ABSTRACT
N,N-Dimethylformamide (DMF)-based ionic liquid is a multipurpose ionic liquid having numerous applications. In the present study it has been shown to be an efficient and recyclable nucleophilic reagent-cum-solvent for the synthesis of a variety of 1,2-disubstituted-3,4-dihydronaphthalenes by cycloaddition of different vinylarenes with electron-deficient alkynes. The present protocol has been demonstrated to be more efficient, green and cost effective as compared with other reported methods.
GRAPHICAL ABSTRACT
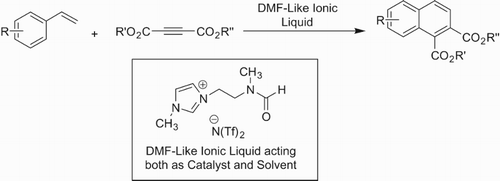
Introduction
There are numerous functionalized cyclic compounds which can be obtained by organo-catalyzed cycloaddition of suitable compounds in one-step. The organo-catalyzed reactions become more effective when performed by the ionic liquid version of organo-catalysts. Such reagents are obtained by adding the organic reagent to an ionic liquid part and are called task-specific ionic liquids. The reported data reveal that such attempts have been quite successful in terms of catalytic efficiency, low cost, green procedure and easy isolation of the product (Citation1).
Jia-Li Jiang et al. have found that in the presence of N,N-dimethylformamide dimethyl acetal (DMF-DMA), vinylarenes undergoes a smooth cycloaddition reaction with electron-deficient alkynes to give the adducts of 1,2-disubstituted 3,4-dihydronaphthalenes () (Citation2). It was claimed to be the first example of an efficient synthesis of functionalized dihydronaphthalenes from the one-step catalytic cycloaddition of easily available vinylarenes and activated alkynes.
Scheme 1. DMF-DMA catalyzed the cycloaddition of vinylarenes with electron-deficient alkynes to give the adducts of 1,2-disubstituted 3,4-dihydronaphthalenes.

It is well known that some functionalized dihydronaphthalenes not only exhibit physiological and biological activities, but they are also valuable intermediates for the synthesis of numerous biologically active natural products and pharmaceuticals. Therefore, the design and development of more efficient synthetic methods for the preparation of 1,2-disubstituted 3,4-dihydronaphthalenes have always been an attractive field of research and has kept stirring the interest of synthetic chemists.
Jia-Li Jiang et al. highlighted the catalytic role of DMF-DMA organo-reagent in the cycloaddition of vinylarenes with electron-deficient alkynes. However, they could not explain the dependence of cycloaddition of styrene and electron-deficient alkynes on DMF-DMA. In my opinion the nitrogen in the DMF-DMA reagent is the key factor that may attack either the vinylic beta carbon of styrene or acetylenic carbon of acetylene dicarboxylate leading to the initiation of polar cycloaddition reaction. The nucleophilicity of the nitrogen in DMF can be enhanced by preventing its delocalization to carbonyl oxygen by acetal formation as shown in .
DMF is defined as a polar aprotic as well as a nucleophilic solvent. Due to this nucleophilic feature of DMF, most of the synthetically useful nucleophilic substitution reactions are carried out in DMF. It facilitates such reactions due to two reasons. First, being a polar solvent it stabilizes the polar intermediates or transition states, second it facilitates the removal of the leaving group by the nucleophilic character of its formamide group. In addition to this, DMF has been reported to have a significant influence on the course of certain reactions. In a review article, it has been shown to be more than just a solvent (Citation3). The reactions that have been reported to be highly influenced by dimethyl formamide include direct oxidation of organic halides to carbonyls by NaIO4-DMF (Citation4), the conversion of alkyl halides into alcohols (Citation5) synthesis of pyrido[2,3-d]pyrimidine derivatives (Citation6), chlorination of 2-hydroxyethylamides (Citation7), preparation of l,l-dioxo-7-substituted cepherns (Citation8), dialkylation of diethyl 3,4-dihydroxy-2,5-thiophene dicarboxylate (Citation9), and O-alkylation of ethylene glycols and carboxylic (Citation10). There are certain organic reagents that work efficiently when used in DMF, i.e. the 1,3,5-trichlorotriazine-dimethylformamide (TCT-DMF) complex is a powerful multipurpose reagent (e.g. 2,4,6-trichloro[l,3,5]-triazine and DMF) (Citation11).
Keeping in view the significant role of DMF in affecting the outcome of certain reactions as well as reagents, it was envisioned to synthesize its ionic liquid version to make it more efficient. The DMF has been found to affect the outcome of these reactions due to the nucleophilic character of its nitrogen atom as evident from their proposed reaction mechanisms. In order to make the ionic liquid version of DMF we tentatively conceived its structure as shown below and synthesized it. It demonstrated all the desired physical properties. It was named as DMF-based task-specific ionic liquid ().
In order to check the efficacy of our DMF-based task-specific ionic liquid, we performed a couple of DMF-dependent reaction with it, i.e. direct conversion of alkyl halide to the corresponding alkyl azide which is essentially a nucleophilic substitution reaction and direct oxidation of various organic halides into the corresponding carbonyl compounds (Citation12). The outcome of these reactions with the DMF-based ionic liquid turned out to be much better than reported routine methods.
Oxidation of alkyl halide with sodium periodate is DMF dependent. The same idea seems to be applicable in the case of DMF-DMA-catalyzed cycloaddition of styrene and activated alkynes to give functionalized dihydronaphthalenes. The possible pathways of the reaction can be visualized as given later in the text. There may be two possibilities of the path of reaction and both yielding the same product. According to the first possibility of the proposed mechanism the oxygen of DMF-based ionic liquid (3a) may attack the beta carbon of vinylic carbon of styrene (3b) to form the zwitter ionic intermediate (3c). The anionic carbon then attacks the acetylenic carbon of acetylene dicarboxylate (3d) producing another zwitter ion intermediate (3e) which then undergoes the indicated bonding arrangements to furnish the product (3f) and regenerated ionic liquid (3a). The former undergoes final aromatization to give the final product (3h) ().
Scheme 3. First possible path way of DMF-like ionic liquid-catalyzed cycloaddition of styrene with electron deficient alkynes.
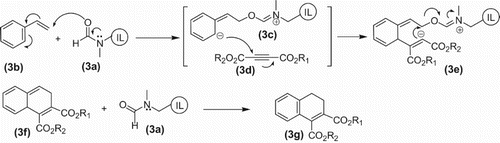
Another possibility is that the oxygen of DMF-based ionic liquid (4a) attacks the acetylenic carbon of acetylene dicarboxylate (4b) leading to a highly charged reactive intermediate (4c). The anionic carbon of the intermediate (4c) attacks the beta carbon of styrene (4d) with the formation of another intermediate (4e) which undergoes indicated bond shuffling to yield (4f) with regeneration of DMF-based ionic liquid (4g). The restoration of aromaticity gives the desired product (4h) ().
Scheme 4. First possible path way of DMF-like ionic liquid-catalyzed cycloaddition of styrene with electron deficient alkynes.
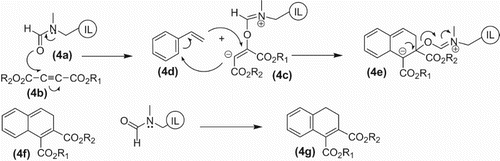
The choice of mechanism apparently seems to depend on the sequence of the addition of reagents.
Results and discussions
After the selection of the structure, a retrosynthesis was carried out to find the facile synthetic scheme for DMF-based ionic liquid. As many synthetic routes can be foreseen, the choice of the reaction scheme was dictated by the availability of chemicals in our laboratory and thus the finalized synthetic scheme was arrived at as shown in .
The N-methylation of aminoethanol (5a) was achieved by reductive amination with paraformaldehyde to give N-methylaminoethanol (5b) through the protocol reported by Sukanta et al. (Citation13). N-methylaminoalcohol was converted to the corresponding chloro derivative (5c) by triphenyl phosphine and tricholoroisocynuric acid (Citation14). At this stage N-formaylation was prefered over conversion of chloride into iodide becuase in this case formamide would also facilitate halide substitution. The N-formylation was achived by heating the secondary amine with ammonium formate to get qauantitative yields of N-choloroethyl-N-methyl formamide (5d) as reported by Reddy et al. (Citation15). The substitution of the chloro group was achieved through the ordinary method of heating (5d) with sodium iodide in dry acetone to get iodo derivative (5e). The N-alkylation of imidazole to form (5f) was achieved by using the methodology developed by Dupont et al. (Citation16). The 1HNMR confirmed the preparation of the desired cation part of the ionic liquid. The required DMF-like ionic liquid (5g) was achieved by anionic metathesis of the iodide salt by using silver bis(trifluoromethanesulfonyl)amide, i.e. silver triflimide, i.e. salt (AgNTf2) through the procedure used by Xun Hea (Citation17). The absence of the iodide ion in mass spectra confirmed anion metathesis. Thus, the successful synthesis of the desired DMF-like task-specific ionic liquid (5g) was achieved.
For the present study, the same DMF-based ionic liquid was prepared and characterized according to the reported procedure (Citation11). Then it was screened for its possible catalytic action to achieve cycloaddition of styrene and dimethylacetylenedicarboxylate. For this we investigated the optimum conditions. The DMF-based ionic liquid will act both as a solvent as well as a reagent.
To determine the optimization of reaction conditions we used styrene and dimethylacetylenedicarboxylate as a model reaction. To 3 ml of DMF-based ionic liquid, styrene (1.0 mmol) was added slowly and drop wise under constant magnetic stirring at 10°C with the aim of preparing the (3c) intermediate. However, the GC-MS analysis did not indicate the desired change under this condition. The resulting mixture appeared to be viscous; we added 1 ml of dichloromethane to reduce the viscosity and to promote the solubility of the reaction contents. Then an equimolar amount of dimethylacetylenedicarboxylate (3b) (1.0 mmol) was added to the reaction mixture with constant stirring and the reaction was allowed to stir for further 10 h. The GCMS analysis indicated 10% of the product. Then the reaction mixture was warmed to a higher temperature, i.e. 25°C and the conversion to product was enhanced to 45%. In order to examine the effect of temperature on the outcome of the reaction, we performed the reaction at various temperatures by repeating the same reaction at different temperatures. The data obtained are shown in . The results indicate the gradual increase in the rate of reaction with an increase in the temperature. The results seem to be best at between 30°C and 35°C. A further increase in temperature may lead to undesired products formed by the nucleophilic attack on ester carbon instead of acetylenic carbon and degradation of ionic liquid.
Table 1. Effect of temperature on rate of reaction.
The model reaction mixture was stirred at 35°C and progress of the reaction was monitored by GC-MS analyses. The completion of the reaction was detected after 32 h of stirring. After completion of the reaction, dichloromethane was removed by a rotary evaporator. The product was extracted from the ionic liquid by simple extraction with 2 × 3 ml diethyl ether in pure form. The ether solvent was removed by rotary evaporation in vacuo. The product was subjected to flash column chromatography (silica gel 60 A, 230–400 mesh) using eluent: hexane/ethyl acetate = 4:1 yielding the desired adduct (92%) as a yellowish liquid. The ether insoluble ionic liquid was dried under vacuum for 15 h. The resulting adduct dimethyl 3,4-dihydro-1,2-naphthalate (3g) was then characterized and confirmed by spectroscopic techniques.
Then we examined the impact of various derivatives of alkynes on their cycloaddition with styrene at 35°C. Dimethylacetylenedicarboxylate furnished the maximum yield (). Whereas other diethyl and ethyl methyl versions also produced best results; however, the phenyl and diphenyl derivatives were found to be quite poor to react with styrene to give the desired corresponding adduct. As for as regioselectivity of the cycloaddition reaction is concerned, the methyl ester site seems to be more reactive for aromatic carbanion of (3c).
Table 2. Reaction of styrene with various acetylene derivatives to yield adducts at 35°C.
In order to scrutinize the scope of the optimized reaction conditions, the cycloaddition reactions of various substituted vinylarenes with dimethylacetylenedicarboxylate were examined and the results are given in . Most of the vinylarenes exhibit a smooth cycloaddition reaction to yield the corresponding adducts in best amounts efficiently under soft and green conditions. In contrast to this, the reported procedure for some transformations obviously involves the use toxic material, high temperature and laborious workup. The mechanism governing this reaction is clearly polar which involves nucleophilic and electrophilic attacks stabilized by the highly polar nature of DMF-like ionic liquid. The type of substituents seems to affect the yield of the product, i.e. the electron-withdrawing groups (EWG) at ortho and para positions yield better products (, entries 11–13) due to stabilization of the negative charge in the intermediate (3c). The improved yields of the products in the ionic liquid solvent can be also ascribed to enhanced stabilization of highly polar-reactive intermediates. The electron-donating groups (EDG) on vinylarenes lead to slightly reduced percentage yields of the products (, entries 1–8). The mechanism (Scheme 3) seems to be more plausible and consistent with the observed data. Greater the power of electron-withdrawing effect, the better the yield (, entries 6–8). A wide variety of vinylarenes were investigated to check the scope of the procedure. Isopropylenearenes were found to be as efficient as simple vinylarenes (, entries 10–11). Vinylcyclopentadiene gave corresponding adducts in a yield comparable to vinyl benzene, whereas the vinyl naphthalene was relatively poor in response. Diene type molecules (, entries 15–16) were quite sluggish under these conditions, probably due to lack of stabilization of (3c) type intermediate. In addition to styrene other heterocyclic vinylarenes such as vinyl pyridine and vinyl furan were also tested in the present protocol. The vinyl pyridine and 2-vinyl furan provide satisfactory yields; however, 3-vinylfuran provided comparatively a lesser amount of the corresponding adducts. The reactivity profile of various vinyl heterocyclic compounds can be rationalized with the polar mechanism shown in Scheme 3. In the case of 3-vinlypyrdine at 35°C, the side product was obtained due to the nucleophilic attack of pyridine nitrogen on acetylenic carbon in (3d). However, at a lower temperature of 20°C no such side product was obtained. In addition to relatively better yields of adducts under the ionic liquid condition, other merits of this methodology include lower temperature, no use of hazardous chemicals and solvents leading to a green and cost-effective procedure. Easy isolation of the product in pure form by simple extraction with ether is another key feature of the current procedure, avoiding laborious workup which often causes the wastage of products. The recycling of the ionic liquid-based reagent is their important aspect. The DMF-like ionic liquid has always shown to be effective for re-use as a solvent as well as a reagent in various reported reactions. A similar kind of recycling pattern has also been shown here in this case as depicted in .
Table 3. DMF-based ionic liquid-catalyzed cyclic addition of vinylarenes with dimethylacetylene dicarboxylate.
Table 4. Number of recycles of DMF-like ionic liquid in cycloaddition of styrene and dimethyl acetylene dicarboxylate.
Jia-Li Jiang et al. employed DMF-DMA as a reagent for cycloaddition of vinylarenes with diethylacetylenedicarboxylate, but the reaction required 1.0 mmol of the catalyst and temperature as high as 110°C for 5 h in toluene. The comparison of tabulated data obtained under ordinary and ionic liquid conditions indicates improvements in the yields of adducts. The procedural convenience and green methodology are additional advantages of the new procedure over the reported one. The changes in solvent conditions (nonpolar toluene to highly polar ionic liquid) have a large impact on the mechanistic path of the reaction
Recycling of catalyst
Recycling of the ionic liquid-based reagents or solvents is one of their key feature. The recycling potential of DMF-like ionic liquid was studied with the cycloaddition reaction of vinyl benzene and dimethylacetylenedicarboxylate. The results obtained are given in . The ionic liquid shows remarkable recycling potential. The slight gradual loss in recovery of ionic liquid may partly be due to handling and structural degradation arising from the loss of the N-methyl group as evident from its 1HNMR.
Experimental
The required chemicals were obtained from Merck and Fluka. All reagents and solvents were purified according to standard methods. IR spectra were run on a Shimadzu FT-IR 8300 spectrophotometer. 1HNMR and 13CNMR spectra were recorded in DMSO-d6 and CDCl3 using a Bruker Avance DPX instrument (1H NMR 250 MHz, 13C NMR 62.9 MHz). Chemical shifts were reported in parts per million (δ) downfield from TMS. All of the coupling constants (J) are in hertz. The mass spectra were recorded on a Shimadzu GC-MS QP 1000 EX instrument. Elemental analyses were run on a Thermo Finnigan Flash EA-1112 series. Melting points were determined in open capillaries with a Buchi 510 melting point apparatus. Thin-layer chromatography was carried out on silica gel F254 analytical sheets obtained from Fluka. Column chromatography was performed on Merck Kiesel gel (230–270 mesh).
Synthesis scheme of DMF-like ionic liquid
N-methyl 2-aminoethanol(5b)
In a 200 ml round-bottom flask, a mixture of amino ethanol (5a) (30 g, 0.491 mol) zinc chloride (133.85 g, 0.982 mol) paraformaldehyde (29.49 g, 0.982 mol) in dichloromethane (150 ml) was stirred at room temperature for 1 h under dry atmosphere. Sodium borohydride (37.14 g, 0.982 mol) was then added and resulting mixture was stirred for 18 h. The progress of the reaction was monitored by TLC using ninhydrin reagent. The reaction mixture was then quenched by the addition of aqueous ammonia (200 ml, 2N), stirred for 10 min and the organic layer was separated. The aqueous part was extracted with dichloromethane (25 ml x1), the combined organic extracts were concentrated in vacuo after drying over anhydrous Na2CO3. The crude product was purified by flash chromatography over neutral alumina using hexane:diethyl ether (3:1) as the eluent to yield 51 g (72%) of pure N-methylaminoethanol (5b) as a colorless liquid product; b.p. 150–155°C.
1H-NMR (400 MHz, DCCl3): δ 2.35 (d, 3H, 3 J2,3 = 5.5 Hz, H-5), 2.60 (m, 2H, 3 J2,3 = 5.5 Hz, H-3), 3.54 (t, 2H, 3 J2,3 = 5.5 Hz, H-2), 3.64 (s-broad, 1H, H-1); 13CNMR (CDCl3), (300 MHz) δ (36.05, CH3), (54.30,CH2), (60.30, CH2); MSEI m/z (% rel. abound.): 75 (M+ 40), 74 (49), 73 (10), 44(100), 31 (15); HRMS (ESI): calcd for C3H9NO (M+) 75.110, found: 75.108.
N-(2-chloroethyl)-N-methyl amine(5c)
Ph3P (267.5 g, 1.02 mol) was dissolved in 200 ml of anhydrous acetonitrile in a 300-ml round-bottom flask. Then 83.7 g, 0.36 mol of tricholoroisocynuric acid was added slowly for about 30 min. The reaction mixture was stirred and heated at 60°C. Then N-methylaminoethanol (5b) (51 g, 0.68 mol) was added to the mixture, and the reaction was stirred for 3 h. On completion of the reaction, 10 ml of water was added to quench the reaction. Most of the acetonitrile was removed by a rotary evaporator and the residue was extracted with 100 ml (4 × 25 ml) diethylether. The organic layers were combined, concentrated and dried over Na2SO4. The product (5c) was further purified with flash chromatography to give 44.51 g (70%) 2-chloro, N-methyl ethylamine as a colorless liquid with b.p. 109–111°C.
1H-NMR (400 MHz, DCCl3): δ 2.54 (d, 3H, 4 J3,2 = 0.63 Hz, H-2), 2.72 (m, 2H, 3 J4,3 = 6 Hz, H-3), 2.96 (s-broad, 1H, H-1), 3.50 (t, 2H, 3 J4,3 = 6 Hz, H-4); 13CNMR (CDCl3), (300 MHz) δ (34.93. CH3) (42.34, CH2) (57.55, CH2). HRMS (ESI): calcd for C3H8ClN (M+) 93.555, found: 93.550.
N-(2-chloroethyl)-N-methyl formamide(5d)
To a solution of N-methyl-2-chloro ethyl amine (5c) (44.51 g, 0.48 mol) in dry acetonitrile (500 ml) was added anhydrous ammonium formate (45.36 g, 0.72 mol) and the resulting mixture was heated at 95°C (bath temperature) for 15 h. Acetonitrile was removed under reduced pressure. The residue was diluted with ethyl acetate (200 ml) and washed with distilled water (4 × 50 ml). The organic layer was concentrated and dried over anhydrous Na2SO4 and then further purified by flash chromatography to yield (51.84 g 90%) colorless liquid formamide (5d).
1H-NMR (400 MHz, DCCl3): δ 3.22 (s, 3H), 3.58 (t, 2H, 3 J6,5 = 6.68 Hz, H-6), 3.92 (t, 2H, 3 J6,5 = 6.68 Hz, H-5), 8.02 (s, 1H, H-2a); 13CNMR (CDCl3), (300 MHz) δ (31.99, CH3) (44.62 CH2) (59.58 CH2) (165.06 CHO). HRMS (ESI): calcd for C4H8ClNO (M+) 121.565, found: 121.564.
N-(2-iodoethyl)-N-methyl formamide(5e)
To a solution of N-(2-chloroethyl)-N-methyl formamide (5d) (51.84 g, 0.24 mol) in 25 ml of anhydrous acetone is added drop wise to a stirred solution of NaI (37.5 g, 0.25 mol) in 250 ml of anhydrous acetone over a period of 45 min at room temperature, stirring was continued for 20 h. The precipitate formed is collected by filtration and washed with 100 ml of acetone. The combined filtrate is evaporated and the residue is distilled to give a colorless oil. And it was further purified by flash chromatography to give 66.34 g, 73% yield.
1H-NMR (400 MHz, DCCl3): δ 3.24 (s, 3H, H-4), 3.60 (t, 2H, 3J6,5 = 7.77 Hz, H-6), 4.00 (t, 2H, 3J6,5 = 7.77 Hz, 4J5,4 = 0.63Hz, 4J5,2a = 0.63Hz H-5), 7.79 (s, 1H, H-2a); 13CNMR (CDCl3), (300 MHz) δ (−0.60, CH2) (32.59 CH3) (56.10 CH2) (164.39 CHO); HRMS (ESI): calcd for C4H8INO (M+) 213.017, found: 213.016.
1-[2-(Formylamino)ethyl]-3-methyl-1H-imidazol-3-ium iodide(5f)
Freshly distilled N-methyl imidazole (19.7 g, 0.24 mol) and (66.34 g, 0.31 mol) of N-(2-iodoethyl)-N-methyl formamide (5e) were added to 100 ml of acetonitrile (CH3CN) and brought to reflux with stirring under nitrogen at 80°C for 24 h. After completion of the reaction as evident from TLC analysis, the reaction mixture was cooled to room temperature and a solid compound was collected during the cooling process. The acetonitrile was removed by a rotary evaporator under vacuo. The resulting white solid was washed with ethyl acetate, dried under reduced pressure at 30°C for 6 h to afford imidazolium iodide (v) yield 46.56 g, 89%.
1H-NMR (400 MHz, CD3OD): δ 3.16 (s, 3H, H-11), 3.92 (t, 2H, 3 J6,7 = 6.63Hz, H-6), 4.19 (t, 2H, 3 J6,7 = 6.63Hz, H-7), 7.40–7.98 7.40(s, 1H, H-5) 7.70(s, 1H, H-4) 7.98(s, 1H, H-2), 7.75(s, 1H, H-9a); 13CNMR (CDCl3), (300 MHz) δ (32.26, CH3) (37.20) (46.97, CH2) (51.27, CH2) (116.41, CH) (123.30, CH) (140.28, CH) (161.49, CHO) HRMS (ESI): calcd for C8H14N3O (M+) 168.216, found: 168.219.
1-[2-(Formylamino) ethyl]-3-methyl-1H-imidazol-3-ium triflimide(5g)
To a solution of 1-[2-(formylamino)ethyl]-3-methyl-1H-imidazol-3-ium iodide (5f) (46.56 g, 0.16 mol) in dry acetonitrile (20 mL) was added silver triflimide (41.12 g, 0.16 mol). The mixture was stirred for 2 h in the dark under nitrogen. The mixture was filtered to remove the light yellow salt and the filtrate was evaporated by rotary evaporation under vacuum and dried in vacuo to generate the product (5g) as a clear liquid with a very light brown color with yield 67 g, 100% yield.
1H-NMR (400 MHz, CD3OD): δ 3.16 (s, 3H, H-12), 3.75 (s, 3H, H-11), 3.82 (t, 2H, 3 J6,7 = 6.63Hz, H-6), 4.08(t, 2H, 3 J6,7 = 6.63Hz, H-7), 7.75(s, 1H, H-9a) 8.40–9.47 (s, 1H, H-5) 8.65(s, 1H, H-4) 8.47(s, 1H, H-2); 13CNMR (CDCl3), (300 MHz) δ (32.26, CH3) (37.20, CH3) (46.97, CH2) (51.27, CH2) (116.41, CH) (123.30, CH) (140.28, CH) (161.49, CHO) (CF3 108.84, 116.71, 124.59, 132.46); HRMS (ESI): calcd for C8H14N3O (M+) 168.216, found: 168.218.
Spectral data of the synthesized compounds
3,4-Dihydro-naphthalene-1,2-dicarboxylic acid dimethyl ester (1)
1HNMR (400 MHz, CDCl3) δ 7.21 (d, 1H, 3 J = 7.48 Hz), 7.17 (q, 1H, 3 J = 4.47, 3 J = 7.29 Hz) 7.07(q, 1H, 3 J = 4.8 Hz, 3 J = 7.47 Hz), 3.75 (s, 3H), 3.73 (s, 3H), 2.80 (t, 2H, 3 J = 8 Hz), 2.95 (m, 2H) (t, 2H, 3 J = 8 Hz); 13CNMR (300 MHz, DCCl3): δ 171.93, 166.32, 134.30, 133.58, 128.48, 126.75, 126.34, 125.86, 123.94, 52.59, 52.30, 29.43, 26.01. HRMS (ESI): calcd for C14H14O4 (M+) 246.2586, found: 246.2585.
7-Methyl-3,4-dihydro-naphthalene-1,2-dicarboxylic acid dimethyl ester (2)
1HNMR (400 MHz, CDCl3) δ 7.32 (s, 1H), 7.16 (d, 1H, 3 J = 7.89 Hz) 6.68(d, 1H, 3 J = 7.89 Hz), 2.77 (t, 2H, 3 J = 8 Hz), 2.95 (t, 2H, 3 J = 8 Hz), 2.53(s, 3H); 13CNMR (300 MHz, DCCl3): δ 171.93, 166.32, 136.02, 135.68, 134.21, 130.62, 127.76, 127.24, 121.86, 52.59, 52.30, 29.43, 26.01, 21.70. HRMS (ESI): calcd for C15H16O4 (M+) 260.2851, found 260.2851.
5-Methyl-3,4-dihydro-naphthalene-1,2-dicarboxylic acid dimethyl ester (3)
1HNMR (400 MHz, CDCl3) δ 7.52 (d, 1H, 3 J = 7.70 Hz) 7.11(q, 1H, 3 J = 7.70 Hz, 3 J = 7.73 Hz), 7.02 (d, 1H, 3 J = 7.73 Hz), 3.75 (s, 3H), 3.73 (s, 3H), 2.96 (t, 2H, 3 J = 8 Hz), 2.85 (t, 2H, 3 J = 8 Hz), 2.33 (s, 3H); 13CNMR (300 MHz, DCCl3): δ 171.93, 166.32, 135.68, 135.36, 134.21, 133.38, 127.31, 126.92, 124.51, 52.59, 52.30, 26.42, 24.07, 19.30. HRMS (ESI): calcd for C15H16O4 (M+) 260.2851, found 260.2850.
7-Chloro-3,4-dihydro-naphthalene-1,2-dicarboxylic acid dimethyl ester (4)
1HNMR (400 MHz, CDCl3) δ 7.84 (d, 1H, 4 J = 2.36 Hz) 7.37 (m, 1H, 3 J = 9 Hz, 4 J = 2.36 Hz), 3.75 (s, 3H), 3.73 (s, 3H), 2.95 (t, 2H, 3 J = 8 Hz), 2.73 (t, 2H, 3 J = 8 Hz); 13CNMR (300 MHz, DCCl3): δ 171.93, 166.32, 134.91, 134.74, 128.81, 125.52, 119.86, 52.59, 52.30, 29.43, 26.01. HRMS (ESI): calcd for C14H13ClO4 (M+) 280.7033, found 280.7043.
5-Chloro-3,4-dihydro-naphthalene-1,2-dicarboxylic acid dimethyl ester (5)
1HNMR (400 MHz, CDCl3) δ 7.45 (d, 1H, 3 J = 2.36 Hz) 6.90 (m, 1H, 3 J = 7.73 Hz, 3 J = 8 Hz), 3.75 (s, 3H), 3.73 (s, 3H), 2.91 (t, 2H, 3 J = 8 Hz), 2.96 (t, 2H, 3 J = 8 Hz); 13CNMR (300 MHz, DCCl3): δ 171.93, 166.32, 134.91, 134.75, 133.26, 126.95, 126.72, 123.51, 52.59, 52.30, 26.22, 25.97. HRMS (ESI): calcd for C14H13ClO4 (M+) 280.7033, found 280.7030.
7-Nitro-3,4-dihydro-naphthalene-1,2-dicarboxylic acid dimethyl ester (6)
1HNMR (400 MHz, CDCl3) δ 8.44 (s, 1H) 8.17 (d, 1H, 3 J = 8.30 Hz), 7.53 (d, 1H, 3 J = 8.30 Hz), 3.75 (s, 3H), 3.73 (s, 3H), 2.77 (t, 2H, 3 J = 8 Hz), 2.95 (t, 2H, 3 J = 8 Hz); 13CNMR (300 MHz, DCCl3): δ 171.93, 166.32, 147.40, 137.52, 136.69, 135.92, 129.59, 128.48, 119.41, 118.98, 52.39, 52.59, 26.43, 26.01. HRMS (ESI): calcd for C14H13NO6 (M+) 291.2562, found 291.2561.
5-Nitro-3,4-dihydro-naphthalene-1,2-dicarboxylic acid dimethyl ester (7)
1HNMR (400 MHz, CDCl3) δ 8.04 (d, 1H, 3 J = 7.88 Hz), 7.96 (d, 1H, 3 J = 8 Hz), 7.20 (q, 1H, 3 J = 8 Hz, 3 J = 7.88 Hz), 3.75 (s, 3H), 3.73 (s, 3H), 3.35 (t, 2H, 3 J = 8 Hz), 3.15 (t, 2H, 3 J = 8 Hz); 13CNMR (300 MHz, DCCl3): δ 171.93, 166.32, 149.37, 136.69, 135.92, 128.66, 127.62, 127.33, 119.85, 52.59, 52.30, 27.99, 23.96. HRMS (ESI): calcd for C14H13NO6 (M+) 291.2562, found 291.2562.
3,4-dihydro-naphthalene-1,2,5-tricarboxylic acid dimethyl ester (8)
1HNMR (400 MHz, CDCl3) δ 12.7 (s, 1H), 8.07 (d, 1H, 3 J = 7.62 Hz), 7.61 (d, 1H, 3 J = 7.70 Hz), 7.34 (q, 1H, 3 J = 7.70 Hz, 3 J = 7.62 Hz), 3.75 (s, 3H), 3.73 (s, 3H), 3.26 (t, 2H, 3 J = 7.60 Hz), 2.51 (t, 2H, 3 J = 7.60 Hz); 13CNMR (300 MHz, DCCl3): δ 171.93, 166.32, 165.17, 135.68, 135.64, 128.53, 127.82, 127.30, 52.59, 52.30, 26.70, 25.19. HRMS (ESI): calcd for C15H14O6 (M+) 290.2681, found 290.2680.
5,7-dihydroxy-3,4-dihydro-naphthalene-1,2-dicarboxylic acid dimethyl ester (9)
1HNMR (400 MHz, CDCl3) δ 8.82 (s, 1H), 6.72 (d, 1H, 4 J = 2.20 Hz), 5.93(d, 1H, 4 J = 2.20Hz), 3.75 (s, 3H), 3.73 (s, 3H), 2.91 (t, 2H, 3 J = 8.75 Hz), 2.87 (t, 2H, 3 J = 8.75 Hz); 13CNMR (300 MHz, DCCl3): δ 171.93, 166.32, 159.27, 154.06, 136.59, 136.26, 128.48, 111.91, 101.47, 97.51, 52.39, 52.30, 26.36, 22.31.HRMS (ESI): calcd for C14H14O6 (M+) 278.2574, found 278.2574.
4-Methyl-3,4-dihydro-naphthalene-1,2-dicarboxylic acid dimethyl ester (10)
1HNMR (400 MHz, CDCl3) δ 7.20(m, 1H), 7.55(d, 1H, 3 J = 7.29 Hz), 7.19(d, 1H, 3 J = 8.02 Hz), 7.14 (q, 1H, 3 J = 7.29 Hz, 3 J = 4.47 Hz), 7.00 (q, 1H, 3 J = 8.02 Hz, 3 J = 4.47 Hz), 3.75 (s, 3H), 3.73 (s, 3H); 2.80 (m, 1H), 2.92 (d, 2H, 3 J = 7.32 Hz) 1.34 (d, 3H, 3 J = 6.99 Hz) 13CNMR (300 MHz, DCCl3): δ 171.76, 166.32, 136.61, 134.30, 127.82, 126.18, 126.14, 123.77, 52.59, 52.30, 32.87, 28.22, 19.73 HRMS (ESI): calcd for C15H16O4 (M+) 260.2851, found 260.2851.
7-Chloro-4-methyl-3,4-dihydro-naphthalene-1,2-dicarboxylic acid dimethyl ester (11)
1HNMR (400 MHz, CDCl3) δ 7.82(s, 1H), 7.35(d, 1H, 3 J = 9), 3.75 (s, 3H), 3.73 (s, 3H); 2.88(d, 2H, 3 J = 7.32), 2.77–2.80 (m, 1H), 1.34 (d, 3H, 3 J = 6.99). 13CNMR (300 MHz, DCCl3): δ 171.76, 166.32, 135.96, 134.91, 134.57, 134.40, 128.50, 125.58, 119.90, 52.59, 52.30, 32.87, 28.22, 19.73.HRMS (ESI): calcd for C15H15ClO4 (M+) 294.7299, found 294.7297.
6,7-Dihydro-1H-indene-4,5-dicarboxylic acid dimethyl ester (12)
1HNMR (400 MHz, CDCl3) δ 7.78(d, 1H), 6.47(m, 1H), 3.08 (d, 2H, 3 J = 2.60 Hz), 2.13 (t, 2H, 3 J = 8.16 Hz), 2.62 (t, 2H, 3 J = 8.16 Hz), 3.75 (s, 3H), 3.73 (s, 3H); 13CNMR (300 MHz, DCCl3): δ 168.82, 166.65, 148.87, 139.78, 133.73, 132.00, 129.49, 54.54, 51.87, 43.42, 24.84, 22.35. HRMS (ESI): calcd for C13H14O4 (M+) 234.2479, found 234.2476.
3,4,5,6-Tetrahydro-anthracene-1,2,7,8-tetracarboxylic acid tetramethyl ester (13)
1HNMR (400 MHz, CDCl3) δ 7.65(s, 1H), 7.23(m, 1H), 3.75 (s, 6H), 3.73 (s, 6H), 2.75–2.81(t, 6H, 3 J = 8 Hz), 2.93–2.99 (t, 6H, 3 J = 8 Hz); 13CNMR (300 MHz, DCCl3): δ 171.93, 166.32, 36.10, 135.68, 132.93, 128.48, 126.19, 118.34, 118.34, 52.59, 52.30, 29.84, 26.01. HRMS (ESI): calcd for C22H22O8 (M+) 414.4053, found 414.4053.
1,2-Dihydro-phenanthrene-3,4-dicarboxylic acid dimethyl ester (14)
1HNMR (400 MHz, CDCl3) δ 8.00 (m, 1H, 3 J = 8.27 Hz, 4 J = 1.50 Hz), 7.82(d, 1H, 3 J = 8.54 Hz), 7.49 (d, 1H, 3 J = 8.54 Hz), 7.37 (m, 1H, 3 J = 7 Hz, 4 J = 1.48 Hz), 7.77 (m, 1H, 3 J = 8.13 Hz, 4 J = 1.48 Hz), 7.50 (m, 1H, 3 J = 8.13 Hz, 4 J = 7 Hz), 3.75 (s, 3H), 3.73 (s, 3H), 3.01–3.99 (q, 2H, 3 J = 8 Hz), 2.83–2.89 (q, 2H, 3 J = 8 Hz); 13CNMR (300 MHz, DCCl3): δ 171.93, 166.32, 147.61, 136.81, 134.30, 128.64, 126.29, 125.73, 125.58, 123.52, 122.56, 52.59, 52.30, 29.43, 26.01. HRMS (ESI): calcd for C18 H18 O4 (M+), 296.3172, found 296.3171.
3,4,5,8-Tetrahydronaphthalene-1,2-dicarboxylic acid dimethyl ester (15)
1HNMR (400 MHz, CDCl3) δ 5.81–5.86 (m, 1H), 5.59–5.64 (m, 1H), 3.71(s, 3H), 3.26(s, 3H), 2.87 (d, 2H, 3 J = 2.78 Hz), 2.39 (d, 2H, 3 J = 2.78 Hz), 2.15 (q, 2H, 3 J = 7.80 Hz), 2.36 (q, 2H, 3 J = 7.80 Hz); 13CNMR (300 MHz, DCCl3): δ 169.20, 165.30, 147.44, 131.04, 124.72, 124.67, 52.11, 51.87, 31.55, 30.33, 25.27, 23.30.HRMS (ESI): calcd for C14 H16 O4 (M+), 248.2744, found 248.2742.
5,6-Dihydro-4H-chromene-7,8-dicarboxylic acid dimethyl ester (16)
1HNMR (400 MHz, CDCl3) δ 6.35 9d, 1H, 3 J = 8 Hz), 5.01 (m, 1H, 3 J = 8 Hz, 3 J = 3.23 Hz), 3.73 (s, 3H), 3.71 (s, 3H), 2.26(t, 2H, 3 J = 7.80 Hz), 2.40 (t, 2H, 3 J = 7.80 Hz); 13CNMR (300 MHz, DCCl3): δ 168.86, 164.69, 143.17, 138.93, 128.62, 127.60, 117.94, 99.93, 51.87, 51.43, 34.98, 24.70, 23.56. HRMS (ESI): calcd for C13H14O5 (M+) 250.2473, found: 250.2473.
5,6-Dihydro-quinoline-7,8-dicarboxylic acid dimethyl ester (17)
1HNMR (400 MHz, CDCl3) δ 8.35 (d, 1H, 3 J = 4.73 Hz), 7.65(d, 1H, 3 J = 8.50 Hz), 7.26(q, 1H, 3 J = 4.73 Hz, 3 J = 8.50 Hz), 3.73 (s, 3H), 3.71 (s, 3H), 2.76 (t, 2H, 3 J = 7.60 Hz), 2.81 (t, 2H, 3 J = 7.60 Hz); 13CNMR (300 MHz, DCCl3): δ 170.98, 160.74, 147.73, 145.79, 136.09, 129.11, 122.34, 52.30, 52.11, 28.07, 26.62. HRMS (ESI): calcd for C13 H13NO4 (M+) 247.2467, found: 247.2465.
6,7-Dihydro-benzofuran-4,5-dicarboxylic acid dimethyl ester (18)
1HNMR (400 MHz, CDCl3) δ 7.53(d, 1H 3 J = 2 Hz), 6.70(d, 1H, 3 J = 2 Hz), 3.75(s, 3H), 3.73(s, 3H), 2.70 (t, 2H, 3 J = 7.71 Hz), 2.80 (t, 2H, 3 J = 7.71 Hz); 13CNMR (300 MHz, DCCl3): δ 170.01, 161.58, 154.74, 143.02, 128.27, 125.19, 120.43, 104.67, 53.84, 51.87, 26.43, 24.23. HRMS (ESI): calcd for C12H12O5 (M+) 236.2207, found: 236.2206.
4,5-Dihydro-benzofuran-6,7-dicarboxylic acid dimethyl ester (19)
1HNMR (400 MHz, CDCl3) δ 7.60 (d, 1H 3 J = 2 Hz), 6.38(d, 1H, 3 J = 2 Hz), 3.75(s, 3H), 3.73(s, 3H), 2.39 (t, 2H, 3 J = 7.60 Hz), 2.92 (t, 2H, 3 J = 7.60 Hz); 13CNMR (300 MHz, DCCl3): δ 168.86, 164.69, 146.22, 139.22, 126.76, 126.76, 126.05, 125.46, 106.43, 51.87, 51.43, 25.42, 24.31. HRMS (ESI): calcd for C12H12O5 (M+) 236.2207, found: 236.2207.
General procedure for cycloaddition
To a solution of DMF-like ionic liquid 5g (3 mL) was added (0.3 mmol, 1 equiv) solution of vinylarenes in 2 ml dichloromethane dropwise within 15 min with constant stirring. After constant stirring for 1 h at low temperature, dimethylacetylenedicarboxylate (0.3 mmol, 1 equiv) was added and then the mixture was warmed to room temperature. Each type of the reaction mixture was then stirred for the required time and at the desired temperature. After completion of the reaction as evident from GC-MS, dichloromethane was removed using a rotary evaporator and the product from ionic liquid was extracted with diethyl ether (6 ml) four times. The ether extract was evaporated using a rotary evaporation in vacuo. The product residue was subject to flash column chromatography (silica gel 60 A, 230–400 mesh) using hexane and ethyl acetate as eluents to afford the product.
Conclusion
The DMF-based ionic liquid has been used as a solvent as well as a nucleophilic reagent for cycloaddition of various vinylarenes with dimethylacetylenedicarboxylates to yield 1,2-disubstituted 3,4-dihydronaphthalenes in best yield depending upon the nature of the substrate The ionic liquid nature of DMF allows to perform the reaction under green conditions with easy operational procedure, facile workup. Less usage of chemicals render this methodology to be cost effective and less hazardous. The best performance of the wide structural diversity of vinylarenes proves the wider scope of this novel procedure.
Acknowledgements
The authors acknowledge spectroscopic and GC-MS facilities extended by H.E.J International Centre for Biological and Chemical Sciences, University of Karachi, Pakistan.
Disclosure statement
No potential conflict of interest was reported by the authors.
Notes on contributors
Ahmed Ali Hullio graduated with Ph.D. degree in Organic chemistry from University of Sindh, Pakistan. He has carried out research projects in ionic liquid based organocatalyst. He has 15 years of teaching experience as a lecturer and Assistant professor in Pakistan. Mr. Hullio has more than 10 research papers in various international journals. She is currently working as an Assistant professor of organic Chemistry at Sindh University.
Hassan Imran Afridi graduated with Ph.D. degree in Analytical chemistry from Sindh University, Pakistan. He has carried out research projects in trace metal analysis. He has more than 10 years of teaching experience. Mr. Afridi has more than 80 research papers in various national and international journals. He is currently working as assistant professor of Analytical Chemistry at National centre of excellence in Analytical Chemistry, Sindh University.
Ghulam Murtaza Mastoi graduated with Ph.D. degree in physical chemistry from Sindh University, Pakistan. He has carried out research projects in water analysis by new atomic spectrophotometric techniques. He has more than 30 years of teaching experience. Mr. Mastoi has more than 75 research papers in various national and international journals. He is currently working as professor of Physical Chemistry at Sindh University.
Additional information
Funding
References
- Hullio, A.A.; Mastoi, G.M. Oriental J. Chem. 2011, 27 (4), 1591–1612.
- Jiang, J.L.; Hua, R. Org. Biomol. Chem. 2007, 5, 1854–1857. doi: 10.1039/b706768b
- Muzart, J. Tetrahedron Lett. 2009, 65 (40), 8313–8323. doi: 10.1016/j.tet.2009.06.091
- Dus, S.; Panigrahi, A.K.; Maikap, G.C. Tetrahedron Lett. 2003, 44, 1375–1377. doi: 10.1016/S0040-4039(02)02885-X
- Abad, A.; Agullo, C.; Cunal, A.C.; Navarro, I. Synthesis 2005, 19, 3355–3361. doi: 10.1055/s-2005-918453
- Cao, L.J.; Jiang, B.; Li, C.M.; Shao, Q.Q.; Shi, F.; Tu, S.J.; Zhou, D.X. Synthesis 2008, 3, 369–376.
- Langer, P.; Wuckelt, J.; Doring, M.; Schreiner, P.R.; Gorls, H. Eur. J. Org. Chem. 2000, 12, 2257.
- Anderson, J.L.; Armstrong, D.W. Anal. Chem. 2003, 75, 4851–4858. doi: 10.1021/ac0345749
- http://www.frcepalenfisonline.com/499254Lhiml.
- Hass, H.B.; Bender, M.L. J. Am. Chem. Soc. 1949, 71, 1767–1769. doi: 10.1021/ja01173a066
- Hullio, A.A.; Mastoi, G.M. Chin. J. Chem. 2012, 30 (7), 1647–1657. doi: 10.1002/cjoc.201280028
- Hullio, A.A.; Mastoi, G.M. Asian J. Chem. 2011, 23 (12), 5411–5418.
- Sukanta, B. Synth. Commun. 1995, 25 (14), 2061–2069. doi: 10.1080/00397919508015887
- Hiegel, G.A.; Rubino, M. Synth. Commun. 2002, 32 (17), 2691–2694. doi: 10.1081/SCC-120006034
- Reddy, G.; Kishore, G.D.; Baskaran, S. Tetrahedron Lett. 2000, 41 (47), 9149–9151. doi: 10.1016/S0040-4039(00)01636-1
- Jairton, D.; Crestina, S.; Consorti, P.; Suarez, A.Z.; de Souza, R.F. Org. Synth. 2004, Coll.2002, 79, 236, 184.
- Hea, X., Chan, T.H. Tetrahedron 2006, 62 (14), 3389–3394. doi: 10.1016/j.tet.2006.01.045