ABSTRACT
This article takes a look at some of the broader themes of value creation that have evolved as part of a circular economy, and illustrates why chemists should (as well as exemplifying how they could) get involved. We begin by outlining five main lessons we have learned since the Ellen MacArthur Foundation was set up in 2010, and then proceed to talk about two concrete examples where the circular economy offers a vision of a system with positive socio-economic and environmental outcomes: mobility and plastics. In each case, we discuss the role chemistry, in particular green chemistry, can play – anchored in the realization that chemists play a central role in guiding society towards its material future.
GRAPHICAL ABSTRACT
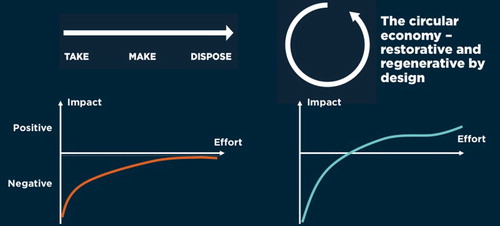
Introduction
Emerging in the past few decades from several schools of thought, such as Cradle2Cradle (Citation1), Biomimicry (Citation2), and the Performance economy (Citation3), the circular economy has recently become a widespread concept. Envisaging an economy that is restorative and regenerative by design, the key aims of a circular economy are to eliminate the concept of waste, rebuild natural capital and create economic value by using – not consuming – resources effectively.
Arguably, the intuitiveness of how the circular economy proposes to address urgent environmental and resource issues, combined with its compelling case for economic value creation (Citation4) is the reason the idea has recently been taken up. Not surprisingly, businesses and policymakers showed early interest, notably the European Commission (whose circular economy package was adopted on 2nd December 2015 (Citation5)) and China. The latter instigated what has grown to become a comprehensive circular economy package as early as 2005, in its 11th 5-year plan (Citation6). China’s circular economy development strategy was recently updated, and now includes measures to make urban systems (including mobility) more circular (Citation7). Many now regard a circular economy framework as a key approach to achieve certain of the United Nations Sustainable Development goals (Citation8), for example goal 12 (ensure sustainable consumption and production patterns) (Citation9).
In parallel, the scientific discourse about the circular economy has grown exponentially (). While still a small topic in terms of absolute number of publications, the rapid increase in papers is a clear indication that the circular economy is transitioning from the scientific fringe to the mainstream (Citation10). From being viewed as a semi-novel way of thinking about waste management, the circular economy is increasingly recognized as a systemic redefinition of how we organize sourcing, manufacturing, and utilization of materials and assets. Yet, it is a cross-disciplinary field that is still in its infancy, and as an entirely new approach to the economy it is in a “validity challenge period (Citation11).” There is still no broad consensus as to what exactly a circular economy entails, or to what extent a transition can be organic or needs to be guided by policy.
Figure 1. Number of published articles and reviews including “circular economy” in title, abstract, or keywords. Source: the Scopus database, www.scopus.com (accessed Aug 21, 2017). *Publications to date.
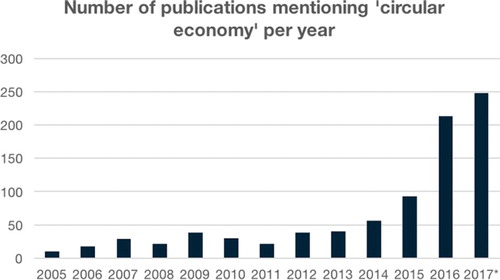
While an ongoing critique of concepts and definitions is welcome, a more direct way of engaging with the issue is to start solving the myriad practical and technological problems needed to realize a circular economy vision. Chemistry is at the heart of all material systems, and green chemistry (Citation12) provides an excellent framework to create systems consistent with a circular economy (consider especially the principles prevent waste, optimize atom economy, use non-hazardous components, use renewable feedstocks, design for degradation).Footnote1 The role of the chemist in a circular economy has recently been extensively reviewed (Citation13), and this article seeks to add some perspectives based on five key lessons we have learned since the Ellen MacArthur Foundation started promoting the circular economy in 2010. Against the backdrop of these lessons, we will dive into a couple of examples illustrating how we – by envisioning a systemic change – will uncover new opportunities and challenges for chemists that go beyond optimizing resource productivity in the current system.
Five important lessons
The linear economy is ripe for disruption. Today’s ‘take-make-dispose’ economy is enormously wasteful. Most of the materials we use, we lose, and the things we make are consistently under-utilized. Our efforts to remedy the problems have generally focused on treating the symptoms, not the cause. Such efforts will only lead to ‘less bad’ outcomes, and will almost certainly not be able to achieve an economy with net positive socio-economic and environmental impacts ((a)).
While the linear economy has brought great prosperity to many parts of the world, its shortcomings have resulted in a heavy environmental toll that is increasing exponentially. We have already overshot many planetary boundaries (Citation14) and we need to find exponential solutions. The inherent wastefulness of the economy means money is being left on the table every day – this is an untapped multi-trillion-dollar restorative and regenerative economic opportunity. For chemists, it becomes important to contrast efforts devised to mitigate the negative impacts of the extractive use of resources with new approaches that unlock the opportunity to better allocate and preserve these resources. For example, while there are clear benefits of better water treatment technology to clean the enormous volumes of contaminated water from textile dyeing, perhaps a strategy with more long-term viability is to invest in technologies designed to reduce the need for dyes, water, and other solvents in the first place, or better still ensure these substances fit the system and circulate safely and effectively within it (Citation13).
A circular economy holds the promise of new prosperity that is restorative and regenerative by design. A circular economy aims to eliminate the concept of waste and pollution, providing a model for a future in which waste simply does not exist. It takes a systems perspective, and presents opportunities for more inclusive economic growth, recognizing the importance of the economy needing to work effectively at all scales: for big and small businesses, for organizations and individuals, globally and locally. In this model, economic activity actually builds and rebuilds overall system health.
Achieving a circular economy rests on three principles:
Designing systems that work, eliminating waste and pollution;
Keeping products and materials at their highest value and in use;
Regenerating natural resources and restoring finite materials to be used again.
A circular economy gives purpose to the digital transformation. Digital technology enables a fundamental shift in the way the economy works and how it can develop in the future. It holds possibilities for radical de-materialization (of everything from print media to chemical experiments). It enables greater transparency on product use and material flows (knowing the composition and history of the things we make and use). Importantly, it opens new ways of operating and participating in the economy – for producers, users, and consumers (one can, for example, envisage distributed ledger technology that enables an intricate reverse logistics network to retrieve products for remanufacturing). It opens a pathway towards a vastly more distributed, personalized, and networked economic future that fundamentally changes the way we design, make, and use things (Citation19). A circular economy mindset is important for chemists to guide this unstoppable digital transformation to a pathway that is mutually beneficial for the economy and the environment. For example, how can we embed information in materials without compromising our ability to recycle them?
A circular economy offers substantial benefits for business, society and the environment. Growth in a circular economy means moving away from creating value in depletive and extractive ways, and instead growing and capturing the opportunities presented by the restorative and regenerative parts of the economy. Growth in a circular economy also brings environmental benefits, such as improving air and water quality, regenerating ecosystems and soils, dramatically lowering water and energy demands, and reducing greenhouse gas (GHG) emissions. This approach to value creation could liberate us from being bound to only reduce the negative impact of economic activities, and instead create a virtuous cycle leading to a net positive impact for the economy, environment, and society ((b)).
Greater profits await innovative businesses that decouple from resource constraints (Citation20), since by doing so they increase their resilience and build better customer relationships. Hundreds of companies (including multinationals such as Unilever, Nike, and IKEA) are already exploring this opportunity (Citation21). By scaling up to an economy-wide system of synergistic products and services, such companies can generate trillions of dollars of value. But while the economic opportunity is well documented (Citation22–24), the technical questions of how we capture them are still largely unanswered. In addition, the transition pathway is fraught with the risk of propagating an even more extractive economy despite our best intentions. For example, consider how technologies to extract valuable chemicals and materials from biomass might simply lead to increased net output rather than replacing production from finite feedstocks. The risk of such a “circular economy rebound” (Citation25) highlights the imperative of careful systems-level design when considering how to apply novel technologies.
A circular economy presents an inspiring vision for an economy that works. It provides a new direction of travel that embraces the reality of systems thinking and complexity. The challenges of getting there are staggering, and nobody – individually or collectively – has all the answers for what the complete vision looks like. However, we are now at an exciting inflection point where the vision (Citation26) is clear and compelling enough to overcome these hurdles. Chemists know the challenges better than most. With over 50 million human-made chemicals and materials registered in the Chemical Abstracts Service database, and nearly 100,000 on the market, how can we design a system that captures the added value of molecules, polymers, and complex materials over and over again? How can we reconcile the ingenuity of chemists, which results in the discovery of a new substance on average every 2.6 seconds (Citation27), with the notion of harmonized flows of pure materials that can be reused? We do not have all the answers yet. But we cannot build the economy we need if we cannot imagine it. It is time to start imagining, and that is precisely why chemists are crucial to the transition to circular economy (Citation28).
Figure 2. (a) A linear economy. Efforts to reduce negative impact can in theory only reach a ‘zero negative’ limit as long as the system does not change to be regenerative by design. (b) A circular economy framework aims to design an economic system in which our activities have a net positive impact on our environment, health, economy, and society as a whole.
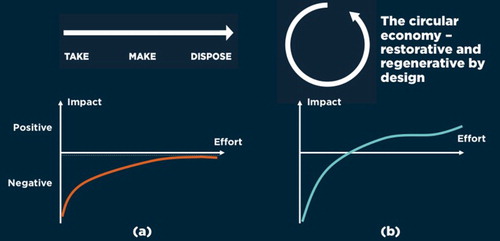
To take a deeper look at how we can apply these five lessons in practice, it is instructive to consider individual economic sectors (broadly defined) and materials systems. The two examples below – the mobility sector and plastic packaging – are areas in which the Ellen MacArthur Foundation has spent substantial time in exploring systemic shifts.
Mobility
The current mobility system, with cars as the primary mode of transport, suffers from tremendous structural waste: the average European car is parked 92% of the time, and only five of the remaining percentage points are spent going somewhere; the rest are wasted in congestion and looking for parking. Of the car’s five seats, one-and-a-half are occupied on average – a utilization rate of 30%. The dead-weight ratio of a 1400 kg car with 1.5 passengers is roughly 12:1 and, since some 86% of the energy from the fuel it burns never reaches the wheels due to losses from the engine and to idling, only 1 cl of each liter of fuel ends up moving people. In addition, the average city sets aside half its land, some of it prime real estate, to the infrastructure needed to drive and park cars (Citation22).
We are so used to cars being a ubiquitous part of society that we rarely think about what a hopeless investment case they are overall. But imagine we applied the three principles of a circular economy to the mobility system – giving us cars that are shared not owned, automated, electrified, and used on-demand? The reduction in structural waste – as well as improvement in productivity and quality of life – could be enormous.
Automation means cars can truly be operated as a service, minimizing idle time. While in the car, we can work or relax, reducing both productivity losses and the societal cost associated with stress-related health issues. Electrification makes cars more energy efficient and reduces local air pollution, reducing health-related costs. During off-peak hours – in the middle of the day and at night – electric cars can also act as useful storage capacity in a smart solar power grid (during the day batteries store excess power and at night, when generation is minimal, they can act as backup capacity) (Citation29).Footnote2
Mobility-as-a-service also completely changes the incentives around building cars. Suddenly, they would need to be more robust, easier to repair, and designed to be re-manufactured – and we would need fewer of them. As a result, demand for primary resources to make cars would significantly decouple from the economic and societal value they create. And by the way, tremendous quantities of land could be unlocked by the reduced need for space for driving and parking, leaving room to make cities denser, more efficient, and more liveable.
One discovers similar opportunities for radical innovation wherever you look, from energy infrastructure to waste management. A report by the Ellen MacArthur Foundation and McKinsey & Company (Citation22) found that redesigning, using the circular economy principles, the three largest segments in terms of European household expenditure (mobility, food, and the built environment) could save households a massive EUR 1.8 trillion by 2030 vs. a baseline scenario. Doing so would reduce primary resource consumption and GHG emissions by 10% and 17% respectively, and at the same time boost growth.
Such a shift cannot happen by itself, but needs significant investment (Citation30) as well as, importantly, technological innovation. There is an abundance of opportunities for chemists and engineers to dive into here. Looking at the mobility example again, an on-demand, automated electric vehicle will cover many more miles per year, which completely changes the specifications for engine and bodywork durability. Light-weighting becomes even more crucial, as electrified cars are heavier – a fertile ground for novel technical materials (some of which are already starting to be derived from biological feedstocks (Citation31)). More importantly, we need reliable technologies to recover the high-performance components when they need replacing.
Plastics
Without a doubt, plastics bring many benefits, and society as we know it would not be able to work without them. They are cheap and high-performing, which is why their use has increased 20-fold in just half a century. But it is becoming increasingly evident that the plastics system is broken (). Globally, only 14% of plastic packaging is collected for recycling, with even less actually making it back into applications. This represents a loss of USD 80–120 billion worth of material to the global economy every year (Citation32).
Even more troubling is that a staggering one-third of all plastic packaging leaks into the environment. The rest is either landfilled or incinerated (with associated adverse health and environmental impacts). A conservative estimate from the UN Environment Programme estimated the negative externalities of the linear plastics system to be USD 40 billion per year – more than the industry’s entire profit pool (Citation33). Recent work on the rate of plastics entering the marine environment (Citation34) has shed light on the severity of the problem despite the difficulties quantifying it. Despite many ongoing efforts to recover ocean plastics on and off coastlines, it appears that almost all plastic that has already entered the oceans are beyond the reach of such efforts (Citation35). The current rate of addition of plastics to the environment also means that even large-scale clean-up activities will be ineffective in preventing the environmental and socio-economic impacts of this leakage (Citation36). Instead, we need to design a better plastics system.
In contrast to a carmaker, a manufacturer of plastics currently has very limited opportunity to monitor or control what happens to their product after it leaves the factory. Because different polymers tend to get co-mingled, and because of their low cost, the chances of making even a small profit by recovering them in today’s system are slim. Add to that an industry in which, over the decades, thinking has become more siloed and areas of expertise narrower, and it is easy to see why, roughly 40 years after the introduction of the recycling symbol, global reuse and recycling rates are so low.
But we cannot build the system we need if we cannot imagine it. The circular economy offers a framework to rethink the plastics system and create an attractive vision for a plastics economy that works. The New Plastics Economy,Footnote3 which has an initial focus on plastic packaging, is an ambitious, three-year initiative to build momentum towards this vision, based on three core ambitions ():
Create an effective economy for after-use plastics. This is the cornerstone of a New Plastics Economy. Achieving it will require many different factors working together, but if scaled-up it will also help achieve the other two ambitions.
Drastically reduce negative impacts (such as leakage into the environment). These impacts are unacceptable symptoms of a broken system, and need fundamental upstream solutions.
Decouple plastics from fossil feedstocks. While effective material loops are always the goal in a circular economy, some materials will escape, for example by biodegradation. It is important that in the long term virgin feedstocks are supplied from materials made from sequestered carbon.
To achieve these ambitions, green chemistry principles are a great place to start. As in the mobility example, a new system needs new technologies, and there are plenty of innovation gaps to bridge to realize a new plastics economy vision, for example:
Preventing waste – there is an opportunity to invent new materials to replace those that cannot get reused or recycled. Developing better chemical recycling processes, separating polymers from additives and replenishing monomers or feedstocks, would at the same time enable higher value material loops. One crucial question for chemists to answer is how to design these chemical recycling systems, given the range of options at hand: from recovering specific materials in isolation (Citation37) to integrating them into a more general cycle of platform chemicals (Citation38).
Using renewable feedstocks with good atom economy – a step-change in renewably sourced polymers would be necessary to enable the long-term vision of de-coupling plastics from fossil feedstocks.
Designing for degradation where appropriate – there are several use cases for high-performing, biodegradable materials, such as applications where co-mingling with organic materials is a likely after-use pathway.
The New Plastics Economy recently published a report Catalysing Action (Citation39), in which we outlined three widely endorsed strategies to start the transition from a linear to a circular plastics economy. It found that, while recycling and reuse can be economically attractive for 70% of all plastics using current or nascent technologies, 30% of plastic packaging by weight (>50% of items) by design is destined to become waste. These items will never be recycled unless radically redesigned or re-invented.
Such items fall into two main categories. The first is small-format items like sachets, tear-offs, and lids. These either do not get collected in the first place or get sorted out because they are too small. The second is multi-layer, multi-material laminates, as used in crisp bags, pouches, and sachets. Separating these layers is technically difficult and very costly compared to the value of the material recovered.
Items like these obviously play an important role in getting products to people and enabling global supply chains, and a multitude of innovative solutions have gone into rendering them lighter, cheaper, and more high-performing. But the catch is that all these innovations have a linear plastics economy in mind, with no system-wide framework to guide new ideas towards the goal of creating a plastic system that works overall. Consequently, their rates of reuse and recycling are, and are expected to remain, vanishingly low.
It is true that any packaging system should be designed to optimize overall environmental impact, for which a life cycle assessment (LCA) approach is the main established method. However, the fact that many products, notably food (Citation40), have such a large environmental impact often leads to the result that unrecycled packaging waste makes sense from an LCA perspective if it prevents the wasting of them. This artifact of LCA could partly be explained by the difficulty of accounting for the total environmental impact of plastics in the environment: there is, for example, no reliable measure of the socio-economic costs of the millions of tonnes of marine plastic particles and their impact on ecosystems. Furthermore, the high environmental impact of food production is in itself a consequence of a linear and extractive economy; the circular economy envisions a regenerative food system with a much lower baseline impact (Citation17 , Citation22 , Citation24). In such a system, the LCA outcome may be different. Science will play a critical role in exploring these issues further.
Based on these arguments, the categories of plastic packaging discussed above are excellent test cases for system-wide innovation. To stimulate such innovation, we recently launched the $2 million New Plastics Economy Innovation Prize (Citation41). Recognizing that this is only one of many efforts needed enable a circular economy for plastics, it is a start of a journey focusing on system-wide solutions, which hopefully leads to intensified effort with a revised perspective, both in academia and business.
Conclusion
This article has sought to illustrate why the linear economy is ripe for disruption and why chemists equipped with the tools of green chemistry, and supported by the vision of a circular economy, have a central role to play in rethinking it. Whether the reader is a student or a professional, they may find themselves, now or in the near future, working in an industrial system that likely brings many benefits. But they may also notice important drawbacks, just like we have seen with mobility and plastics.
We should remember that we can do more than just use less – we can reinvent the future of our society. By rethinking the current model, we can create a positive vision of a system that works. And as we have seen, imagining the system we want is the first step on a path towards a better future. Chemists, in particular green chemistry practitioners, are very well placed to act as expert guides on that journey.
Acknowledgements
The author thanks Ian Banks for valuable comments and copyediting support, and to the three reviewers for valuable input on a draft version of this paper. The arguments and viewpoints put forward in this article represent those of the author and are not necessarily the position of the Ellen MacArthur Foundation.
Notes on contributor
Mats Linder leads the innovation programme of the New Plastics Economy initiative, which is run by the Ellen MacArthur Foundation. He holds a PhD in Physical Chemistry from the Royal Institute of Technology in Stockholm.
Notes
1. Designing for (biological) degradation is often not a preferred option, as pointed out by many authors. Metabolic decomposition invariably leads to losses (e.g. carbon dioxide and water) without much value capture, and methods to preserve materials should be favored when possible. For example, designing for chemical recycling (i.e. the decomposition to new chemicals) would be more productive (see, e.g. Citation14 , Citation39). There are, however, special cases where biodegradation if helpful to avoid bio-incompatible (and sometimes toxic) substances ending up as pollution.
2. For example, a “smart solar charging network” pilot was started in Utrecht, NL, in 2016, and included partners like the City Council and Renault.
3. The initiative is led by the Ellen MacArthur Foundation in collaboration with a broad group of leading companies, cities, philanthropists, policy-makers, academics, students, NGOs, and citizens. Available from: www.newplasticseconomy.org.
References
- McDonough, W.; Braungart, M. Cradle to Cradle: Remaking the Way we Make Things;North Point Press: New York, 2002.
- Benyus, J. Biomimicry: Innovation Inspired by Nature;HarperCollins: New York, 2002.
- Stahel, W.H. Nature. 2016, 531, 435–438. doi: 10.1038/531435a
- Ellen MacArthur Foundation, Towards a Circular Economy vol. I. 2012.
- European Commission, Report from the Commission ( … ) on the Implementation of the Circular Economy Action Plan. Jan 26, 2017. http://ec.europa.eu/environment/circular-economy/implementation_report.pdf (accessed Aug 24, 2017)
- Mathews, J.A; Tan, H. Nature. 2016, 531, 440–442. doi: 10.1038/531440a
- China Association of Circular Economy. May 4, 2017. http://www.chinacace.org/news/view?id=8261 (accessed Aug 24, 2017)
- United Nations, Sustainable Development Goals: 17 Goals to Transform Our World. http://www.un.org/sustainabledevelopment/sustainable-development-goals/ (accessed Aug 24, 2017)
- United Nations, Partnerships for SDGs. https://sustainabledevelopment.un.org/partnership/search/?menu=1629&str=circular+economy (accessed Aug 24, 2017)
- Bocken, N.M.P.; Olivetti, E.A.; Cullen, J.M.; Potting, J.; Lifset, R. J. Ind. Ecol. 2017, 21, 476–482. doi: 10.1111/jiec.12606
- Blomsma, F.; Brennan, G. J. Ind. Ecol. 2017, 21, 603–614. doi: 10.1111/jiec.12603
- Anastas, P.T.; Warner, J.C. Green Chemistry: Theory and Practice, Oxford University Press: Cary (NC), 2000.
- Clark, J.H.; Farmer, T.J.; Herrero-Davila, L.; Sherwood, J. Green Chem. 2016, 18, 3914–3934. doi: 10.1039/C6GC00501B
- Steffen, W.; Richardson, K.; Rockström, J.; Cornell, S.E.; Fetzer, I.; Bennett, E.M.; Biggs, R.; Carpenter, S.R.; de Vries, V.; de Wit, C.A.;et al. Science. 2015, 347, 1259855. doi: 10.1126/science.1259855
- Midgley, G., Ed. Systems Thinking vol. 1–4;Sage: London, 2002.
- Whitesides, G.M. Angew. Chem. Int. Ed. 2015, 54, 3196–3209. doi: 10.1002/anie.201410884
- Toensmeier, E.; Blake, A. Perennial Industrial Crops: Green Chemistry and Carbon Sequestration. In Handbook of Ecomaterials; Martinez, E., Kharisova, O. Kharisov, B., Eds. Springer (accepted, publication expected 2017; https://meteor.springer.com/ecomaterials/?id=550&tab=About&mode=ReadPage&entity=3787)
- Zuin, V. Curr. Opin. Green Sus. Chem. 2016, 2, 40–44.
- Ellen MacArthur Foundation and World Economic Forum, Intelligent Assets: Unlocking the Circular Economy Potential. 2016.
- Ellen MacArthur Foundation, Towards the Circular Economy vol. II. 2013.
- Circular Economy 100 Programme. https://www.ellenmacarthurfoundation.org/ce100/ (accessed Aug 24, 2017)
- Ellen MacArthur Foundation, SUN, McKinsey Center for Business and Environment;Growth Within: A Circular Economy Vision for Europe. 2015.
- Club of Rome, The Circular Economy and Benefits for Society. 2016.
- Ellen MacArthur Foundation, Circular Economy in India: Rethinking Growth for long-Term Prosperity. 2016.
- Zink, T.; Geyer, R. J. Ind. Ecol. 2017, 21, 593–602. doi: 10.1111/jiec.12545
- Ellen MacArthur Foundation, Circular Economy Overview. https://www.ellenmacarthurfoundation.org/circular-economy/overview/characteristics (accessed Aug 24, 2017)
- Madrigal A. Wired. 2009 September 9.
- Clift, R. Why Chemical Engineers – Not Just Economists – Are Key to a Circular Future. GreenBiz. June 23, 2017. https://www.greenbiz.com/article/why-chemists-not-just-economists-are-key-circular-future (accessed Aug 24, 2017)
- Renault. The Smart Solar Charging Network Project. March 11, 2016. http://media.renault.com/global/en-gb/renaultgroup/Media/PressRelease.aspx?mediaid=76330 (accessed Aug 24, 2017)
- Ellen MacArthur Foundation, SUN, SystemIQ, Achieving Growth Within: A €320-billion Circular Economy Investment Opportunity Available to Europe up to 2025. 2017.
- O’Dowd, E. Mazda Exhibits its First Car Made with a Bioplastic Exterior. Bio-Based World News. Jan 8, 2017. https://www.biobasedworldnews.com/mazda-exhibits-its-first-car-made-with-a-bioplastic-exterior (accessed Aug 24, 2017)
- Ellen MacArthur Foundation, World Economic Forum and McKinsey & Company, The New Plastics Economy – Rethinking the Future of Plastics. 2016.
- United Nations Environment Programme, Valuing Plastics: The Business Case for Measuring, Managing and Disclosing Plastic Use in the Consumer Goods Industry. 2014.
- Jambeck, J.R.; Geyer, R.; Wilcox, C.; Siegler, T.R.; Perryman, M.; Andrady, A.; Narayan, R.; Lavender Law, K. Science. 2015, 347, 768–771. doi: 10.1126/science.1260352
- Moss, E.; Eidson, A.; Jambeck J. Sea of Opportunity: Supply Chain Investment Opportunities to Address Marine Plastic Pollution. Encourage Capital on behalf of Vulcan, Inc, New York, 2017.
- Ocean Conservancy, The Next Wave: Investment Strategies for Plastics Free Seas. 2017.
- Hong, M.; Chen, E.X.-Y. Green Chem. 2017, 19, 3692–3706. doi: 10.1039/C7GC01496A
- Antonelli, E.; Iaquaniello, G.; Salladini, A.; Spadaccini, L.; Perathoner, S.; Centi, G. ChemSusChem. 2017, 10, 912–920. doi: 10.1002/cssc.201601555
- Ellen MacArthur Foundation and World Economic Forum, The New Plastics Economy – Catalysing Action. 2017.
- Williams, H.; Wikström, F. J. Cleaner Prod. 2011, 19, 43–48. doi: 10.1016/j.jclepro.2010.08.008
- New Plastics Economy Innovation Prize. www.newplasticseconomy.org/innovation-prize (accessed Oct 17, 2017)