, Gum karaya and Syzygium aromaticum have been used for the synthesis of CuNPs (Citation15–18). The genus Ferula comprises perennial herbs distributed from the mediterranean region to central Asia. Thirty species of this genus exist in Iran of which 15 are endemic (Citation19). The plants of this genus have been reported to have many pharmacological activities such as antimicrobial, antifungal, anti-inflammatory and antioxidant activities (Citation20–23). F. macrocolea is an endemic species of Iran that has been traditionally used as Anti-nausea and anti-stomach acid (Citation24). The present study evaluates the antioxidant properties of the F. macrocolea flowers extract, the green synthesis and characterization of CuNPs, and screening of their antibacterial activity against different strains of bacteria and cytotoxicity on normal human lymphocytes.
2. Experimental procedure
2.1. Collection of plant materials
The flowers of the wild F. macrocolea were collected from their local region (Kohdasht, Lorestan, Iran). They were thoroughly washed using normal water, followed by distilled water and then dried in the shade at room temperature.
2.2. Preparation of plant extract
The F. macrocolea flowers were cut into small pieces and crushed with help of mortar and pestle. Ten grams of powder sample were mixed into 100 mL of deionized water and the mixture was heated for 30 min in water bath at 80°C. The extract was then cooled and centrifuged at 12000 rpm for 20 min and filtered. The filtrate was stored at 4°C to use further.
2.3. Antioxidant activity
Antioxidant activity of extract was estimated on the basis of the stable 1,1-diphenyl-2-picrylhydrazyl (DPPH) free radical scavenging effect (Citation25). The radical scavenging activity (RSA) calculated using the following equation:(1) where AC is the absorbance of DPPH without sample and AS is the absorbance of DPPH in the presence of sample. The concentration of sample required to scavenge 50% of DPPH radicals was measured as IC50.
2.4. Synthesis of CuNPs
Various concentrations of copper(II) sulfate pentahydrate solution and the extract in different pH, temperature and incubation time were mixed. The reaction mixture was allowed to stand in a dark room to complete the reaction. The obtained precipitation was purified by repeated centrifugation at 12000 rpm for 20 min, dried in oven at 80oC for 8 h and stored in properly containers.
2.5. Characterization of NPs
The reduction of copper ions in copper(II) sulfate pentahydrate solution to CuNPs was periodically monitored by ultraviolet–visible (UV-Vis) Spectrophotometer. UV-Vis spectral analysis was done by JENWAY 6405 spectrometer at the range of 300–800 nm. The FT-IR spectroscopy were carried out for both the F. macrocolea flowers aqueous extract and the synthesized CuNPs to identify possible biomolecules in the F. macrocolea flowers extract that can participate in reduction process of copper ions and capping of the resulting CuNPs. The samples grinded with potassium bromide (KBr) and analyzed by shimadzu fourier transform infrared (FTIR) 8400S spectrophotometer at range of 4000–400 cm−1 with a resolution of 4 cm−1 . The sample morphology and size were examined with Tescan Mira 3 LMU scanning electron microscope (SEM) coupled with energy-dispersive X-ray (EDX) spectroscopy at a potential of 15 kV with 1 nm resolution. The composition of materials was identified by X-ray fluorescence (XRF) spectrometer (GNR, TX2000). An X-ray diffractometer (philips x’pert pro mpd) with Cu Kα radiation (1.5409 A°) was used to study of size and crystalline structure of the particles.
2.6. Antibacterial assay
Antibacterial activity of synthesized CuNPs was evaluated against Staphylococcus aureus PTCC 1112 (ATCC 6538), Bacillus cereus PTCC 1015 (ATCC 11778), Escherichia coli PTCC 1330 (ATCC 8739) and Klebsiella pneumonia PTCC 1053 (ATCC 10031) by disc diffusion method (Citation26). Nutrient agar plates were seeded with overnight bacterial culture. 30 μL of different concentrations (100−1000 μg/mL) of biosynthesized CuNPs were placed on the surface of the inoculated plates. After incubation at 37°C for 24 h, zone diameters were measured (mm). The minimum inhibitory concentration (MIC) values were also determined using broth microdilution method (Citation27).
2.7. Cytotoxicity assay
Normal lymphocytes isolated from healthy blood donors were seeded in triplicate wells of the 96-well flat bottom plate at a density of 2 × 104 cells/well. After spreading (4 h), the cells were treated with CuNPs colloidal suspension and incubated at 37°C in a humidified atmosphere of CO2 incubator. After 24 h incubation, cell cultures were evaluated by Methylthiazolyldiphenyl-tetrazolium bromide (MTT) assay (Citation28). The percent viability of cells exposed to treatments was calculated using the following equation:(2)
Concentration that inhibits 50% of cell growth was used as a parameter for cytotoxicity (Citation29).
3. Results and discussion
3.1. Synthesis of CuNPs
The results indicated that F. macrocolea flowers are a rich source of antioxidant compounds (DPPH IC50 146.3 ± 0.2 μg/mL). Therefore, their aqueous extract was used to reduce copper ions present in copper(II) sulfate pentahydrate solution and synthesis of CuNPs. The parameters such as concentration of extract and copper(II) sulfate pentahydrate solution, temperature, pH and incubation time affected the process of CuNPs synthesis. The maximum surface plasmon resonance (SPR) peak intensity was detected at 0.01 M copper(II) sulfate pentahydrate volume ratio equal to 1.5, pH 12, temperature 80°C and incubation time 2 h. By adding the F. macrocolea extract to copper(II) sulfate pentahydrate solution, the mixture color began to change from light blue to light green and finally dark brown (), which may correspond to the surface plasmon resonance of CuNPs.
3.2. UV–Vis spectra analysis
Synthesis and stability of CuNPs were confirmed using UV-Vis spectra analysis. The absorption spectrum of the synthesized NPs exhibited a peak at about 576 nm typical for CuNPs ((a)). The peak was observed even after one week indicating the stability of CuNPs. In addition, the colloidal suspension of CuNPs in liquid ammonia showed an absorption peak at 630 nm, which is specific for CuNPs ((b)).
3.3. FT-IR spectra analysis
The FT-IR analysis was used to determine the possible biomolecules responsible for the reduction of copper(II) sulfate pentahydrate solution and capping of the CuNPs. In (a), F. macrocolea extract shows the absorption peaks at 3417, 2927,1625, 1407 and 1078 cm−1 corresponding to O–H alcohol and phenol or N–H stretching of amine, C–H stretching of aromatic compound, C = O stretching in carbonyl group and −C−O stretching of ester, respectively. The intense signal 1078 cm−1 can be attributed to stretching vibrations of C–O-C bonds (Citation30–32). Most of the absorption peaks of the F. macrocolea flowers extract also exist in the FT-IR spectrum of CuNPs, either at identical positions or with minor changes in the position as well as the intensity of absorption, for instance the peaks at 3446, 2927, 1645, 1450 and 1080 cm−1 ((b)). The results indicated the organic compounds contained in F. macrocolea flowers extract such as phenolic and terpenoid compounds which can be identified by peak of hydroxyl group at 3417 cm−1 not only act as a reducing agent, but also act as capping ligands on the surface of the Phytosynthesized CuNPs.
3.4. SEM analysis
The SEM was performed to observe the morphological shape and size of synthesized CuNPs. The SEM analysis of CuNPs revealed the spherical-shaped particles ranged between 11 and 33 nm, which is in agreement with X-ray diffraction (XRD) . The SEM image of biosynthesized CuNPs was shown in .
3.5. EDX analysis
The presence of copper was confirmed by EDX analysis (). The synthesized NPs displayed an optical absorption peak about 1 keV, which is typical for the absorption of CuNPs. Carbon and oxygen signals are the major contaminants in produced CuNPs. Peak for C are from the grid used and oxygen is due to physical absorption of oxygen from environment during sample preparation.
3.6. XRF analysis
Results of XRF analysis indicated the existence of copper in the sample. The impurity elements were calcium (23.4%) and gallium (10%). Gallium was used as internal standard ().
3.7. X-Ray diffraction
The XRD pattern of CuNPs synthesized by F. macrocolea flowers extract has been shown in . Four main diffraction peaks for CuNPs were observed at 2-theta value of 36.72°, 43.54°, 50.60° and 74.24°, which correspond to 111, 111, 200 and 220 crystallographic planes of copper (Citation33–35). The average crystalline size of the CuNPs was estimated from the Debye–Scherrer’s equation (D = 0.9λ/β cos θ), where λ is wavelength of X-rays (1.5409 A°), β is the full width at half maximum of the diffraction peak (in radians) and θ is the Bragg angle, respectively (Citation36). According to Debye-Scherrer equation, the average size of the products was found to be 23.3 nm which is in agreement with the particle size measured from SEM.
3.8. Antibacterial activity
The antibacterial activity of synthesized CuNPs was evaluated against E. coli, S. aureus, K. pneumonia and B. sereus bacteria. The CuNPs showed activity against all tested organisms. It was found that the zone of inhibition increased with increasing the concentration of CuNPs ().
Figure 8. Antibacterial effect of the CuNPs against bacteria, (a) S. aureus, (b) B. cereus, (c) E. coli and (d) K. pneumonia.
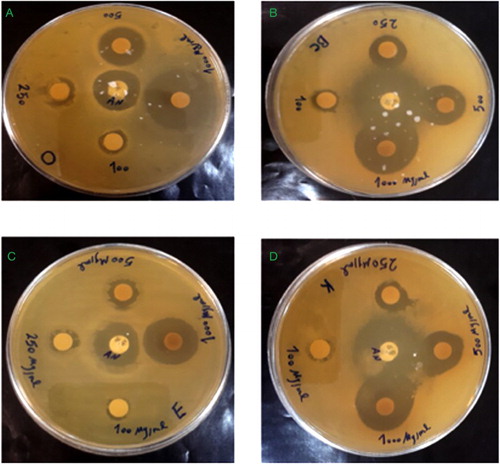
The maximum of inhibition zone was 23 mm in S. aureus bacteria followed by B. cereus, K. pneumonia and E. coli. The results obtained by microdilution tests were in agreement with the disc diffusion tests, where the antibacterial activity of synthesized CuNPs was higher against the gram-positive bacteria than the gram negatives. MIC value of CuNPs against S. aureus was 31.25 µg/mL ().
Table 1. Antibacterial activity of synthesized CuNPs.
The exact mechanism behind the biocidal activity of CuNPs is not yet fully known. It was suggested that copper ions originating from the CuNPs may interact with phosphorus and sulfur-containing biomolecules such as DNA and protein to distort their structures and thus disrupt biochemical processes (Citation37–39). Chatterjee et al. (Citation40) reported that the cell killing is caused by the NP-mediated superoxide radicals production in cells, which results in cellular lipid peroxidation, protein oxidation, and DNA degradation. Copper ions originating from the oxidation of the CuNPs are the prime effector for these effects.
3.9. Cytotoxic assay
In order to compare the effect of the CuNPs synthesized using F. macrocolea flowers extract on the bacteria with their toxicity toward mammalian cells, the cell viability of normal human peripheral blood cells treated with synthesized CuNPs was evaluated using the MTT assay at concentrations about their MIC value obtained in the antibacterial test. After 24 h of treatment, the CuNPs exhibited 83–88% cell viability at 50 and 100 μg/mL, respectively. It was observed that almost 100% of normal human peripheral blood cells survived up to 25 μg/mL CuNPs ().
Table 2. Percent viability of cells exposed to the CuNPs.
4. Conclusions
This study presents a simple, fast, cheap and eco-friendly method for CuNPs synthesis. The method was based on the reduction of copper(II) sulfate pentahydrate salt by F. macrocolea flowers aqueous extract. The synthesis of CuNPs was confirmed by visual observation, UV-Vis, SEM, XRD, EDX and XRF analysis. FTIR analysis showed that biomolecules present in the extract acted as capping and stabilizing agents in the formation of CuNPs. CuNPs synthesis was dependent on temperature, pH, time and concentration of the reactants. Antibacterial tests demonstrated the synthesized CuNPs were active against both gram-positive and gram-negative bacteria, but gram-positive bacteria were more sensitive to CuNPs as compared to gram-negative bacteria. Cytotoxicity assay indicated no decrease in cell viability of human lymphocytes treated with phytochemical-coated CuNPs up to a concentration of 25 µg/mL. These findings showed that green method could be used as a good alternative to the current physical and chemical methods associated with environmental toxicity.
Supplement_Material.doc
Download MS Word (502 KB)Acknowledgments
The authors are grateful to G. Veiskarami for help in plant identification.
Disclosure statement
The authors declare that there are no conflicts of interest regarding this manuscript.
Notes on contributors
Sima Shiravand received the Bachelor's degree in biology from Payame Noor University, Khorramabad, Iran, in 2001. She received the M.Sc. degree in biochemistry from Lorestan University, Khorramabad, Iran, in 2005. Since then, he has been with Biology Research Laboratory, Department of Biology, Lorestan University, Khorramabad, Iran. His main areas of research interest are Antibiotics; green synthesis; medicinal plants.
Farideh Azarbani received the M.Sc. degree in biochemistry from Faculty of Medical Sciences, Tarbiat Modares University, Tehran, Iran, in 2000. She received the Ph.D. degree in biochemistry from Tehran University, Tehran, Iran, in 2007. Since then, he has been with Department of Chemistry (2007–2010) and Department of Biology (from 2010 to now), Lorestan University, Khorramabad, Iran, where she is currently an Assistant Professor and head. His main areas of research interest are medicinal chemistry; Enzymology; Antibiotics; green synthesis; medicinal plants.
Additional information
Funding
References
- Sampath, M.; Vijayan, R.; Tamilarasu, E.; Tamilselvan, A.; Sengottuvelan, B. J. Nanotechnol. 2014, 33, 1–7. doi: 10.1155/2014/626523
- Al-Marhaby, F.A.; Seoudi, R. WJNSE. 2016, 6, 29–37. doi: 10.4236/wjnse.2016.61003
- Huergo, M.A.; Maier, C.M.; Castez, M.F.; Vericat, C.; Nedev, S.; Salvarezza, R.C.; Urban, A.S.; Feldmann, J. ACS Nano. 2016, 10, 3614–3621. doi: 10.1021/acsnano.5b08095
- Ismail, R.A.; Almashhadani, N.J.; Sadik, R.H. Appl. Nanosci. 2017, 7, 109–116. doi: 10.1007/s13204-017-0550-6
- Rehana, D.; Mahendiran, D.; Senthil Kumar, R.; Kalilur Rahiman, A. Biomed. Pharmacother. 2017, 89, 1067–1077. doi: 10.1016/j.biopha.2017.02.101
- Rehana, D.; Mahendiran, D.; Senthil Kumar, R.; Kalilur Rahiman, A. Bioprocess. Biosyst Eng. 2017, 40, 943–957. doi: 10.1007/s00449-017-1758-2
- Khatoon, N.; Mazumder, J.A.; Sardar, M. J. Nanosci. Curr. Res. 2017, 2, 1000107–1000115.
- Honary, S.; Barabadi, H.; Gharaeifathabad, E.; Naghibi, F. Dig. J. Nanomater. Bios. 2012, 7, 999–1005.
- Gunalan, S.; Sivaraj, R.; Venckatesh, R. Spectrochim. Acta. A. Mol. Biomol. Spectrosc. 2012, 97, 1140–1144. doi: 10.1016/j.saa.2012.07.096
- Kumar, V.; Yadav, SK. J. Chem. Technol. Biotechnol. 2009, 84, 151–157. doi: 10.1002/jctb.2023
- Varshney, R.; Bhadauria, S.; Gaur, MS. Nano. Biomed. Eng. 2012, 4, 99–106. doi: 10.5101/nbe.v4i2.p99-106
- Gupta, R.; Sanotra, S.; Sheikh, H.N.; Kalsotra, B.L. JNSC. 2013, 3, 1–9.
- Samadi, S.; Khalilian, F.; Tabatabaee, A. JNSC. 2014, 4, 1–8.
- Ghane, M.; Sadeghi, B.; Jafari, A.R.; Paknejhad, A.R. Int. J. Nano. Dimens. 2010, 1, 33–40.
- Lee, H.J.; Song, J.Y.; Kim, B.S. J. Chem. Technol. Biotechnol. 2013, 88, 1971–1977.
- Kulkarni, B.V.; Kulkarni, P. Int. J. Chem. 2013, 1, 1–4.
- Padil, V.V.T.; Cernik, M. Int. J. Nanomedicine. 2013, 8, 889–898.
- Subhankari, I.; Nayak, P.L. World. J. Nano. Sci. Technol. 2013, 2, 14–17.
- Mozaffarian, V. A Dictionary of Iranian Plant Names, Farhang Moaser Publishers: Tehran, 1996; pp 228–229.
- Khodayari, H.; Amani, S.H.; Amiri, H. J. Med. Plants. Ecophytochemistry. 2013, 8, 12–26.
- Maggi, F.; Cecchini, C.; Cresci, A.; Coman, M.M.; Tirillini, B.; Sagratini, G.; Papa, F. Fitoterapia. 2009, 80, 68–72. doi: 10.1016/j.fitote.2008.10.001
- Iranshahi, M.; Fata, A.; Emami, B.; Shahri, B.M.J.; Fazly Bazzaz, B.S. Nat. Prod. Commun. 2008, 3, 1543–1546.
- Kartal, N.; Sokmen, M.; Tepe, B.; Daferera, D.; Polissiou, M.; Sokmen, A. Food. Chem. 2007, 100, 584–589. doi: 10.1016/j.foodchem.2005.09.084
- Mandegary, A.; Sayyah, M.; Reza Heidari, M. DARU. 2004, 12, 58–62.
- Huang, D.; Ou, B.; Prior, R.L. J. Agric. Food. Chem. 2005, 53, 1841–1856. doi: 10.1021/jf030723c
- Li, Z.; Lee, D.; Sheng, X.; Cohen, R.E.; Rubner, M.F. Langmuir. 2006, 22, 9820–9823. doi: 10.1021/la0622166
- Engelkirk, P.G.; Duben-Engelkirk, J.L. Laboratory Diagnosis of Infectious Diseases: Essentials of Diagnostic Microbiology, LWW: Baltimore, 2008; pp 168–169.
- Mosmann, T. J. Immunol. Methods. 1983, 65, 55–63. doi: 10.1016/0022-1759(83)90303-4
- Cole, SP. Cancer. Chemother. Pharmacol. 1986, 17, 259–263. doi: 10.1007/BF00256695
- Rajathi1, K.; Sridhar, S. Int. J. Chem. Tech. Res. 2013, 5, 1707–1713.
- Rastogi, L.; Arunachalam, J. Mater. Chem. Phys. 2011, 129, 558–563. doi: 10.1016/j.matchemphys.2011.04.068
- Shankar, S.; Ahmad, A.; Sastry, M. Biotechnol. Prog. 2003, 19, 1627–1631. doi: 10.1021/bp034070w
- Mott, D.; Galkowski, J.; Wang, L.; Luo, J.; Zhong, C. J. Langmuir. 2007, 23, 5740–5745. doi: 10.1021/la0635092
- Sastry, A.; Aamanchi, R.B.K.; Prasad, C.S.R.L.; Murty, B.S. Environ. Chem. lett. 2013, 11, 183–187. doi: 10.1007/s10311-012-0395-x
- Usman, M.S.; Ibrahim, N.A.; Shameli, K.; Zainuddin, N.; Yunus, W.M. Molecules. 2012, 17, 14928–14936. doi: 10.3390/molecules171214928
- Montes-Burgos, D.; Hole, W.P.; Smith, J., Lynch, I., Dawson, K. J. Nanopart. Res. 2010, 12, 47–53. doi: 10.1007/s11051-009-9774-z
- Raffi, M.; Mehrwan, S.; Bhatti, T.; Akhter, J.; Hameed, A.; Yawar, W.; ul Hasan, M. Ann. Microbiol. 2010, 60, 75–80. doi: 10.1007/s13213-010-0015-6
- Wu, X.H.; Ye, L.; Liu, K.; Wang, W.; Wei, J.; Chen F.P.; Liu C.S. Biomed. Mater. 2009, 4, 045008. doi: 10.1088/1748-6041/4/4/045008
- Ruparelia, J.P.; Chatterjee, A.K.; Duttagupta, S.P.; Mukherji, S. Acta. Biomater. 2008, 4, 707–716. doi: 10.1016/j.actbio.2007.11.006
- Chatterjee, A.K.; Chakraborty, R.; Basu, T. nanotech. 2014, 25, 11360–11370. doi: 10.1088/0957-4484/25/13/135101