ABSTRACT
A set of N-substituted pyrrole derivatives have been designed and synthesized using sulfonated multi-walled carbon nanotubes as a recyclable heterogeneous catalyst under ultrasound irradiation. This reaction was carried out between 2,5-dimethoxy tetrahydrofuran and primary amines in water under green conditions. This method has some advantages such as: short reaction times, excellent product yields, simplicity of the procedure, easy work-up and high purity of products.
GRAPHICAL ABSTRACT
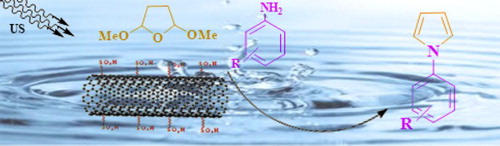
Introduction
Pyrroles are among the most important heterocyclic compounds with diverse biological activities and pharmaceutical agents (Citation1). They are found as the fundamental structural origin in various classes of natural and biologically important compounds such as coenzymes, porphyrins, bile pigments and alkaloids (Citation2–7). They are also precious intermediates in organic reactions (Citation8). Consequently, a wide range of methods have been devised for the synthesis of pyrroles. However, many of the methods are included such as: Paal–Knorr reaction (Citation9,Citation10), aza-Michael addition (Citation11) and multicomponent reactions (Citation12). In recent years, the synthesis of pyrrole derivatives has been reported in the presence of different catalysts such as: Bi(NO3)3.5H2O/MW (Citation13), molecular iodine/MW (Citation14) and PMA/SiO2 (Citation15), Fe2O3-SO3H on Fe3O4/MW (Citation16), H3PW12O40 on SiO2/MW (Citation17), nano FGT/H2O/MW (Citation18,Citation19), γ-Fe2O3@SiO2-Sb-IL/Reflux (Citation20), Bi(NO3)3.5H2O/US (Citation21).
Although these reported works are convenient methods for the preparation of pyrroles, the present method compared with the used catalysts has some advantages such as thermal and chemical stability, non-flammability properties, high activity, simplicity of the procedure, easy reaction work-up, recyclability, cleans method and resulting products with high purity.
Most of the catalysts contain metals or metal complexes supported on a material with suitable surface area as support. The most important characteristics of a support are the surface area, chemical composition, electronic, mechanical and stability properties. Until now, many allotropes of carbon have been discovered such as graphene, fullerene and carbon nanotubes (CNTs). They are used as support for the synthesis of heterogeneous catalyst. Chemical modification of CNTs is a method for enhancing their usages such as catalytic applications (Citation22), medicine (Citation23), optical devices (Citation24) and electronic instruments. These applications made them a significant material in science and technology. Chemical oxidation of nanotubes is generally carried out using gaseous or liquid oxidants. A more general procedure used for CNTs oxidation is that of using oxidizing agents in aqueous solutions. Oxidation of MWCNTs using acid-sonication has been studied by Xing et al., who demonstrated that the dispersion stability and solubility of the treated MWCNTs in water improve significantly (Citation25). Also, the ozone in the presence of an oxidative ozonide cleavage reagent (H2O2) has been reported as a good oxidizing agent (Citation26).
The application of ultrasonic irradiation in organic reactions has made progress in recent years. Sonication increases the reaction rate and eschews the use of high reaction temperatures (Citation27). The advantages of the ultrasound-assisted method such as short reaction times, good yields and mild reaction conditions are well documented (Citation28). The effects of ultrasonic irradiation obtained during organic reactions are due to cavitations. In the case of volatile component holes are trusty to act as micro reactor; as the volatile components enter the micro bubbles, high temperature and pressure are produced during breaking of their chemical bonds hence reacting with other species (Citation29–31). The presence of ultrasound irradiation in the liquid–liquid system, cavitational falling near the liquid–liquid juncture interrupts the interface and induce of one liquid into the other formed emulsion, and leads to a dramatic increase in the interfacial contact area across which transfer of species can take place.
In continuation of our recent work on using the ultrasound in organic synthesis (Citation32–35) and catalytic reaction (Citation36), herein we hope to report an efficient ultrasound-assisted eco-friendly method for the synthesis of N-substituted pyrroles by treatment of 2,5-dimethoxy tetrahydrofuran and various primary aromatic amines in the presence of sulfonated multi-walled CNTs as a heterogeneous catalyst in aqueous media.
Results and discussion
Preparation and characterization of the catalyst
The preparation of the MWCNTs-SO3H catalyst was performed in two steps as described in Scheme 1. In the first step, MWCNTs were converted to MWCNTs-COOH with HCl (37%) and HNO3 (63%). This step was carried out in order to purify of MWCNTs and to remove fullerene, amorphous carbon and metal particles such as Fe, Co, Ni and increase the reactivity (Scheme 1). This step also introduced oxygen comprising groups, mainly carboxyl groups on the MWCNTs (Citation37,Citation38). After this step, sulfuric acid (98%) was added to the MWCNTs-COOH to obtain the MWCNTs-SO3H. The prepared catalyst was revealed by scanning electron microscopy (SEM), X-ray powder diffraction (XRD), FT-IR, BET, electron dispersive X-ray (EDX) and X-ray photoelectron spectroscopy (XPS) spectroscopy (Figures 1S−6S and Table 1S in the Supporting Information) (Citation39,Citation40).
The reaction of 2,5-dimethoxy tetrahydrofuran with different types of primary aromatic amines in 1.2:1 mole ratio has been studied in the presence of MWCNT-SO3H as the heterogeneous catalyst under ultrasonic irradiation at a power of 65 W in water as a solvent (Scheme 2).
In the first step, the optimization of the catalyst amount in the reaction was carried out. The reaction of 2,5-dimethoxy tetrahydrofuran with 4-methoxy aniline with 1.2:1 mol ratio was done with different amounts of catalyst under ultrasonic irradiation at a power of 60 W and water as the solvent. The obtained results are presented in . Taking into consideration the results in this table, the optimum amount of used MWCNT-SO3H as the catalyst in this reaction was obtained: 0.02 g (, entry 2). After the optimization of the catalyst amount, the reaction was carried out in water under ultrasonic irradiation at different powers (). In the same way, the highest yield for N-aryl pyrrole as product was resulted from the reaction run under ultrasonic irradiation with a power of 65 W (, entry 2).
Table 1. Optimization of the catalyst amounts under ultrasonic irradiation (60 W) in watera.
Table 2. Optimization of ultrasonic power by using MWCNT-SO3H (0.02 g) in watera.
In continuation, the reaction of 2,5-dimethoxy tetrahydrofuran with 4-methoxy aniline (1.2:1 mol ratio) in the presence of MWCNT-SO3H (0.02 g) and power of 65 W was carried out in various solvents (). The best results were obtained using water as a solvent (, entry 4). It seems that ethanol was not a convenient solvent for this reaction due to the reasons such as the difference between dipole moment and polarity of ethanol and other used solvents for further solubilization of the reaction intermediates. Also, further ability of water for hydrogen bonding formation with reaction intermediates was compared with ethanol.
Table 3. Optimization of solvents in the presence of MWCNT-SO3H (0.02 g) under ultrasonic irradiation (65 W)a.
In order to ascertain the limitations of this protocol, the reaction of 2,5-dimethoxy tetrahydrofuran with several primary aromatic amines was carried out according to the general experimental procedure. The corresponding products and their results are summarized in . As can be seen from this table, the products were obtained in excellent yields and short reaction times for all of the entries except entry 10, 3j product, due to the steric hindrance of chlorine atom in the ortho situation of the amino group nucleophile that led to a reduction of the yield of reaction. Also, the usage of MWCNT-SO3H as a catalyst in the present work results in higher yields and efficiencies and lower times for the preparation of some N-substituted pyrroles compared with previously reported works using graphene oxide catalyst (Citation41) and catalyst-free (Citation42), in which the products were obtained in lower yields and longer reaction times (, entries 13–15). These results can be related to advantages of the catalyst role in this reaction.
Table 4. Synthesis of various N-aryl pyrrole using the catalytic amount of MWCNT-SO3H as catalyst under ultrasonic irradiation in watera.
In order to investigate the effects of ultrasonic irradiation and to evaluate and compare conventional heating with the ultrasound-assisted method, we continued our efforts under different conditions. The results are listed in . The ultrasound could increase the rate of reaction and therefore reduce the energy consumption. The chemical and physical effects of ultrasound are primarily from sonic cavitation, which includes formation, growth and collapse of the hole. The drive force behind the increased efficiency of N-aryl pyrroles formation by ultrasound is due to the increase in the temperature due to the formation of hot spots; and due to increase in the reactant impact surface area through the cavitations’ event.
Table 5. Reaction of 2,5-dimethoxy tetrahydrofuran with several amines in different conditionsa.
In this study, N-aryl pyrroles as products of an efficient method were prepared through the reaction of 2,5-dimethoxy tetrahydrofuran and various primary aromatic amines in the presence of heterogeneous catalytic amount of MWCNT-SO3H (0.02 g) under ultrasonic irradiation and water as a convenient solvent.
For practical applications of heterogeneous catalysts, the life-time of the MWCNTs-SO3H and its level of reusability are very important features. At the end of each reaction, the catalyst was isolated by filtration, washed exhaustively with chloroform and ethanol and dried at 100°C for 24 h before being used with fresh materials. The catalyst was reused for four runs; the yields were ranged from 95% to 90% ().
The structures of products and MWCNTs-SO3H were confirmed by spectroscopic and physical data such as: IR, 1H NMR, 13C NMR, XRD, SEM, BET, EDX, Raman and XPS spectroscopy. The 1H NMR spectra of 1-(3-nitrophenyl)-1H-pyrrole showed the signals around δ = 6.33–7.34 ppm that are assigned to protons of aromatic rings. The 13C NMR spectra of the above-mentioned compound showed the signals around δ = 110.0–150.0 ppm that are assigned to carbon atoms of the aromatic rings.
A plausible mechanism for the formation of N-aryl pyrroles is presented in Scheme 3. As can be seen, in the first step, the acetal group of 2,5-dimethoxy tetrahydrofuran compound was protonated (a). Then, the nucleophilic attack of the hydroxyl group of the substrate to the carbon atom of acetal produces the intermediate (b). Next, b was protonated by MWCNTs-SO3H and dehydrated and converted to carbonyl group d, then, d was protonated by MWCNTs-SO3H, and the nucleophilic attack of the amino group to carbonyl e occurs. The f was protonated, cyclized and dehydrated to give N-aryl pyrrole as a desired product.
Experimental
Materials and apparatus
All commercially available reagents were used without further purification and purchased from Merck Chemical Company in high purity. The used solvents were purified by standard procedures. IR spectra were obtained as KBr pellets on a Perkin-Elmer 781 spectrophotometer and on an impact 400 Nicolet FTIR spectrophotometer. 1H NMR and 13C NMR were recorded in CDCl3 and DMSO-d6 solvents on a Bruker DRX-400 spectrometer with tetramethylsilane as the internal reference. UV-Vis spectra were recorded with a Perkin-Elmer 550 spectrophotometer in CH2Cl2 solvents. The nanostructures were characterized using a Holland Philips Xpert XRD diffractometer (CuK, radiation, k = 0.154056 nm), at a scanning speed of 2°/min from 10° to 100° (2Ø). The morphological study of nanocomposites was investigated by a SEM (Leo 1455VP). EDX of catalyst was performed on a Philips Holland model XL30. The surface area analysis (BET) was performed by using an automated gas adsorption analyzer (NANO SORD). The Raman spectra were recorded with an Almega Thermo Nicolet Dispersive Raman spectrometer excited at 532 nm. XPS spectra were measured on an ESCA-3000 electron spectrometer with non-monochromatized Mg Kα X-rays as the excitation source. Melting points obtained with a Yanagimoto micro melting point apparatus are uncorrected. The purity determination of the substrates and reaction monitoring were accomplished by TLC on silica-gel polygram SILG/UV 254 plates (from Merck Company).
General procedure for the synthesis of MWCNTs-SO3H
MWCNTs (1 g) and deionized water (100 ml) were added to a beaker and was sonicated for 30 min. After the sonication, the solvent was removed in vacuum and the obtained MWCNTs were transferred to another flask including 25 ml HNO3 (63%); 25 ml HCl (37%) and stirred at 80°C for 4 h under a nitrogen atmosphere. Then the solution was filtered in vacuum and washed throughly with deionized water and then dried at 100°C for 10 h. In this step, the MWCNTs-COOH was obtained. The MWCNTs-COOH (1 g) and deionized water (50 ml) were sonicated for 15 min. Then, the water was filtered and MWCNTs-COOH was dried. Then 40 ml of H2SO4 (98%) was added to the setup at 250–270°C for 20 h under a nitrogen atmosphere. After cooling the solution, the liquid was filtered and washed throughout with deionized water several times. The obtained solid was dried at 100°C for 12 h. After this step, the MWCNTs-SO3H was obtained and used in the synthesis of N-aryl pyrroles.
General procedure for the synthesis of N-aryl pyrrole
To a mixture of 2,5-dimethoxy tetrahydrofuran (1.2 mmol) and different primary aromatic amines (1 mmol) in water, MWCNT-SO3H (0.02 g) was added and reacted under ultrasonic irradiation (65 W). The progress of the reactions was monitored by TLC (ethyl acetate/n-hexane 6/4). The catalyst along with the solid product was collected by filtration from the reaction mixture. The filtrated solid was dissolved in chloroform, the catalyst separated from the reaction mixture and washed with chloroform. The solvent was removed in vacuum to give the crude product. Then, the crude product was recrystallized from ethanol, N-aryl pyrroles were obtained as pure products. The recovered catalyst (0.019 g) was reused in the reaction for four runs. All of the pure products were characterized by comparison of their physical and spectral data with authentic samples (Citation43–53).
1-Phenyl-1H-pyrrole(3a); brown solid; m.p. = 55–57°C, (m.p. = 56–58°C) (Citation43); UV-Vis (CH2Cl2) λmax: 310; IR (KBr) ν(cm−1): 3033 (C–H, sp2 stretch), 1497, 1597 (C9C Ar), 746, 692 (C–H, sp2 OOP); 1H NMR (400 MHz, CDCl3) δ (ppm): 6.37–6.38 (d, 2H, J 4.0 Hz, 2CH), 7.06–7.07 (d, 2H, J 4.0 Hz, 2CH), 7.33–7.41 (m, 5H, 5CH).
1-p-Tolyl-1H-pyrrole(3b); black solid; m.p. = 79–81°C, (m.p. = 80–82°C) (Citation44); UV-Vis (CH2Cl2) λmax: 315; IR (KBr) ν(Cm−1): 3050 (C–H, sp2 stretch), 2922 (C–H, sp3), 1509, 1616 (C9C, Ar); 1H NMR(CDCl3, 400 MHz) δ (ppm): 2.38 (s, 3H, CH3), 6.34 (s, 2H, 2CH), 7.01 (s, 2H, 2CH), 7.23 (d, 2H, J 8.0 Hz, 2CH), 7.27–7.29 (d, 2H, J 8.0 Hz, 2CH).
1-(4-Methoxy phenyl)-1H-pyrrole(3c); black solid, m.p. = 87–89°C, (m.p. = 88–89°C) (Citation45); UV-Vis (CH2Cl2) λmax; 325; IR (KBr) ν(cm−1): 3033(C–H, sp2 stretch), 2926 (C–H, sp3), 1597(C9C, Ar), 1310 (C–O), 749 (C–H sp2 OOP); 1H NMR (CDCl3, 400 MHz) δ (ppm): 3.85 (s, 3H, CH3), 6.33 (s, 2H, 2CH), 6.95–6.97 (d, 2H, J 8.4 Hz, 2CH), 7.01 (s, 2H, 2CH), 7.31–7.34 (d, 2H, J 8.4 Hz, 2CH).
1-(3-Methoxy phenyl)-1H-pyrrole(3d); yellow solid; m.p. = 46–48°C, (b.p. = 98–100°C) (Citation46); UV-Vis (CH2Cl2) λmax; 320; IR (KBr) ν (cm−1): 3000 (C–H, sp2), 2850 (C–H sp3), 1400–1600 (C9C, Ar), 1300 (C–O), 743 (C–H sp2 OOP); 1H NMR(CDCl3, 400 MHz) δ (ppm): 3.86 (s, 3H, CH3), 6.36 (s, 2H, 2CH), 6.80–6.82 (d, 1H, J 8.0 Hz, CH), 6.95 (s, 1H, CH), 7.00–7.02 (d, 1H, J 8.0 Hz, CH), 7.11 (s, 2H, 2CH), 7.32–7.36 (t, 1H, J 8.4 Hz, CH).
1-(4-Hydroxy phenyl)-1H-pyrrole(3e); brown solid, m.p. = 114–116°C, (m.p. = 113–115°C) (Citation47); UV-Vis (CH2Cl2) λmax; 325; IR (KBr) ν (cm−1): 3448 (O-H, stretch), 3135 (C–H, sp2), 1519 (C9C, Ar), 1024 (C–O, stretch), 732 (C–H sp2 OOP); 1H NMR (DMSO-d6, 400 MHz) δ (ppm): 6.19 (s, 2H, 2CH), 6.52 (s, 1H, OH), 6.80–6.82 (d, 2H, J 8.8 Hz, 2CH), 7.16 (s, 2H, 2CH), 7.31–7.33 (d, 2H, J 8.4 Hz, 2CH).
1-(Naphthyl)-1H-pyrrole(3f); brown solid; m.p. = 116–118°C, (m.p. = 120°C) (Citation48); UV-Vis (CH2Cl2) λmax; 356; IR (KBr) ν(cm−1): 3054 (C–H, sp2 stretch), 1596, 1485 (C9C, Ar), 728 (C–H sp2 OOP); 1H NMR(CDCl3, 400 MHz) δ (ppm): 6.46 (s, 2H, 2CH), 7.04 (s, 2H, 2CH), 7.51–7.57 (q, 4H, J 6.8 Hz, 4CH), 7.79–7.81 (d, 1H, J 7.2 Hz, CH), 7.90–8.00 (q, 2H, J 7.6 Hz, 2CH).
1-(4-Chloro phenyl)-1H-pyrrole(3g); yellow solid; m.p. = 80–82°C, (m.p. = 81–83°C) (Citation49); UV-Vis (CH2Cl2) λmax; 312; IR (KBr) ν(cm−1): 3102 (C–H, sp2 stretch), 1501(C9C, Ar), 728 (C–Cl); 1H NMR(CDCl3, 400 MHz) δ (ppm): 6.36–6.37 (d, 2H, J 2.0 Hz, 2CH), 7.06 (d, 2H, J 1.6 Hz, 2CH), 7.32–7.34 (d, 2H, J 8.8 Hz, 2CH), 7.39–7.41 (d, 2H, J 8.8 Hz, 2CH).
1-(4-Bromo phenyl)-1H-pyrrole(3h); yellow solid; m.p. = 91–93°C, (m.p. = 93–94.5°C) (Citation50); UV-Vis (CH2Cl2) λmax; 315; IR (KBr) ν(cm−1): 3130 (C–H, sp2), 1497, 1590 (C9C, Ar), 727(C–H sp2 OOP), 512 (C–Br); 1H NMR(CDCl3, 400 MHz) δ (ppm): 6.36–6.37 (d, 2H, J 2.0 Hz, 2CH), 7.06 (d, 2H, J 2.0 Hz, 2CH), 7.27–7.29 (d, 2H, J 9.2 Hz, 2CH), 7.53–7.56 (d, 2H, J 8.8 Hz, 2CH); 13C NMR (CDCl3, 100 MHz) δ(ppm):110.85, 119.28, 121.62, 129.64, 131.04, 139.33.
1-(3-Chloro phenyl)-1H-pyrrole(3i); brown solid; m.p. = 51–53°C, (m.p. = 51–52°C) (Citation51); UV-Vis (CH2Cl2) λmax; 311; IR (KBr) ν(cm−1): 3102 (C–H, sp2 stretch), 1500 (C9C, Ar), 720–820 (C–Cl); 1H NMR (CDCl3, 400 MHz) δ (ppm): 6.36 (s, 2H, 2CH), 7.08 (s, 2H, 2CH), 7.21–7.23 (d, 1H, J 7.6 Hz, CH), 7.28–7.30 (d, 1H, J 8.4 Hz, CH), 7.34–7.38 (t, 1H, J 8.0 Hz, CH), 7.40 (s, 1H, CH).
1-(2-Chloro phenyl)-1H-pyrrole(3j); black oil; UV-Vis (CH2Cl2) λmax; 310; IR (KBr) ν(cm−1): 3050 (C–H, sp2 stretch), 1500 (C9C, Ar), 720–820 (C–Cl); 1H NMR(CDCl3, 400 MHz) δ (ppm): 6.43–6.44 (d, 2H, J 4.0 Hz, 2CH), 7.18–7.19 (d, 2H, J 4.0 Hz, 2CH), 7.61–7.65 (t, 1H, J 8.0 Hz, CH), 7.74–7.76 (t, 1H, J 4.0 Hz, CH), 8.11–8.13 (d, 2H, J 8.0 Hz, 2CH).
1-(4-Nitro phenyl)-1H-pyrrole(3k); yellow solid; m.p. = 179–181°C, (m.p. = 180–183°C) (Citation52); UV-Vis (CH2Cl2) λmax; 335; IR (KBr) ν(cm−1): 3150 (C–H, sp2), 1616 (C9C, Ar), 1527 (N=O), 1347 (C–N); 1H NMR(CDCl3, 400 MHz) δ (ppm): 6.44 (s, 2H, 2CH), 7.20 (s, 2H, 2CH), 7.52–7.55 (d, 2H, J 8.8 Hz, 2CH), 8.32–8.34 (d, 2H, J 9.2 Hz, 2CH).
1-(3-Nitro phenyl)-1H-pyrrole(3l); yellow solid; m.p. = 67–69°C, (m.p. = 66–68°C) (Citation53); UV-Vis (CH2Cl2) λmax; 332; IR (KBr) ν(cm−1): 3150 (C–H, sp2), 1616 (C9C, Ar), 1527 (N=O), 1347 (CN); 1H NMR (CDCl3, 400 MHz) δ (ppm): 6.42–6.43 (t, 2H, J 2.0 Hz, 2CH), 7.17–7.18 (t, 2H, J 2.4 Hz, 2CH), 7.60–7.64 (t, 1H, J 8.4 Hz, CH), 7.73–7.75 (d, 1H, J 7.6 Hz, CH), 8.10–8.12 (d, 1H, J 8.0 Hz, CH), 8.26–8.28 (t, 1H, J 2.0 Hz, CH); 13C NMR (CDCl3, 100 MHz) δ (ppm): 111.84, 114.33, 119.17, 119.83, 125.65, 130.92, 141.31, 149.00.
Conclusion
In this research, we described the synthesis of N-aryl pyrroles using 2,5-dimethoxy tetrahydrofuran with different primary aromatic amines. This reaction was carried out in the presence of catalytic amount of MWCNT-SO3H under ultrasonic irradiations. The corresponding products were obtained in excellent yields, high purity and short reaction times. The products were confirmed by physical and spectroscopic data such as: IR, 1H NMR, 13C NMR spectroscopy.
Supplement_Material.docx
Download MS Word (2 MB)Disclosure statement
No potential conflict of interest was reported by the authors.
Notes on contributors
Hossein Naeimi received his M.Sc. degree in 1991 from Shahid Beheshti University and Ph.D. degree in 1998 from Shiraz University. After working at Government College, he became Assistant Professor at University of Kashan and subsequently became Associate Professor in 2004 and Professor in 2008. His research interests include synthesis of Schiff bases, heterocycles and anthraquinones.
Mahla Dadaei received her B.Sc. degree in Chemistry from the University of Kashan, in 2003 and then her M.Sc. degree in organic chemistry from the same University in 2013. Now, she is Ph.D student in organic chemistry in the same University.
Additional information
Funding
References
- Regina, G.L.; Silvestri, R.; Artico, M.; Lavechia, A.; Novellino, E.; Befani, O.; Turini, P.; Agostinelli, E.J. Med. Chem. 2007, 50, 922–931. doi: 10.1021/jm060882y
- Jones, R.A.; Bean, G.P. Academic Press: London1977, 1.
- Sundberg, R.J. In Comprehensive Heterocyclic Chemistry; Katritzky, A.R., Rees, C., Eds.; Pergamon Press: Oxford, 1984. Vol. 4, p 370.
- Sundberg, R.J. In Comprehensive Heterocyclic Chemistry II; Katritzky, A.R., Rees, C., Scriven, E.F.V., Eds.; Pergamon Press: Oxford, 1996. Vol. 2, p 149.
- Boger, D.L.; Boyce, C.W.; Labroli, M.A.; Sehon, C.A.; Jin, Q.;Total Syntheses of Ningalin A, Lamellarin, O.; Lukianol, A.; Permethyl, S.A.J. Am. Chem Soc. 1999, 121, 54–62. doi: 10.1021/ja982078+
- Gupton, T. J. Heterocycl. Chem. 2006, 2, 53–92.
- Fan, H.; Peng, J.; Hamann, M.T.; Hu, J.F. Chem. Rev. 2008, 108, 264–287. doi: 10.1021/cr078199m
- Finar, I.L. Longman, London. 1975, 2, 885–913.
- Ruault, P.; Pilard, J.F.; Touaux, B.; Boullet, F.T.; Hamelin, J. Synlett. 1994,935–936. doi: 10.1055/s-1994-23054
- Danks, T.N. Tetrahedron Lett. 1999, 40, 3957–3960. doi: 10.1016/S0040-4039(99)00620-6
- Furstner, A.; Weintritt, H.; Hupperts, A. J. Org. Chem. 1995, 60, 6637–6641. doi: 10.1021/jo00125a068
- Katritzky, A.; Jiang, J.; Steel, P.J. J. Org. Chem. 1994, 59, 4551–4555. doi: 10.1021/jo00095a034
- Rivera, S.; Bandyopadhyay, D.; Banik, B.K. Tetrahedron Lett. 2009, 50, 5445–5448. doi: 10.1016/j.tetlet.2009.06.002
- Bandyopadhyay, D.; Mukherjee, S.; Banik, B.K. Molecules 2010, 15, 2520–2525. doi: 10.3390/molecules15042520
- Kumar, M.A.; Krishna, A.B.; Babu, B.H.; Reddy, C.B.; Reddy, C.S. Synth. Commun. 2008, 38, 3456–3464. doi: 10.1080/00397910802157843
- Mahmoudi, H.; Jafari, A.A. ChemCatChem. 2013, 5, 3743–3749. doi: 10.1002/cctc.201300623
- Jafari, A.A.; Mahmoudi, H.; Mirjalili, B.F. J. Iran. Chem. Soc. 2011, 8, 851–856. doi: 10.1007/BF03245915
- Polshettiwar, V.; Baruwati, B.; Varma, R.S. Chem. Commun. 2009, 46, 1837. doi: 10.1039/b900784a
- Polshettiwar, V.; Varma, R.S. Tetrahedron. 2010, 66, 1091–1097. doi: 10.1016/j.tet.2009.11.015
- Ma, F.P.; Li, P.H.; Li, B.L.; Mo, L.P.; Liu, N.; Kang, H.J.; Liu, Y.N.; Zhang, Z.H. Appl. Catal A. 2013, 457, 34–41. doi: 10.1016/j.apcata.2013.03.005
- Bandyopadhyay, D.; Mukherjee, S.; Granados, J.C.; Short, J.D.; Banik, B.K. Eur. J. Med. Chem. 2012, 50, 209–215. doi: 10.1016/j.ejmech.2012.01.055
- Serp, P.; Corrias, M.; Kakk, P. Appl. Catal A. 2003, 253, 337–358. doi: 10.1016/S0926-860X(03)00549-0
- Zhang, W.; Zhang, Z.; Zhang, Y. Nanoscale. Res. Lett. 2011, 6, 555. doi: 10.1186/1556-276X-6-555
- Derycke, V.; Aurray, S.; Borghetti, J. Physicue 2009, 10, 330.
- Xing, Y.C.; Li, L.; Chusuei, C.C.; Hull, R.V. Langmuir 2005, 21, 4185–4190. doi: 10.1021/la047268e
- Naeimi, H.; Mohajeri, A.; Moradi, L.; Rashidi, A.M. Appl. Surf. Sci. 2009, 256, 631–635. doi: 10.1016/j.apsusc.2009.08.094
- Bougrin, K.; Loupy, A.; Petit, A.; Benhida, R.; Fourrey, J.L.; Daou, B.; Soufiaoui M. Tetrahedron Lett. 2000, 41, 4875–4879. doi: 10.1016/S0040-4039(00)00723-1
- Ambulgekar, G.V.; Samant, S.D.; Pandit, A.B. Ultrason Sonochem. 2005, 12, 85–90. doi: 10.1016/j.ultsonch.2004.04.005
- Suslick, K.S. Sonochemistry Science 1990, 247, 1439.
- Mason, T. J. Chem. Soc. Rev. 1997, 26, 434.
- Cravotto, G.; Cintas, P. Chem. Soc. Rev. 2006, 35, 180–196. doi: 10.1039/B503848K
- Naeimi, H.; Rabiei, Kh. Ultrason. Sonochem. 2012, 19, 130–135. doi: 10.1016/j.ultsonch.2011.06.012
- Ghahremanzadeh, R.; Rashid, Z.; Zarnani, A.H.; Naeimi, H. Ultrason. Sonochem. 2014, 21, 1451–1460. doi: 10.1016/j.ultsonch.2014.02.014
- Naeimi, H.; Rabiei, Kh. Bull. Chem. Soc. JPn 2011, 84, 1112–1117. doi: 10.1246/bcsj.20110106
- Rabiei, Kh.; Naeimi, H. Ultrason. Sonochem. 2015, 24, 150–154. doi: 10.1016/j.ultsonch.2014.12.011
- Naeimi, H.; Foroughi, H. Chin. J. Catal. 2015, 36, 734–741. doi: 10.1016/S1872-2067(14)60304-1
- Osorio, A.G.; Bueno, I.C.L.; Bergmann, C.P. Appl. Surf. Sci. 2008, 255, 2485–2489. doi: 10.1016/j.apsusc.2008.07.144
- Peng, H.; Alemany, L.B.; Margrave, J.L.; Khabishesku, V.N. J. Am. Chem. Soc. 2003, 125, 15174–15182. doi: 10.1021/ja037746s
- Ago, H.; Kugler, T.; Cacialli, F.; Salaneck, W.R.; Shaffer, M.S.P.; Windle, A.H.; Friend, R.H. J. Phys. Chem B. 1999, 103, 8116–8121. doi: 10.1021/jp991659y
- Duesberg, G.S.; Graupner, R.; Downes, P.; Minett, A.; Ley, L.; Roth, S.; Nicoloso, N. Synth. Met. 2004, 142, 263–266. doi: 10.1016/j.synthmet.2003.09.009
- Chen, C.-Y.; Guo, X.-Y.; Lu, G.-Q.; Pedersen, C.-M.; Qiao, Y.; Hou, X.-L.; Wang, Y.-X. New Carbon Mat. 2017, 32(2), 160–167. doi: 10.1016/S1872-5805(17)60013-6
- Cho, H.; Madden, R.; Nisanci, B.; Torok, B. Green Chem. 2015, 17, 1088–1099. doi: 10.1039/C4GC01523A
- Miles, K.C.; Mays, S.M.; Southerland, B.K.; Auvil, T.J.; Ketcha, D.M. Arkivoc 2009, xiv, 181–190.
- Chang, K.L.; Jung, H.J.; Ji, S.Y. J. Hetero. Chem. 2000, 37, 15–24. doi: 10.1002/jhet.5570370104
- Haitao, Y.; Chao, X.; Zhiwei, M.; Ruyu, C. Eur. J. Org. Chem. 2011, 18, 3353–3360.
- Ferenc, F.; Katalin, F.; Angelika, T.; Laszlo, T. Tetrahedron 1997, 53, 4883–4888. doi: 10.1016/S0040-4020(97)00183-X
- Chao-Wu, Y.; Chen-Wei, H.; Ji-Wang, C.; Grace, C. Org. Lett. 2012, 14, 3688–3691. doi: 10.1021/ol301523q
- Katla, R.; Narayana, M.S.; Durga, N.Y.V. Synth. Commun. 2012, 42, 2471–2477. doi: 10.1080/00397911.2011.560744
- Rui, Z.; Lixin, X.; Xinyan, W.; Chuanjie, C.; Deyong, S.; Yuefei, H. Adv. Synth. Catal. 2008, 350, 1253–1257. doi: 10.1002/adsc.200700535
- Weiqiang, C.; Jianhui, W. Organometallics 2013, 32, 1958–1963. doi: 10.1021/om400046r
- Hui-Jun, K.; Bao-Le, L.; Pei-He, L.; Ning, L.; Ya-Nan, L.; Fei-Ping, M.; Li-Ping, M.; Zhan- Hui, Z. Appl. Catal. 2013, 457, 34–41. doi: 10.1016/j.apcata.2013.03.005
- Prakash, R.V.; Vijay, K.A.; Rama, R.K. Tetrahedron Lett. 2011, 52, 777–780. doi: 10.1016/j.tetlet.2010.12.016
- Corelli, F.; Massa, S.; Stefancich, G.; Artico, M.; Panico, S.; Simonetti, N. Farm. Ed. Scient. 1984, 39, 95–109.