ABSTRACT
The methanolic extract of Peumus boldus (P. boldus) has been studied as a potential green corrosion inhibitor for carbon steel in 0.5 M H2SO4 using gravimetric, potentiodynamic polarization and electrochemical impedance spectroscopy tests at 25, 40 and 60°C, and concentrations of 0, 0.1, 0.3, 0.5, 0.7 and 1.0 g/L. Results indicate that P. boldus is physically adsorbed onto the steel surface and acts as a mixed corrosion inhibitor of carbon steel corrosion. The data fitted best to the Temkin adsorption isotherm. The inhibition efficiency of P. boldus extracts was observed to increase with increasing its concentration but decreases on increasing the test temperature. The inhibitive effect is attributed to the presence of vitamin E, Limonene and eucalyptol in the extract.
GRAPHICAL ABSTRACT
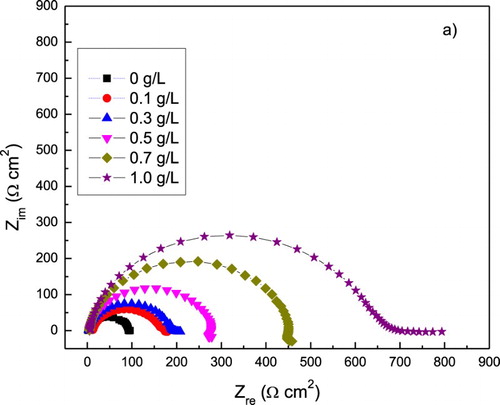
1. Introduction
Carbon steel is one of the most used metals in industry for many purposes. However, it is in contact with many corrosive chemicals such as hydrochloric and sulfuric acids in processes such as chemical cleaning, which could lead to the dissolution of the metal. In order to protect the metal surface from these aggressive environments, several techniques such as coatings, cathodic protection, anodic protection and corrosion inhibitors are available. In many practical applications, the use of corrosion inhibitors is the most widely used method for corrosion mitigation (Citation1). Various synthetic organic and inorganic chemicals have been studied as corrosion inhibitors for mild steel in different aqueous media (Citation2–9). Since many synthetic inhibitors are toxic, environmental concerns favor the search for natural non-toxic compounds that manifest a strong affinity towards metal surfaces (Citation10–20). Affinity of organic compounds for metal surfaces and subsequent adsorption is reportedly (Citation21–24) favored by the presence of polar functional groups containing S, O and N atoms. The π electrons in these heteroatoms are believed to be responsible for their adsorption onto the metal surface and inhibitive effects. The corrosion inhibitors obtained from plants are highly preferred because they are readily available, inexpensive and environmentally benign. There are many studies that report the use of leaves, bark and fruits of different plants as green corrosion inhibitors for mild steel in various aggressive media. Extracts from Pisidium guajava (Citation17), Punica granatum (Citation18), Ginko biloba (Citation19), Tinospo racrispa (Citation20), Ficus carica (Citation21), Uncaria gambir (Citation22), Phyllanthus amarus (Citation23), Murraya koenigii (Citation24), Justicia gendarussa (Citation25), Azadiracta indica (Citation26), Hibiscus sabdariffa and Zenthoxy lumalatum (Citation27) have been studied as green corrosion inhibitors for mild steel in various acidic media because of the antioxidants contained inside them.
Peumus boldus (Boldo) is a perennial tree originated from the central regions of Chile. Its leaves are widely used in the treatment of digestive and hepatobiliary disorders, and they are included in a number of pharmacopoeias. Additionally, it has been shown that its leaf extracts possess great antioxidant properties (Citation28). Its activity is mainly due to the presence of flavonoids and alkaloids, especially the aporphine alkaloid boldine, although other constituents like the glycoside boldoglucine, some phenolic compounds and the essential oil have been reported to exert some activity (Citation29, Citation30). Research carried out in the early 1990s concluded that boldine is one of the most potent natural antioxidants (Citation31). However, in a work carried out by Quezada et al. (Citation32), it was shown that the antioxidant activity in boldo leaf extracts is mainly due to the flavonoid fraction (44.1%) followed by the alkaloid fraction (15.5%), with cate chine and boldine being the main contributors of the antioxidant activity of these two fractions, 60.9% and 35.6% of the total activity, respectively. Thus, due to the important antioxidants present in P. boldus, the main goal of this work is to evaluate its use as a green corrosion inhibitor for carbon steel in sulfuric acid, an electrolyte frequently used in the industry.
2. Experimental procedure
2.1. Testing solution
Dried leaves of P. boldus were crushed and soaked into methanol during 72 h, after which, the solution was filtered and the solvent evaporated, obtaining a solid paste. This extract was not soluble in the electrolyte, so it was weighed, diluted in 2 ml of methanol and 98 ml of water and used as stock solution. As corrosive solution, 0.5 M H2SO4 was used under normally aerated conditions, prepared with analytical grade reagents, since it is normally used in chemical processes such as pickling and decaling. Concentrations of methanol extract of P. boldus were 0, 0.1, 0.3, 0.5, 0.7 and 1.0 g/L.
2.2. Testing material
As testing materials, 1018 carbon steel rods, containing 0.14% C, 0.90% Mn, 0.30% S, 0.030% P and as balance Fe, with a diameter of 6.00 mm was used. Its microstructure is shown in , which consists of ferrite as steel matrix in major proportion and perlite in lower proportion. For the electrochemical tests, specimens measuring 10 mm long were used, with a copper wire spot welded on it, encapsulated in a commercial, polymeric resin, ground with 1200 emery paper, washed with water and degreased with acetone and warm air.
2.3. Electrochemical techniques
In this work, potentiodynamic polarization curves (PC) and electrochemical impedance spectroscopy (EIS) measurements were used. For the PC, specimen was immersed into the solution during 20 min, after which the free corrosion potential value, Ecorr, reached a steady-state value. After this, the specimen was polarized from −1000 mV upto + 1000 mV, with respect to the Ecorr value, at a scan rate of 1 mV/s. Corrosion current density values, Icorr, were calculated by using Tafel extrapolation. A fully automated potentiostat/galvanostat from ACM instruments was used for this. A saturated calomel electrode and a graphite rod were used as reference and auxiliary electrodes, respectively. For the EIS tests, a signal with an amplitude of 20 mV was applied to the specimen, which was at the Ecorr value, in an frequency interval of 20 KHz–0.05 Hz. Testing temperatures were room temperature (25°C), 40°C and 60°C. All the experiments were repeated three times.
2.4. Weight loss experiments
For the weight loss measurements, specimens measuring 20 mm in length and a diameter 6.00 mm were used. They were ground to 1200 grade emery paper, washed and degreased with acetone. The weight loss value, ΔW, was calculated according to:(1) where m1 and m2 are the mass of the specimen before and after corrosion, respectively, and A, the exposed area of the specimen. Experiments lasted 72 h. For the weight loss tests, inhibitor efficiency, I.E., was calculated as follows:
(2) where ΔW1 and ΔW2 are the weight loss without and with inhibitor, respectively. Selected specimens were observed in a LEO 1450VP scanning electron microscope (SEM) for their analysis.
2.5. FTIR spectroscopic analysis of green corrosion inhibitor
The green corrosion inhibitor as well the corrosion products were examined under Fast Fourier Transformed Infrared (FTIR) analysis by using a Bruker equipment in KBr pellet in the 4500–570 cm−1 interval. The peak values of the FTIR were recorded. Each analysis was repeated twice to detect the characteristic peaks and their functional groups.
2.6. Gas chromatography/mass spectrometry analysis
The P. boldus extract was also analyzed on a GC Agilent 6890 System Plus coupled to Agilent 5973 Network Mass selective detector, to detect all majority organic natural compounds content in the methanol extract. The gas chromatography/mass spectrometry (GC–MS) equipped with silica capillary column (30 m × 0.25 mm, film thickness 0.25 mm). The GC conditions were temperature 45–250°C with a temperature gradient of 10°C/min. Injected a volume 1.0 μL of green corrosion inhibitor at 0.02 g/L of concentration. The identification of the majority of components was based on their retention times by comparison with standard retention times.
3. Results
3.1. Weight loss tests
The effect of P. boldus concentration on the weight loss, I.E. and the metal surface coverage, θ, for 1018 carbon steel in 0.5 M H2SO4 solution at 25°C, 40°C and 60°C are given in . This table shows that the weight loss decreases as the P. boldus concentration increases, obtaining the lowest weight loss with the addition of 1.0 g/L. As the temperature increases, the weight loss increases, obtaining the highest weight loss at 60°C.
Table 1. Effect of P. boldus concentration on the weight loss, inhibitor efficiency and Θ at 25°C, 40°C and 60°C.
The I.E. and metal surface coverage increase with the P. boldus concentration, reaching its highest value when 1.0 g/L of inhibitor is added. Thus, the decrease in the weight loss is due to the P. boldus adsorption on the surface metal (Citation33, Citation34), since as the metal surface covered by P. boldus increases, the I.E. increases also. I.E. and surface coverage area decreased as the temperature increased. So, if we assume that the P. boldus adsorption on the surface metal is the responsible for the decrease in the corrosion rate, then its adsorption must follow one of the different adsorption isotherms, such as Langmuir, Temkin or Frumkin. As shown in , according to their correlation coefficients, the best fitting was obtained with a Temkin type of adsorption isotherm. Temkin isotherm is given by:(3) where θ is the metal surface covered by the inhibitor, given by the I.E. divided by 100, f designates the molecular interaction parameter related to the molecular interaction in the adsorption layer as well as the heterogeneity of the surface, Cinh is the inhibitor concentration and Kads is the equilibrium constant of adsorption process. In this model, adsorption is characterized by a uniform distribution of binding energies, up to some maximum binding energy and it would decrease linearly rather than in a logarithmic way with θ.
Figure 2. (a) Tempkin and (b) Langmuir type of adsorption isotherms for 1018 carbon steel in 0.5 M H2SO4 in presence of P. boldus extract.
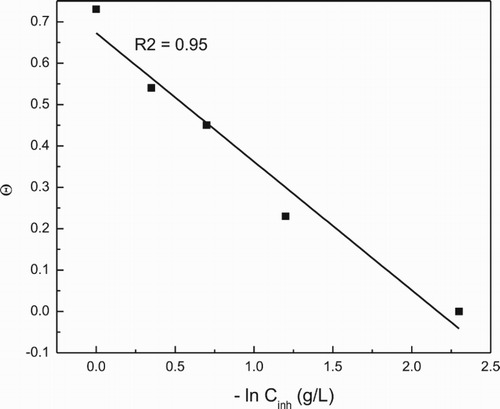
By using data from , and after fitting, the equilibrium constant Kads was determined and, by using following equation:(4) Gibbs energy, ΔGad, was calculated, obtaining a value of −21.7 kJ mol−1. Generally, values of the adsorption free-energy around −20 kJ mol−1 have typically been correlated with the electrostatic interactions between organic molecules and charged metal surface (physisorption) while those values in the order of −40 kJ mol−1 are associated with charge sharing or transfer from the organic molecules to the metal surface (chemisorption) to form a co-ordinate type of bond (Citation35). The negative value of the free-energy of adsorption value means that the adsorption process is spontaneous, while the value around −20 kJ mol−1 indicates that P. boldus was physically adsorbed on the steel surface. Similar results have been reported for the adsorption of Pongamia pinnata and Lasianthera africana onto mild steel immersed in sulfuric and hydrochloric acid respectively (Citation18, Citation36), for the adsorption of Osmanthus fragran onto carbon steel in hydrochloric acid (Citation37), for the adsorption of a Bis-Schiff bases of isatin onto mild steel in hydrochloric acid (Citation38) and for Pactin terrestrial onto aluminum in hydrochloric acid (Citation39).
The apparent activation energy, Ea, associated with 1018 carbon steel in uninhibited and inhibited acid solution was determined by using an Arrhenius-type plot according to the following equation:(5) where ΔW is weight loss value, R is the molar gas constant, T is the absolute temperature and F is the frequency factor. Arrhenius plots of (log ΔW) against 1/T for 1018 carbon steel in 0.5 M H2SO4 in the absence and presence of P. boldus is shown in . The apparent activation energy obtained for the corrosion process in the free acid solution was found to be 15.46 and 23.1 kJ mol−1 in presence of the inhibitor respectively. Notably, the energy barrier of the corrosion reaction increased in the presence of the inhibitor, hence the corrosion rate decreases, most probably due to the physisorption of the inhibitor on the steel surface. This increase in the activation energy for the dissolution of carbon steel in inhibited solution has been interpreted as a physical adsorption (Citation18, Citation40).
3.2. Potentiodynamic PC
The effect of P. boldus concentration on the PC for 1018 carbon steel in 0.5 M H2SO4 at 25°C is given in , where it can be seen that the plots describe an active behavior. The Ecorr value reamains almost constant regardless of the inhibitor concentration, however, the Icorr value decreases as the P. boldus concentraion increases, reaching its lowest value with the addition of 1.0 g/L of P. boldus. Both, the cathodic and anodic Tafel slopes, were affected by the presence of P. boldus; however, it had a greater influence on the anodic slope, which was decreased with the addition of P. boldus, indicating that it acts as a mixed type of inhibitor. I.E. was calculated by the following equation:(6) where Icorr and I′corr are the corrosion current density values without and with the addition of inhibitor, respectively. shows that I.E. values increase with an increase in the P. boldus concentration, in agreement with the weight loss results.
Figure 4. Effect of P. boldus concentration in the polarization curves for 1018 carbon steel in 0.5 M H2SO4 at 25°C.
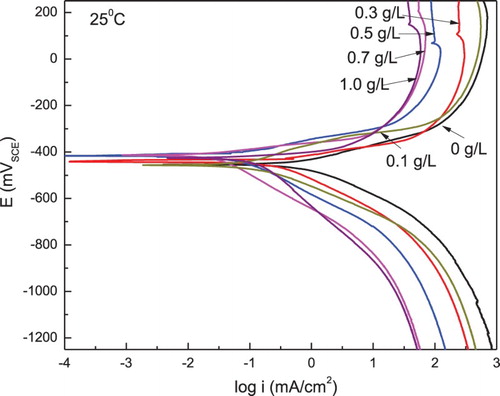
Table 2. Electrochemical parameters obtained from PC for 1018 carbon steel in 0.5 M H2SO4 at different concentrations of P. boldus at 25°C.
The effect of temeprature in the PC for 1018 carbon steeel in 0.5 M H2SO4 containing the most efficient inhibitor cocentration of P. boldus, that is, 1.0 g/L, is shown in , where it can be seen that although the Ecorr value is unnaffected, both the anodic and cathodic current density values increased at least one order of magnitude when the temperature increases from 25°C to 60°C. The Icorr value also increases for almost two orders of magnitude with the temperature. Thus, a complete loss in the corrosion protection given by the P. boldus extract with an increase in the temperature, maybe due to the extract degradation.
3.3. EIS measuremnts
EIS data in both Nyquist and Bode formats for 1018 carbon steel in 0.5 M H2SO4 with different concentrations of P. boldus is given in . Nyquist digrams, (a), show a single, depressed, capacitive-like semicircle at all the frequency values, with its center below the real axis, indicating a charge transfer controlled corrosion process. The semicircle diameter increases with the P. boldus concentration, which is due to the adsorption of the inhibitor and the formation of a protective film. At low frequency values, some elongations can be observed, which can be due to the accumulation of species at the metal/solution interface (Citation41, Citation42) and increase the total impedance value. On the other hand, Bode plots, (b), show a single peak around 200 Hz, indicating the presence of only one time constant. As the P. boldus concentration increases, the phase angle remain constant over a wide frequency range, indicating the presence of two phase constants, and it reaches a phase angle close to −80°. The semicircle diameter corresponds to the polarization resistance, Rp, which is the sum of the solution resistance, Rs, charge transfer resistance, Rct, and the resistance of all the accumulated corrosion products, Rf, at the metal/solution interface. The Rp or Rct values are inversely proportional to the corrosion rate.
Figure 6. Effect of P. boldus concentration in the (a) Nyquist and (b) Bode curves for 1018 carbon steel in 0.5 M H2SO4 at 25°C. Symbols are experimental data, lines are the fitted results.
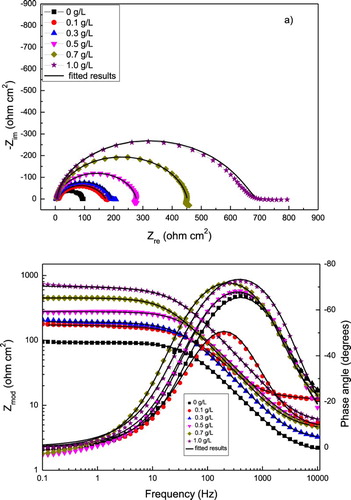
Thus, EIS data can be represented by two electric equivalent circuits as those shown in . The depression of the semicircles shown in the Nyquist plot with their centers below the real axis is a phenomenon known as “dispersion effect” and it is attributed to the surface roughness and other heterogeneities, (Citation43, Citation44). It can be noticed from that in the uninhibited solution only one constant phase element (CPE) appears, whereas for bronze in the presence of P. boldus, two CPEs appear instead of an ideal capacitor due to the dispersion effect. The impedance of the CPE, ZCPE, is given by (Citation45):(7) where Y0 is the admittance, i = √−1, ω, the angular frequency and n, a physical parameter which gives interphase properties of the working electrode such as roughness, inhibitor adsorption, etc. In , Rs is the solution resistance, Rct, the double-layer resistance where the charge transfer takes place. CPEdl is a CPE related to capacitance of the double electrochemical layer. This CPEdl is placed instead of an ideal capacitor, Cdl, to take into account the dispersion effects due to surface roughness and other surface heterogeneities. Rf corresponds to the resistance of the film formed by the inhibitor and corrosion products, whereas that CPEf is the CPE related to the capacitance of the film formed by the inhibitor and the corrosion products. Electrochemical parameters obtained from the fitting of EIS data by using electric circuits shown in are given in . It can be seen that Rct increase, whereas CPEdl decreases with an increase in the P. boldus concentration, reducing the corrosion rate, probably due to the adsorption of the inhibitor. On the other hand, Rf increases also with the inhibitor concentration, decreasing the film-formed inhibitor conductivity, which shows that the protectiveness of the film increases in presence of the inhibitor. As the P. boldus concentration increases and the corrosion rate is decreased, ndl value increases, due to a decrease in the metal surface roughness due to a decrease in the corrosion rate. I.E. values were calculated by using following equation:
(8) where Rinh and Rblank are the charge transfer resistance values with and without inhibitor, respectively. Efficiency values obtained with PC and EIS measurements, and , and used to obtain the adsorption isotherms. As it can be seen from , once again, the best fit was obtained with the Temkin adsorption isotherm, and, by fitting the data, the equilibrium constant Kads and the ΔGad was calculated, obtaining a value of −18.4 and −16.7 kJ mol−1, which indicates a physical type of adsorption.
Figure 8. Temkin type of adsorption isotherm for 1018 carbon steel in 0.5 M H2SO4 in presence of P. boldus extract by using data from the PC and EIS measurements.
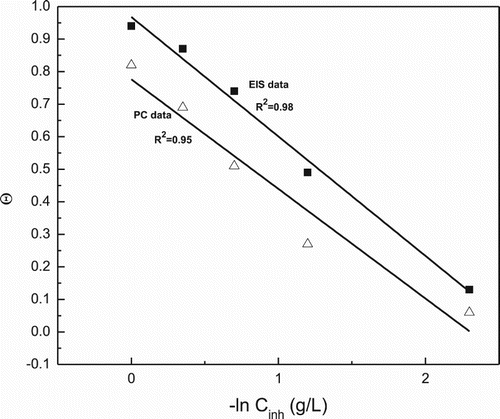
Table 3. Electrochemical parameters used to fit EIS data shown in .
An alternative way to calculate the double-layer capacitance Cdl, is by using following equation:(9) where fmax is the frequency value where the maximum value of imaginary impedance is found. Results given in indicate an increase in the charge transfer resistance with the P. boldus concentration and thus, a decrease in the corrosion rate. On the other hand, the double-layer capacitance value, Cdl, which is related to CPEdl, decreases as the P. boldus concentration increases, reaching its lowest value at a concentration of 1.0 g/L. An increase in Rct refers to more impediment of the active area at the metal surface as a result of the increase in inhibitor concentration (Citation41, Citation42). In addition, the values of the double-layer capacitance (Cdl) decrease by adding inhibitor in to corrosive solution. Additionally, the double-layer capacitance value can be calculated with equation:
(10) where ε is the double-layer dielectric constant, ε0, the vacuum electrical permittivity, δ, the double-layer thickness, and A, the surface area. Thus, the decrease in the Cdl value is attributed to the replacement of the adsorbed water molecules at the surface metal by the inhibitor having lower dielectric constant (Citation43). Also, the decrease in surface area which acts as a site for charging may be considered as another reason for the Cdl decrease (Citation44). These points suggest that the role of inhibitor molecules is preceded by its adsorption at the metal–solution interface.
Figure 9. Effect of P. boldus concentration in charge transfer resistance, Rct and double-layer capacitance, Cdl, values for 1018 carbon steel in 0.5 M H2SO4 at 25°C.
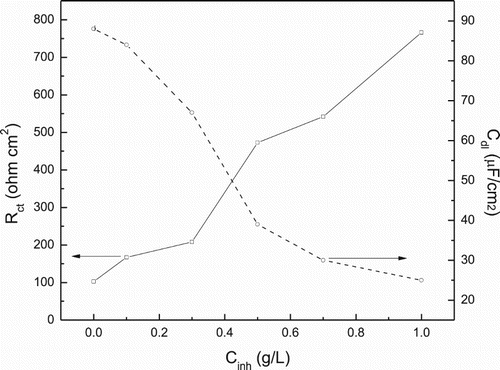
The effect of testing temperature in the Nyquist diagrams for 1018 carbon steel in 0.5 M H2SO4 solution containing 0 and 1.0 g/L of P. boldus is shown in . This figure shows that data display a single depressed, capacitive-like semicircle at all frequency values regardless of the testing temperature, indicating that the corrosion mechanism is not affected by the temperature. However, either in the absence or presence of P. boldus, a marked decrease in the semicircle diameter is observed when the temperature increases from 25°C to 60°C, indicating an increase in the corrosion rate, as observed in the PC shown in , due to the lack of protection given by the P. boldus extract. The semicircle diameters were higher in presence of P. boldus than in its absence. shows the effect of testing temperature on the Rct values in both the absence and presence of P. boldus as well as in the I.E. values. It is clear the detrimental effect of temperature, since inhibitor efficiency decreases with increasing the testing temperature.
Figure 10. Effect of temperature in the Nyquist diagrams for 1018 carbon steel in 0.5 M H2SO4 containing (a) 0 and (b) 1.0 g/L of P. boldus.
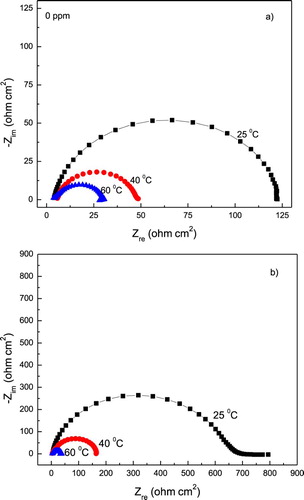
Table 4. Variation of Rct values for 1018 carbon steel in 0.5 M H2SO4 with 0 and 1.0 g/L of P. boldus together with the inhibitor efficiency as a function of testing temperature.
3.4. Sem images
Some SEM micrographs of the specimens corroded during 72 h to the corrosive solution containing 0 or 1.0 g/L of P. boldus at 25°C, 40°C and 60°C are given in . For specimen immersed in the uninhibited solution, ((a,c,e)), the formed corrosion products film is full of porous and cracks which increase in number as the temperature increases from. The corrosive solution can ingress through these porous or cracks and corrodes the underlying metal. On the other hand, for steel immersed in the solution containing 1.0 g/L, ((b,d,f)), the formed film is more compact with much less amount of porous or cracks, but the number of these defects increased with the temperature, explaining why the corrosion rate increased with the temperature even in the presence of P. boldus extract. This formed film is the result of the reaction between the released Fe2+ ions during the dissolution of the steel, and the inhibitor, forming a film. During the adsorption of an organic inhibitor on a metal surface, in this case, physical adsorption, as shown in Section 3.1 above, it normally involves the replacement of water molecules absorbed on the metal surface by the inhibitor as follows:(11) The inhibitor may then combine with freshly generated Fe2+
ions on steel surface, forming metal-inhibitor complexes (Citation45):
(12)
(13)
Figure 11. SEM micrographs of 1018 carbon steel immersed in 0.5 M H2SO4 containing 0 g/L (a, c and e) and 1.0 g/L (b, d and f) of P. boldus at 25°C (a and b), 40°C (c and d) and 60°C (e and f).
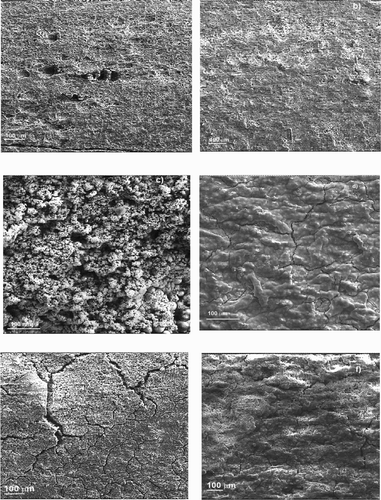
These complexes form the corrosion products film, which can be either protective or not protective. In this case, the formed complexes between Fe2+ ions and P. boldus extract reduces the corrosion rate, at least at 25°C, according to micrographs shown in and the weight loss results.
3.5. GC–MS analysis of green inhibitor
The main compounds found are given organized in . From the chromatogram, only nine compounds could be separated, and only seven compounds were identified by comparing the mass spectrometry fragmentation with the library software. The identified compound, with chemical structure as given in , were monoterpenes such as p-cymene (1), limonene (2), eucalyptol (3), tabanone (4) and α-tocopherol (vitamin E, 6), one flavonoid, which is chrysin (5), and one alkaloid, namely indolo[2,1-1]isoquinoline, 5,6,12,12a-tetrahydro-2,3,9,10-tetramethoxy (7). Limonene and eucalyptol have been previously identified in the chemical content of green corrosion inhibitors (Citation24, Citation25, Citation46). Vitamin E (α-tocopherol) has been reported as corrosion inhibitor also for Cu and a Cu-40Zn alloys in acid rain, having an efficiency value of 90% (Citation26). The rest of the compounds has not been previously reported as corrosion inhibitors. The IR characterization is widely used technique for functional groups (Citation47–53), whereas nuclear magnetic resonance is a technique for pure compounds to identify the type of hydrogens or carbons. The green inhibitor extracted from P. boldus is a mixture of natural compounds, that is way to know what are the principal and interesting functional groups as –OH, –C9O, etc., which could act as a green corrosion inhibitor. Similarly, Verma et al. (Citation45) used Spirogyra algae as a green corrosion inhibitor for mild steel in hydrochloric acid. They attributed the inhibition effect of S. algae to the presence of hydroxyl, amino and carboxyl groups which are responsible for their adsorption on the mild steel surface. Similarly, Ngobiry (Citation48) used Brassica oleracea extract for the corrosion inhibition of a C-Mn steel in 0.5 M H2SO4 obtaining efficiency values higher than 95% which was attributed to the presence of hydroxyl, amino and carboxyl groups in the extract. As can be seen, the GC–MS analysis provided excellent information about the chemical content and relative abundance in the mixture.
Figure 12. Chemical structure of the main components found in the methanol P. boldus extract showing (a) p-cymene, (b) limonene, (c) eucalyptol, (d) tabanone, (e) α-tocopherol (vitamin E), (f) chrysin and (g) indolo[1-1, 2]isoquinoline, 5,6,12,12a-tetrahydro-2,3,9,10-tetramethoxy (7).
![Figure 12. Chemical structure of the main components found in the methanol P. boldus extract showing (a) p-cymene, (b) limonene, (c) eucalyptol, (d) tabanone, (e) α-tocopherol (vitamin E), (f) chrysin and (g) indolo[1-1, 2]isoquinoline, 5,6,12,12a-tetrahydro-2,3,9,10-tetramethoxy (7).](/cms/asset/5d57e2e4-34de-4e53-96ab-12c06cbd6c5c/tgcl_a_1369167_f0012_b.gif)
Table 5. Identified compounds according to the chromatogram of P. boldus extract.
In most inhibition studies, the formation of donor–acceptor surface complexes between π-electrons of inhibitor and the vacant d-orbital of metal were postulated (Citation46, Citation49). The presence of heteroatoms atoms such N and O directly attached to the aromatic ring enhances the availability of π-electrons to get bonded to the vacant d-orbital of Fe. The high performance of P. boldus could also be due to large size of this molecule which covers wide areas on the metal surface and thus retarding the corrosion (Citation50, Citation51). However, a synergistic effect of this molecule with the other constituents may play important role on the overall effect of P. boldus extract. shows a summary of the main results obtained with the use of different green inhibitors for steel in different acid environments (Citation17, Citation47, Citation48, Citation52, Citation53). It can be seen that there is not a unique way that these inhibitors are adsorbed on to the steel metal, either chemical or physical adsorption. Also, in most of the cases, these naturally occurring inhibitors affect both the cathodic and anodic reactions, acting as mixed type of inhibitors regardless of the used acid. In all cases, including in this work, high efficiency values, higher than 90%, were obtained, which is very encouraging.
Table 6. Comparison of results obtained with different green inhibitors for steel in different acid environments.
4. Conclusions
A study of the methanolic extract of P. boldus for 1018 carbon steel in 0.5 M H2SO4 has shown that it acts as a good, anodic type of inhibitor, with its efficiency increasing with its concentration, but decreasing with the testing temperature. Additionally, its efficiency increases with increase in the time only during a few hours, after which, it is desorbed, decreasing its efficiency. Its inhibitory properties are due to the presence of organic compounds, mainly flavonoids and alkaloids, such as vitamin E, Limonene and eucalyptol, among others, which have heteroatoms, which make the P. boldus to be physically adsorbed on the steel surface, following a Temkin type of adsorption isotherm, forming protective corrosion products.
Disclosure statement
No potential conflict of interest was reported by the authors.
Notes on contributors
M. Y. Díaz-Cardenas is a PhD student at CIICAp, Universidad Autonoma del Estado de Morelos. She is Chemical Engineer and has an M.Sc. in Engineering and Applied Sciences.
Dra. M. G. Valladares-Cisneros is a Lecturer at the Facultad de Ciencias Quimcias e Ingenieria, Universidad Autonoma del Estado de Morelos. She works in Fitochemistry of Natural Products.
Dra. S. Lagunas-Rivera is a Lecturer at the Department of Chemistry at the Instituto Tecnologico de Tuxtla Gutierrez, Chiapas, Mexico. She works in Fitochemistry Natural Products.
Dr. V. M. Salinas-Bravo works at the Department of Materials, at the Instituto Nacional de Electricidad y Energias Renovables. He is a specialist in Corrosion and protection of metals.
Dr. R. Lopez-Sesenes is a lecturer at the Facultad de Ciencias Quimicas e Ingenieria, Universidad Autonoma del Estado de Morelos. He is specialist in Corrosion and Protection of Metals.
Dr. J. G. Gonzalez-Rodriguez has a PhD in corrosion Science and Engineering. He works in Corrosion Inhibitors.
References
- Finsgar, M.; Jackson, J. Corros. Sci. 2014, 86 (1), 17–41. doi: 10.1016/j.corsci.2014.04.044
- Ellayyachy, M.; Elidrissi, A.; Hammouti, B. Corros. Sci. 2006, 48 (10), 2470–2479. doi: 10.1016/j.corsci.2005.09.016
- Tebbji, K.; Faska, N.; Tounsi, A.; Oudda, H.; Benkaddour, M.; Hammouti, B. Mater. Chem. Phys. 2007, 106, 260–267. doi: 10.1016/j.matchemphys.2007.05.046
- Lashgari, M.; Malek, A.M. Electrochim. Acta, 2010, 55 (12), 5253–5257. doi: 10.1016/j.electacta.2010.04.054
- Barouni, K.; Bazzi, L.; Salghi, R.; Mihit, M.; Hammouti, B.; Albourine, A.; El Elissami, S. Mater. Lett. 2008, 62 (8), 3325–3327. doi: 10.1016/j.matlet.2008.02.068
- Bentiss, F.; Traisnel, M.; Lagrenee, M. Corros. Sci. 2000, 42 (1), 127–146. doi: 10.1016/S0010-938X(99)00049-9
- Ouchrif, A.; Zegmout, M.; Hammouti, B.; Dafali, A.; Benkaddour, M.; Ramdani, A.; Elkadiri, S. Prog. Org. Coat. 2005, 53 (2), 292–296. doi: 10.1016/j.porgcoat.2005.02.010
- Osman, M.M.; Shalaby, M.N. Mater. Chem. Phys. 2003, 77 (3), 261–269. doi: 10.1016/S0254-0584(01)00580-6
- Martinez, D.; Gonzalez, R.; Montemayor, K.; Juarez-Hernandez, A.; Fajardo, G.; Hernandez-Rodriguez, M.A.L. Wear, 2009, 267, 255–258. doi: 10.1016/j.wear.2008.12.056
- Zhang, B.; He, C.; Wang, C.; Sun, P.; Li, F.; Lin, Y. Corros. Sci. 2015, 94 (1), 6–20. doi: 10.1016/j.corsci.2014.11.035
- Ji, G.; Anjum, S.; Sundaram, S.; Prakash, R. Corros. Sci. 2015, 90 (1), 107–117. doi: 10.1016/j.corsci.2014.10.002
- Ngobiri, N.C.; Oguzie, E.E.; Li, Y.; Liu, L.; Oforka, N.C.; Akaranta, O. Int. J. Corrosion, 2015, 2015, 1–9. doi: 10.1155/2015/404139
- El Hamdani, N.; Fdil, R.; Tourabi, M.; Jama, C.; Bentiss, F. Appl. Surf. Sci. 2015, 357, 1294–1305. doi: 10.1016/j.apsusc.2015.09.159
- Rajeswari, V.; Kesavan, D.; Gopiraman, M.; Viswanathamurthi, P.; Poonkuzhali, K.; Palvannan, T. Appl. Surf. Sci. 2014, 314, 537–545. doi: 10.1016/j.apsusc.2014.07.017
- Raja, P.B.; Qureshi, A.K.; Rahim, A.A.; Osman, H.; Awang, K. Corros. Sci. 2013, 69 (2), 292–301. doi: 10.1016/j.corsci.2012.11.042
- Deng, S.; Li, X. Corros. Sci. 2012, 64 (1), 253–262. doi: 10.1016/j.corsci.2012.07.017
- Anupama, K.K.; Ramya, K.; Shainy, K.M.; Joseph, A. Mater. Chem. Phys. 2015, 167, 28–41. doi: 10.1016/j.matchemphys.2015.09.013
- Singh, A.; Ahamad, I.; Singh, V.K.; Quraishi, A. J. Solid State Electrochem. 2011, 15 (10), 1087–1097. doi: 10.1007/s10008-010-1172-z
- Fouda, A.S.; Shalabi, K.; Idress, A.A. Green Chem. Lett. Rev. 2015, 8 (1), 17–29. doi: 10.1080/17518253.2015.1073797
- Abdel Rahman, H.H.; Seleim, S.M.; Hafez, A.M.; Helmy, A.A. Green Chem. Lett. Rev. 2015, 8 (2), 88–94. doi: 10.1080/17518253.2015.1111430
- Behpour, M.; Ghoreishi, S.M.; Khayatkashani, M.; Soltani, N. Mater. Chem. Phys. 2012, 131, 621–633. doi: 10.1016/j.matchemphys.2011.10.027
- Deng, S.; Li, X. Corros. Sci. 2012, 55 (2), 407–415. doi: 10.1016/j.corsci.2011.11.005
- Ibrahim, T.H.; Zour, M.A. Int. J. Electrochem. Sci. 2011, 6 (6), 6442–6456.
- Hussin, M.H.; Kassim, M.J. Mater. Chem. Phys. 2011, 125, 461–468. doi: 10.1016/j.matchemphys.2010.10.032
- Quraishi, M.A.; Singh, A.; Singh, V.K.; Yadav, D.K.; Singh, A.K. Mater. Chem. Phys. 2010, 122, 114–122. doi: 10.1016/j.matchemphys.2010.02.066
- Fuchs-Godec, R.; Zerjav, G. Corros. Sci. 2015, 97 (1), 7–16. doi: 10.1016/j.corsci.2015.03.016
- Okafor, P.C.; Ikpi, M.E.; Uwah, I.E.; Ebenso, E.E.; Ekpe, U.J.; Umoren, S.A. Corros. Sci. 2008, 50 (11), 2310–2317. doi: 10.1016/j.corsci.2008.05.009
- Rodriguez de Almeida, E.; Mário-Melo, A.; Xavier, H. Phytother. Res. 2000, 14 (2) 99–102. doi: 10.1002/(SICI)1099-1573(200003)14:2<99::AID-PTR600>3.0.CO;2-4
- Simirgiotis, M.J.; Schmeda-Hirschmann, G. J. Chromatogr. 2010, 1217, 443–449. doi: 10.1016/j.chroma.2009.11.014
- O’Brien, P., Carrasco-Pozo, C., Speisky, H. Chem. Biol. Interact. 2006, 159, 1–17. doi: 10.1016/j.cbi.2005.09.002
- Speisky, H.; Cassels, B.K. Pharmacol. Res. 1994, 29 (1), 1–12. doi: 10.1016/1043-6618(94)80093-6
- Quezada, N.; Asencio, M.; del Valle, J.M.; Aguilera, J.M.; Gomez, B. J. Food Sci. 2004, 69 (2), 371C–376C.
- Rahmouni, K.; Keddam, M.; Srhiri, A.; Takenouti, H. Corros. Sci. 2005, 47 (10), 3249–3266. doi: 10.1016/j.corsci.2005.06.017
- Bousselmi, L.; Fiaud, C.; Tribollet, B.; Triki, E. Electrochim. Acta, 1999, 44 (12), 4357–4363. doi: 10.1016/S0013-4686(99)00151-6
- Popova, A.; Sokolova, E.; Raicheva, S.; Christov, M. Corros. Sci. 2003, 45 (1), 33–58. doi: 10.1016/S0010-938X(02)00072-0
- Eddy, N.O.; Odoemelam, S.A.; Odiongenyi, A.O. J. Appl. Electrochem. 2009, 39 (7), 849–857. doi: 10.1007/s10800-008-9731-z
- Bethencourt, M.; Botana, F.J.; Calvino, J.J.; Marcos, M.; RodrÍguez-Chacón, M.A. Corros. Sci. 1998, 40 (10), 1803–1819. doi: 10.1016/S0010-938X(98)00077-8
- Li, X.; Deng, S.; Fu, H. Corros. Sci. 2012, 62 (2), 163–175. doi: 10.1016/j.corsci.2012.05.008
- Oguzie, E.E. Mat. Chem. Phy. 2006, 99, 441–446. doi: 10.1016/j.matchemphys.2005.11.018
- Larabi, L.; Benali, O.; Harek, Y. Mater. Lett. 2007, 61 (11), 3287–3291. doi: 10.1016/j.matlet.2006.11.054
- Özcan, M.; Dehri, I.; Erbil, M. Appl. Surf. Sci. 2004, 236, 155–164. doi: 10.1016/j.apsusc.2004.04.017
- Solmaz, R.; Kardas, G.; Culha, M.; Yazıcı, B.; Erbil, M. Electrochim. Acta, 2008, 53 (12), 5941–5952. doi: 10.1016/j.electacta.2008.03.055
- Zhang, D.Q.; Gao, L.X.; Zhou, G.D. J. Appl. Electrochem. 2003, 33 (2), 361–366. doi: 10.1023/A:1024403314993
- Raistrick, I.D. Ann. Rev. Mater. Sci. 1986, 16, 343–370 doi: 10.1146/annurev.ms.16.080186.002015
- Poorqasemi, E.; Abootalebi, O.; Peikari, M.; Haqdar, F. Corros. Sci. 2009, 51 (9), 1043–1054. doi: 10.1016/j.corsci.2009.03.001
- Okafor, P.C.; Ebenso, E.E.; Ekpe, U.J. Int. J. Electrochem. Sci. 2010, 5 (2), 978–992.
- Verma, D.K.; Khan, F. Green Chem. Lett. Rev. 2016, 9 (1), 52–60. doi: 10.1080/17518253.2015.1137976
- Ngobiri, N.C.; Oguzie, E.E.; Li, Y.; Liu, L. Int. J. Corr. 2015, 2015, 9.
- Poornima, T.; Nayak, J.; Shetty, A.N. J. App. Electrochem. 2011, 41 (2), 223–233. doi: 10.1007/s10800-010-0227-2
- Bontiss, F.; Lagrence, M.; Traisne, M. Corrosion, 2000, 56 (6), 733–742. doi: 10.5006/1.3280577
- Akalezi, C.O.; Oguzie, E. E. Int. J. Ind. Chem. 2015, 6 (2), 273–284. doi: 10.1007/s40090-015-0050-z
- Singh, A.K.; Mohapatra, S.; Pani, B. J. Ind. Eng. Chem. 2016, 33, 288–297. doi: 10.1016/j.jiec.2015.10.014
- Victoria, S.N.; Prasad, R.; Manivannan, R. Int. J. Electrochem. Sci. 2015, 10 (5), 2220–2238.