ABSTRACT
The behavior of the gorse extract has been investigated as a new corrosion inhibitor by weight-loss measurements, potentiodynamic polarization curves, electrochemical impedance spectroscopy and scanning electron microscopy. Weight-loss measurements showed an increase in inhibition efficiency (IE) with the immersion time and the concentration of the extract. At 800 mg L−1, the extract IE ranged from 72.5% to 93.8% for 2 and 48 h, respectively. The Ea decreased slightly with the addition of the extract, characterizing the chemical adsorption by the molecules present in the extract on the surface. The measurements of electrochemical impedance spectroscopy showed an increase in Rct and a decrease in Cdl, reaching 96.6% of IE at 1600 mg L−1. The polarization curves indicated that the gorse extract served as an adsorption inhibitor, reducing both the anodic and cathodic current density. The high-molecular-weight fraction isolated from the gorse extract also showed high inhibition efficiencies.
GRAPHICAL ABSTRACT
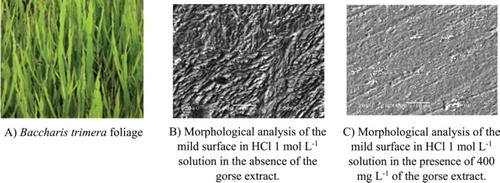
Highlights
The IE remains quite constant with the temperature with a slight increase.
The polarization curves indicate that the extract acts as a mixed-type inhibitor.
The electrochemical measurements showed that the inhibitor is a blocking type.
Adsorption of the extract followed a Langmuir adsorption isotherm.
Introduction
To reduce the high cost generated by corrosion, corrosion inhibitors have been widely used by many industries as one of the best methods to protect against deterioration of materials. In addition, many research groups seek the use of new compounds for this purpose ( Citation1). Although the use of corrosion inhibitors is one of the best methods to prevent corrosion, it is important to check the properties and mechanisms of action of these substances to avoid harmful effects to the environment, since many products make up a mixture of compounds organic ( Citation1). These inhibitory molecules consist of heterocyclic compounds with polar functional groups (e.g. N, S, O and P) and double bonds conjugated with different aromatic systems. These compounds are adsorbed on the metal surface to hinder the destruction reaction with aggressive means. They are both physically and chemically active adsorbate-type substances ( Citation2).
Most of the inhibitors currently used are synthetic, expensive and not always friendly to the environment. Consequently, there is a need to develop a new class of ecological corrosion inhibitor with low toxicity and good inhibition efficiency. Therefore, many researchers direct their research to the study of natural extracts as corrosion inhibitors for different metals and corrosive media ( Citation1). Research related to inhibitors of natural sources such as leaves, stems, fruits, plant seeds and food residues have shown a high efficiency of inhibition of corrosion for different metals and corrosive media ( Citation1–31). gives a comparison of the inhibition efficiency (IE%) of different extracts from natural sources. Good inhibition efficiencies can be achieved, reaching 98% for corrosion of carbon steel in HCl solution using the garlic peel extract.
Table 1. Percentage of corrosion inhibition efficiency for some extracts of natural products.
It is extremely important that the research of new formulations of corrosion inhibitors meet new industrial objectives without prejudice to society. From this point of view, the development of green corrosion inhibitors is a very promising future. Aqueous plant extracts are viewed as a source of naturally occurring biodegradable organic compounds and can be extracted by simple procedures resulting in high efficiencies ( Citation6).
The gorse (Baccharis trimera (Less.) DC) is a plant of the genus Baccharis represented by more than 400 species ( Citation32). Recent studies have shown antisecretory, anti-inflammatory and antioxidant properties, attributed to the presence of diterpene compounds such as phytochemicals, flavonoids and phenolic acids ( Citation33). According to Pocá ( Citation34), the anti-inflammatory, antiulcer and analgesic activities are attributed mainly to the presence of saponins and tannins present in their composition.
The objective of the present work is to investigate the inhibitory action of aqueous gorse extract on mild steel corrosion in 1 mol L−1 HCl medium by weight-loss measurements and electrochemical techniques, as well as through surface analysis using scanning electron microscopy (SEM).
Experimental
Obtaining the extract
Leaves of the gorse herbs, B. trimera (Less.) DC; Asteraceae, from the brand Estrella da Terra, were used as samples for this study.
Sixty grams of the gorse leaves were placed in 600 mL of double boiled distilled water for infusion for 1 h. Filtration was then performed and the filtrate was stored in glass containers and placed in a freezer at −4°C.
The containers containing the frozen filtrate were placed in a lyophilizer of the Liotop brand; model L101 at an average temperature of −52°C, so that the entire water content of the sample was removed without its modified structure, generating a powder as a final product.
Obtaining the high-molecular-weight fraction (HMWF)
Isolation of the HMWF was performed using an ultrafiltration method. A 100-mL aliquot of the aqueous gorse extract was added to a 3-kDa cut-off ultrafiltration apparatus (Millipore, USA) which was immediately centrifuged. The fraction retained by the membrane was washed three times with water and then lyophilized and stored at −4°C prior to analysis. This fraction was also studied by weight-loss measurements during 24 h of immersion time and by electrochemical impedance in 1 mol L−1 HCl.
Weight-loss measurements
Samples of mild steel with the following composition (% by weight): C: 0.18, P: 0.04, S: 0.05, Mn: 0.30, Si: trace and Fe: balance were abraded with 100 grade emery paper, sandblasted, washed with doubly distilled water, degreased with acetone and air-dried. Weight loss was determined by gravimetric testing using an analytical balance with an accuracy of 0.1 mg.
In variable time tests, three mild steel coupons were placed in glass containers so that the entire surface was in contact with a 1 mol L−1 HCl solution prepared from 37% HCl purchased from Merck Co. (Darmstadt, Germany) and doubly distilled water. The solutions in the absence and presence of inhibitor at concentrations ranging from 100 to 800 mg L−1 were dropped into containers and left at intervals of 2, 24, and 48 h. After the elapsed time, the coupons were removed from the solution, rinsed with doubly distilled water and acetone, dried and weighed again.
Each assay containing three coupons was performed in duplicates and the inhibition efficiency was obtained by averaging the two assays. The inhibition efficiency (IE%) was calculated from (
Citation6):(1) where Wcorr and Wcorr,0 are the corrosion rates of the mild steel in 1 mol L−1 HCl, in the presence and absence of the extract, respectively, in g cm−2 h−1.
The tests with temperature variation were carried out in the temperature range of 25–55 °C for 2 h in the presence and absence of 200 mg L−1 of the gorse extract. For this experiment, we used two jacketed containers, in which circulated water from a coupled thermostat bath, where the temperature was controlled. After the elapsed time, the coupons were removed from the solution, rinsed with doubly distilled water and acetone, dried and weighed again. These tests were also carried out, each with three coupons in duplicate, where inhibition efficiency was obtained by averaging the efficiencies calculated from the two tests.
Electrochemical essays
Mild steel coupons were abraded using the AROPOL 2V (AROTEC) polishing machine and water grit paper having a granulometry of 100, 320, 600, 1200 and 2000, washed with doubly distilled water and dried.
All electrochemical measurements were performed on a standard three-electrode Pyrex cell which was composed of a mild steel coupon as a working electrode, a saturated calomel electrode (Hg/Hg2Cl2/Cl−) as the reference electrode and a platinum wire as a counter electrode.
The electrochemical measurements were performed in a model AUTOLAB-PGSTAT128N potentiostat/galvanostat with a Metrohm impedance module and the software used was FRA (frequency response analyzer) for measurements of electrochemical impedance and general-purpose electrochemical system to obtain the curves polarization and the open-circuit potential (OCP).
OCP measurements
For all electrochemical analyzer, the OCP was monitored for 4000 s until it became constant.
Electrochemical polarization
Anodic and cathodic polarization curves were obtained in the presence and absence of the extract, varying the potential from −300 to +300 mV with respect to the OCP at a speed of 1 mV s−1.
After obtaining the polarization curves, the Tafel extrapolation method was used, where the corrosion current densities, jcorr, were obtained and IE% values were calculated according to the following equation (
Citation6):(2) where jcorr,0 and jcorr are the corrosion current densities of mild steel in 1 mol L−1 HCl medium in the absence and presence of the inhibitor, respectively.
Electrochemical impedance spectroscopy
This test was performed using the FRA software where impedance measurements were made by applying 10 mV (rms) as a disturbance in a frequency range of 100 kHz to 10 mHz, with the working electrode at the stable OCP.
Using this technique, some electrochemical parameters were determined as the charge transfer resistance (Rct). With this assigned value, it is possible to calculate the degree of surface coverage (θ) and the inhibition efficiency, IE%, given by the following equations (
Citation6):(3)
(4) where Rct,0 and Rct are the mild steel charge transfer resistances in 1 mol L−1 HCl medium in the absence and presence of the inhibitor, respectively.
Scanning electron microscopy (SEM)
In order to analyze the morphology of the mild steel surface, two coupons were mechanically abraded with emery paper of 100–2000 meshes, as described in the electrochemical tests. After abrasion, the specimens were washed with doubly distilled water, degreased with acetone and finally dried.
Each coupon was immersed in a 1 mol L−1 HCl solution for 2 h, in the absence and presence of 400 mg L−1 of the gorse extract; after that time, they were removed from the solution and washed with doubly distilled water, degreased with acetone and dried.
After this procedure, the coupons were analyzed on a scanning electron microscope, JEOL JSM 6460LV with an acceleration voltage of 20 kV. The image was increased 2000×.
Total phenolic content
The quantification of total phenols was performed using the methodology used by Singleton et al. ( Citation35), by reacting the sample with the Folin reagent and the gallic acid calibration curves.
In all, 200 μL of the standard solutions of gallic acid (from 5 to 70 mg L−1) and the sample were added to test tubes, where 1400 μL of Milli-Q water and 100 μL of reagent of Folin were also added. The tubes containing the solutions and samples were vortexed and then waited for a minimum period of 30 s and a maximum of 8 min, to which were added 300 μL of a 20% Na2CO3 solution (m/v). The tubes were taken to a 40°C water bath for 30 min.
Using a spectrophotometer, model Lambda XLS +, brand Perkin Elmer, measurements of the absorbance of the standard solutions and of the sample containing the extract (to be diluted 10 times) were taken at a wavelength of 765 nm.
Results and discussion
Weight-loss measurements
The results of the gravimetric tests for mild steel varying the time and concentration of the extract are shown in .
Table 2. Results of gravimetric tests for mild steel by varying the time and concentration of the extract.
The stability of the inhibitory behavior of this new corrosion inhibitor was investigated by weight-loss measurements in 1 mol L−1 HCl for different immersion times. The corrosion rate (Wcorr) decreases as the time increases, especially in the presence of the extract. Consequently, the inhibition efficiency increases with the immersion time. The increase in IE over time reveals the stability of the molecules of the extract on the metallic surface. The increase in inhibition efficiency with increasing immersion time is due to the increase in the surface coverage with time. Moreover, these results show that the adsorption of the gorse extract on the mild steel is somewhat long and completed around 24 h ( Citation8). In addition, the inhibition efficiency increases with the concentration of the extract, resulting in an increase in the surface coverage. This result can be attributed to the increase in the number of molecules occupied by the inhibitor at the metal interface ( Citation19).
The extract of gorse presents an inhibition efficiency of 91.7% in 24 h and of 93.8% in 48 h of immersion in the presence of 800 mg L−1 of the extract.
shows the results obtained for weight-loss measurements performed during 2 h of total immersion of the mild steel, in the absence and presence of 200 mg L−1 of the gorse extract at temperatures ranging from 25°C to 55°C.
Table 3. Results of gravimetric tests in the presence and absence of 200 mg L−1 of the gorse extract for 2 h varying the time.
An increase in corrosion rates was observed both in the absence and in the presence of inhibitor as the temperature increases. The IE remains quite constant with the temperature with a slight increase. This result supposes that as the temperature of the solution increases, the water molecules desorption from the metal surface is more favored resulting in an increase in surface coverage.
(A) shows the Arrhenius plot for the mild steel in 1 mol L−1 HCl medium, in the absence and presence of 200 mg L−1 of the extract. Activation energies were calculated from the Arrhenius plots, Equation (5) (
Citation36), which represent the relation between ln Wcorr and the reciprocal of the absolute temperature.(5) where A is the frequency factor, Ea is the apparent activation energy, R is the constant for ideal gases and T is the absolute temperature.
Figure 1. Arrhenius plots: (A) ln Wcorr × 1/T and (B) ln(Wcorr/T) × 1/T for mild steel in 1 mol L−1 HCl in the absence and presence of gorse extract.
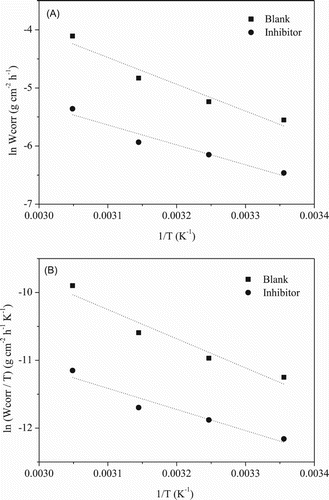
An alternative formulation of the Arrhenius equation is (
Citation36)(6) where h is the Planck constant, N is the Avogadro number, ΔS* is the entropy of activation and ΔH* is the enthalpy of activation. A graph of ln(Wcorr/T) vs. 1/T gave a line with a slope of −ΔH*/R and an intercept of ln(R/Nh) + ΔS*/R ((B)), whose values of ΔS* and ΔH* were calculated and are listed in . The data in reveal the values of the thermodynamic activation functions (Ea and ΔH*) for corrosion of the mild steel in 1 mol L−1 HCl in the absence and presence of the inhibitor. A somewhat lower value of Ea and ΔH* was observed in the presence of inhibitor. This phenomenon could be attributed to the inhibitor chemisorption involving the transfer of charge from the gorse extract molecules to the mild steel surface. This assumption is confirmed by the small increase in IE with increasing temperature. The positive signs of ΔH* obtained reveal the endothermic nature of the corrosion process (
Citation36).
Table 4. Apparent activation energy associated with the corrosion process in the absence and presence of the gorse extract.
The values of Ea are greater than the corresponding values of ΔH*, showing that the corrosion process must involve a gaseous reaction, merely the H2(g) evolution, allied with a decrease in the total reaction volume. Furthermore, the difference between Ea and ΔH* is 2.6 kJ mol−1, which is equal to the average value of RT (2.6 kJ mol−1) and indicates that the corrosion process is a unimolecular reaction ( Citation13). The activation entropy ΔS* was negative both in the absence and in the presence of the inhibitor, implying that the activated complex represented the rate determining step with respect to the association rather than the dissociation step. This infers that there was a decline in the disorder from the reagents to the activated complex ( Citation13).
Electrochemical results and discussion
Potentiodynamic polarization study
shows the anodic and cathodic curves for the mild steel in 1 mol L−1 HCl solution, obtained in the absence and presence of the gorse extract, in the concentrations shown below.
Figure 2. Polarization curves for the mild steel in 1 mol L−1 HCl in the absence and presence of different concentrations of gorse extract.
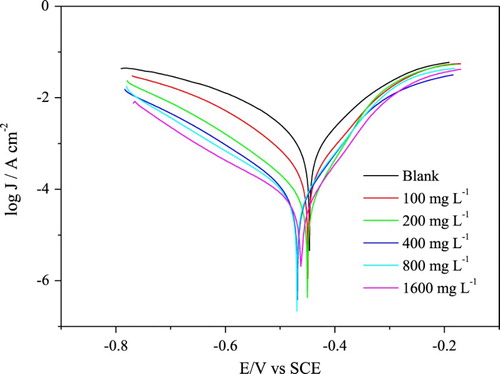
shows the electrochemical parameters such as corrosion potential (Ecorr), corrosion current density (jcorr) and anodic (βa) and cathodic (βc) Tafel constants obtained by the Tafel extrapolation method and the stabilized OCP.
Table 5. Electrochemical parameters obtained from polarization curves.
shows that both OCP and Ecorr, derived from the Tafel plots, show a slight cathodic shift with respect to the blank with a maximum displacement of 29 mV for Ecorr, demonstrating that the extract acts as a mixed-type inhibitor with a bit cathodic character.
As can be seen from , there were no significant changes in the values of the anodic and cathodic Tafel constants, for both the anodic (βa) and cathodic curves (βc), indicating that the inhibitor does not change the anodic and cathodic reactions, responsible for the dissolution of the metal and the reduction of , respectively. These results show that this inhibitor is a blocking type, acting by surface screening.
The presence of the extract decreased both the anodic and cathodic current densities decreasing the corrosion current density (jcorr) in the presence of the inhibitor relative to the blank test. From the 200 mg L−1 extract, the values of jcorr barely vary, and the same effect occurs with the inhibition efficiency while maintaining a value of 92%.
Electrochemical impedance spectroscopy
shows the electrochemical impedance diagrams obtained in the OCP for the mild steel in 1 mol L−1 HCl medium, in the absence and presence of different concentrations of extract. In the absence of the gorse extract, only one capacitive loop was observed in all frequency ranges which can be attributed to a single time constant. This loop is depressed and is often attributed to dispersion effects, which have been ascribed to surface roughness and inhomogeneities during corrosion ( Citation37).
Figure 3. Electrochemical impedance diagrams for mild steel in 1 mol L−1 HCl medium in the presence and absence of different concentrations of gorse extract.
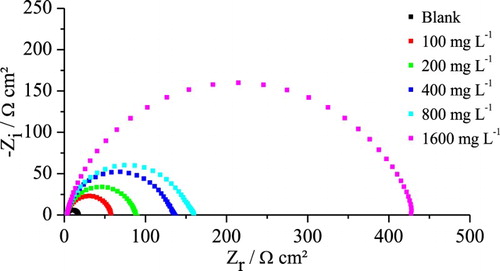
In addition, the electrochemical impedance diagrams obtained in the presence of the gorse extract present the same behavior, where a significant effect can be observed: the charge transfer resistance, Rct, whose value is inversely proportional to the corrosion rate ( Citation37), increases significantly with increasing extract concentration, reaching 96.6% EI at 1600 mg L−1. When comparing this result with the data given in , we can demonstrate that the gorse extract is a very effective inhibitor in the HCl solution, only remaining behind the garlic peel extract ( Citation19).
The electrochemical impedance diagrams were analyzed based on an equivalent circuit shown in , where Rs represents the ohmic resistance of the solution, Rct represents the resistance to charge transfer and CPE is the constant phase element, which was used instead of a double-layer capacitor to give a more precise adjustment. The CPE impedance is expressed by (
Citation37)(7) where Y0 is the magnitude of the CPE and n can take values between −1 and 1. When the capacitive loop presents a depressed semicircular appearance, n can take values between 0.5 and 1, which is often stated as a frequency dispersion as a result of inhomogeneity or roughness of the solid surface (
Citation37).
Figure 4. Equivalent circuit used to interpret the electrochemical impedance diagrams obtained for mild steel in the absence and presence of the extract.
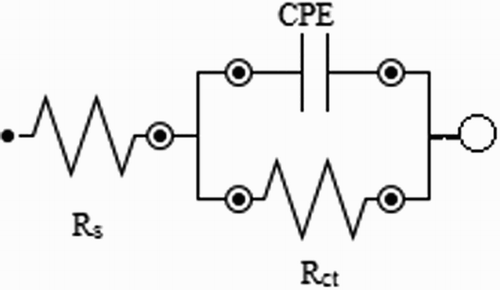
The double-layer capacitance, Cdl, for a circuit including a CPE was calculated from the following equation (
Citation37):(8) where ωmax = 2πfmax, and fmax is the frequency in which the imaginary component of the impedance is maximal.
shows the parameters obtained by adjusting the recorded EIS data using the equivalent circuit of . The increase in the extract concentration significantly decreases the frequency in which the imaginary component of the impedance is maximum (fmax) and increases the charge transfer resistance (Rct). These effects decrease the value of double-layer capacitance, which can be caused by the decrease in the local dielectric constant and/or by an increase in the thickness of the electrical double layer. These results show that the presence of the gorse extract changes the electrical double-layer structure, intending that the inhibitory molecules act by adsorption at the metal/solution interface.
Table 6. Electrochemical parameters obtained from the electrochemical impedance spectroscopy.
The adsorption of an organic adsorbate between the metal/solution interface can be considered as a replacement adsorption process between the organic molecules in the aqueous solution Org(soln) and the water molecules on the metal surface H2O(ads) (
Citation12, Citation31):(9) where Org(ads) are the organic molecules adsorbed on the metal surface, H2O(soln) is the water molecules in the aqueous solution and x is the size ratio representing the number of water molecules replaced by an organic adsorbate molecule.
From the electrochemical impedance data, it was possible to determine the degree of coverage of the metal surface (θ) as a function of inhibitor concentration. The experimental results were adjusted to different adsorption isotherms (Langmuir, Temkin, Flory–Huggins and El-Awady according to the following equations ( Citation12, Citation31, Citation38, Citation39):
Langmuir:(10) Temkin:
(11) Flory–Huggins:
(12) El-Awady:
(13) Langmuir’s theory admits that adsorption occurs at a specific and homogeneous site and each inhibitory molecule holds only one site (
Citation31).
The Temkin isotherm assumes that the heat of adsorption of all the molecules covering the adsorbent decreases linearly as a function of the coating due to the interactions between the adsorbed molecules (a > 0, attraction, <0, repulsion) ( Citation12, Citation31).
The Flory–Huggins isotherm believes that an active site may be occupied by more than one inhibitory molecule (parameter x). x greater than one unit indicates that more than one molecule of H2O(ads) was substituted by an organic molecule, i.e. x is the number of water molecules replaced by an inhibitory molecule ( Citation31).
Awady et al. ( Citation38) consider that a single inhibitory molecule can adsorb more than one active site (parameter y). y smaller than one unit shows that a single inhibitory molecule was adsorbed on more than one active site, i.e. y is the number of inhibitor molecules occupying an active site on the metal surface.
shows the parameters for the four different adsorption isotherms () obtained for the gorse extract as a corrosion inhibitor. According to Souza et al., in works of corrosion inhibitors, suitable correlation coefficients fall between 0.99 and 0.60 ( Citation40).
Figure 5. Langmuir (A), Temkin (B), Flory–Huggins (C) and El-Awady (D) isotherms for the adsorption of gorse extract on the mild steel surface in 1 mol L−1 HCl solution.
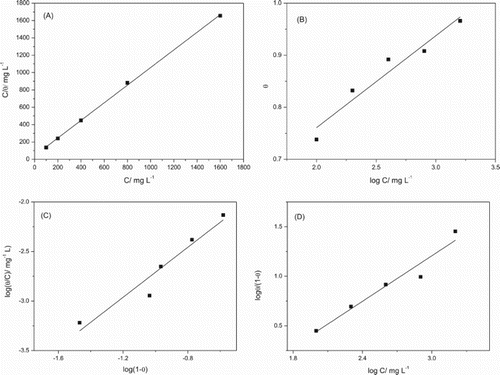
Table 7. Adsorption parameters obtained with gorse extract as corrosion inhibitor for mild steel in 1 mol L−1 HCl.
The Langmuir isotherm showed a better linear correlation coefficient, with a linear correlation coefficient of 0.9992 and an angular coefficient of 1.016. This behavior suggests that the molecules present in the gorse extract were adsorbed onto the mild steel surface according to a Langmuir adsorption isotherm, which accepts that the inhibition is produced by monolayer adsorption on the metal surface containing a fixed number of adsorption sites, and each site holds an adsorbate with no interaction between the adsorbate molecules. From the linear coefficient, the adsorption equilibrium constant (Kads) was calculated with a value of 0.024 L mg−1. As the angular coefficient is slightly greater than one, the existence of interactions between the adsorbed molecules and/or a number other than one of inhibitory molecules occupying an active site can be considered ( Citation31).
From the Temkin isotherm, it is possible to admit that there is a repulsive interaction between the inhibitory molecules adsorbed, once a < 0. It is also possible to consider that more than one molecule of H2O(ads) was substituted by an inhibitor molecule once for the Flory–Huggins x > 1 equation and for the El-Awady equation y < 0.
In summary, the angular coefficient obtained from the Langmuir isotherm makes it appear that the relationship between the active site and the adsorbed molecule is not a unit. This fact seems to be confirmed by the Flory–Huggins and El-Awady isotherms; a single inhibitory molecule is adsorbed on more than one active site where there was one molecule of water.
These results suggest the existence of a monolayer on the mild steel surface, which involves the chemical adsorption of bulky molecules on the metallic surface. Since the chemical nature of the adsorbed molecules is unknown, the determination of the thermodynamic parameters such as ΔGads is not possible ( Citation6, Citation19).
Mechanism
According to the literature, flavonoids and caffeoylquinic acids are found both in the infusions (our case) and in the hydroalcoholic preparations of B. trimera, but there are significant qualitative and quantitative variations between these samples ( Citation41,Citation42).
Based on this, weight-loss measurements were performed with caffeoyl derivative (5-caffeoylquinic acid) and flavonoid (quercetin) in 1 mol L−1 HCl for 24 h of immersion time. In the case of quercetin, 1% ethanol was used as a co-solvent because of its very low solubility in aqueous solution. presents the corrosion rates obtained from the measurements of weight loss in 1 mol L−1 HCl, in the absence and presence of 100 mg L−1 of 5-caffeoylquinic acid and quercetin. The inhibition efficiency levels of these compounds were 15.7% and 14.7%, respectively. These inhibition efficiency values are several times lower than those observed for the gorse extract (). It should be noted that although the concentration levels of these compounds are relatively low in the aqueous extract, the corrosion inhibition efficiency was 65.6% for 100 mg L−1 of the gorse extract. Therefore, it suggests that isolated chlorogenic acids and flavonoids do not appear to be responsible for the corrosion inhibition results observed for the gorse extract. In the present study, the HMWF, rich in macromolecules such as proteins and polysaccharides, was isolated from the aqueous extract of gorse.
Table 8. Weight-loss measurements for mild steel in 1 mol L−1 HCl solution in the absence and presence of 5-caffeoylquinic acid (5-CQA), quercetin and HMWF.
The high molecular fraction was studied by weight-loss measurements during 24 h of immersion time and by electrochemical impedance in 1 mol L−1 HCl. In weight-loss measurements, the inhibition efficiency of HMWF was 91.5% and 92.0% for 100 and 200 mg L−1 of HMWF, respectively. Under the same conditions, the aqueous gorse extract had an inhibition efficiency of 65.6% and 85.1%, respectively. Therefore, macromolecules such as proteins and/or polysaccharides, present in this extract, appear to be responsible for the inhibitory action of the aqueous extract of gorse.
shows the electrochemical impedance diagrams for mild steel in 1 mol L−1 HCl solution in the absence and presence of 200 mg L−1 of HMWF. The inhibitory action of the HMWF can be observed. As observed for the aqueous gorse extract in the impedance diagrams, the HMWF also shows only a depressed capacitive loop, where two significant effects can be observed: the charge transfer resistance increases significantly and fmax decreases in the presence of the extract (). The inhibition efficiency for HMWF was 84.7%, whereas for the gorse extract, it was 83.2%, corroborating the results obtained in the measurements of weight loss.
Figure 6. Electrochemical impedance diagrams for mild steel in 1 mol L−1 HCl medium in the absence and presence of 100 mg L−1 of HMWF.
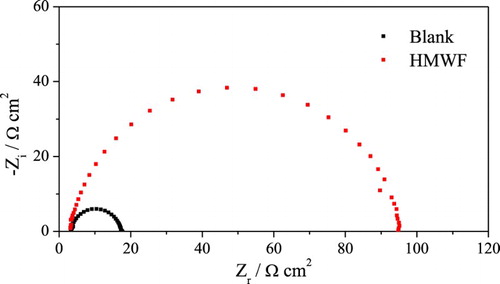
Table 9. Electrochemical parameters obtained from the electrochemical impedance spectroscopy in the presence of 200 mg L−1 of HMWF.
It is still not possible to determine which components present in the gorse extracts created their relatively high capacity to inhibit corrosion. However, it can be speculated that the macromolecules present in the gorse extracts could have been responsible for the results of this study.
Both proteins and polysaccharides are naturally occurring macromolecules, the first consisting of one or more long chains of amino acid residues linked together by peptide bonds and the second consisting of long chains of monosaccharide units linked together by glycosidic bonds. The presence of N and O in proteins and O in the polysaccharides is a potential source of electron pairs available for adsorption on the metal surface, promoting the partial blocking of the metal and the consequent inhibition of corrosion in acid media.
Polymers may be a better choice as they possess long chain molecules and show high inhibition efficiency at very low concentration in most aggressive media ( Citation43). Both proteins and polysaccharides have shown an inhibitory effect on the mild steel corrosion ( Citation43, Citation44, Citation45).
Scanning electron microscopy
(A,B) shows the results of analysis of the mild steel surface in the absence and presence of 400 mg L−1 of gorse extract for 2 h of immersion.
Figure 7. A – Morphological analysis of the mild steel surface in 1 mol L−1 HCl medium in the absence of the gorse extract. B – Morphological analysis of the mild steel surface in 1 mol L−1 HCl medium in the presence of 400 mg L−1 of the gorse extract.
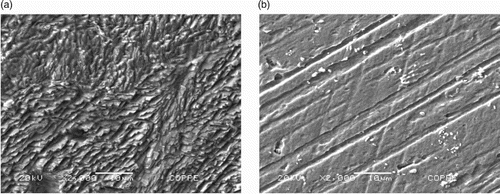
It can be seen that in (A), the surface is more rough, which means that this surface underwent corrosion due to immersion in 1 mol L−1 HCl. In (B), there is a smoother surface showing lines formed due to the abrasion treatment. This demonstrates a protective mild steel inhibitor when immersed in a 1 mol L−1 HCl solution containing the inhibitor.
Total phenols test
The reading of the sample absorbances was performed in triplicate and the values obtained are presented in . Through the analytical curve of gallic acid and the average absorbance obtained, the total phenol content in the extract of gorse extract, which had the value 115.0 mg g−1 of the extract, could be calculated.
Table 10. Absorbance values of the sample containing the gorse extract.
The test shows that the total phenol content cannot be solely responsible for the corrosion inhibition of mild steel. The studies carried out by Torres et al. ( Citation6) using 400 mg L−1 of coffee grounds and having 64.2 mg g−1 as the total phenols, resulting in a 93% IE from the electrochemical impedance tests. Souza et al. ( Citation7) also used 400 mg L−1 of aqueous green mate extract with 293 mg g−1 as total phenols, which led to an inhibition efficiency of 89%. While this study, although the total phenol concentration has been found to be higher than Torres et al. ( Citation6) and lower than Souza et al. ( Citation7), the efficiency of inhibition found at 400 mg L−1 extract of gorse through electrochemical impedance spectroscopy was 89.2%, very similar to the other studies cited. In addition, based on the results of HMWF, it is possible to propose that macromolecules as proteins and/or polysaccharides are probably responsible for the inhibitory action observed by the aqueous extract of gorse.
Conclusions
Aqueous gorse extract acts as an efficacious inhibitor to prevent corrosion of mild steel when present in a 1 mol L−1 HCl solution.
The IE increased with the [extract], immersion time and temperature.
The behavior of the gorse extract as a corrosion inhibitor of the mild steel in acid solution can be attributed to the chemisorption by the molecules present in the extract on the metal surface.
Adsorption of the extract followed a Langmuir adsorption isotherm.
The electrochemical results obtained from measurements of electrochemical impedance and polarization curves suggest that adsorption of the inhibitor does not affect the dissolution kinetics of the metal surface not covered by the inhibitor acting by surface screening.
The total phenol content cannot be solely responsible for the corrosion inhibition of mild steel.
Finally, based on the results of the HMWF, it is possible to propose that macromolecules as proteins and/or polysaccharides are probably responsible for the inhibitory action observed by the aqueous extract of gorse.
Disclosure statement
No potential conflict of interest was reported by the authors.
Notes on contributors
Rafaela da Silva Trindade obtained her Bachelor degree in Chemistry from the Federal University of Rio de Janeiro (BRAZIL). Currently, she is working at “Contraprova doping e Toxicologia”, a national company, as a laboratory assistant doing analyses of hair and urine to detect drug abuse.
Matheus Rangel dos Santos obtained his electrical technician degree from Escola Técnica Estadual Visconde de Mauá(Brazil). He is currently an undergraduate student under the supervision of Dr. Eliane D'elia at UFRJ Rio de Janeiro, Brazil. His research focuses on natural corrosion inhibitor.
Renata Fernandes Braga Cordeiro graduated in Chemistry from the University of the State of Rio de Janeiro (UERJ)(2012). She had a Scholarship for Scientific Initiation at the Laboratory of Electrochemistry and Corrosion at the Department of Analytical Chemistry of UERJ. She worked with electrodeposition from direct current, protective coatings and corrosion resistance in Zn-Co alloys. She is currently a PhD student (CNPQ Scholar) at the Federal University of Rio de Janeiro (UFRJ) under the guidance of Professor Eliane D'Elia. She develops multifunctional additives from coffee crop residues.
Eliane D'Elia holds a bachelor's degree in Chemistry from the Federal University of Rio de Janeiro (1989), a Master's degree in Metallurgical and Materials Engineering from the Federal University of Rio de Janeiro (1992) and a sandwich doctorate in Metallurgical and Materials Engineering from the Federal University of Rio de Janeiro and École Nationale Supérieure de Chimie de Toulouse - Toulouse - France (1996). She is professor of the Chemistry Institute of Federal University of Rio de Janeiro since 1993. She coordinates the research group LABEE: Electrochemical and Electroanalytical Laboratory. The LABEE group works in the Chemistry field, with emphasis in Electrochemistry, working mainly in the following subjects: electroanalytical techniques for monitoring the quality of fuels and metallic corrosion.
Additional information
Funding
References
- Raja, P.B.; Sethuraman, M.G. Mater. Lett. 2008, 62, 113–116. doi: 10.1016/j.matlet.2007.04.079
- Sharma, S.K.; Peter, A.; Obot, I.B. J. Anal. Sci. Tech. 2015, 6, 1–16. doi: 10.1186/s40543-014-0041-2
- El-Etre, A.Y.; Abdallah, M. Corros. Sci. 2000, 42, 731–738. doi: 10.1016/S0010-938X(99)00106-7
- Abiola, O.K.; Otaigbe, J.O.E. Corros. Sci. 2009, 51, 2790–2793. doi: 10.1016/j.corsci.2009.07.006
- da Rocha, J.C.; Gomes, A.C.P.; D’Elia, E.; Cruz, A.P.G.; Cabral, L.M.C.; Torres, A.G.; Monteiro, M.V.C. Int. J. Electrochem. Sci. 2012, 7, 11941–11956.
- Torres, V.V.; Amado, R.S.; de Sá, C.F.; Fernandez, T.L.; Riehl, C.A.S.; Torres, A.G.; D’Elia, E. Corros. Sci. 2011, 53, 2385–2392. doi: 10.1016/j.corsci.2011.03.021
- Souza, T.F.; Magalhães, M.; Torres, V.V.; D’Elia, E. Int. J. Electrochem. Sci. 2015, 10, 22–33.
- El Hamdani, N.; Fdil, R.; Tourabi, M.; Jama, C. Appl. Surf. Sci. 2015, 357, 1294–1305. doi: 10.1016/j.apsusc.2015.09.159
- Soltani, N.; Tavakkoli, N.; Khayatkashani, M.; Jalali, M.R.; Mosavizade, A. Corros. Sci. 2012, 62, 122–135. doi: 10.1016/j.corsci.2012.05.003
- Sethuraman, M.G.; Aishwarya, V.; Kamal, C.; Edison, T.J.I. Arab. J. Chem. 2017, 10, S522–S530. doi: 10.1016/j.arabjc.2012.10.013
- Verma, D.K.; Khan, F. Chem. Lett. Rev. 2016, 9, 52–60. doi: 10.1080/17518253.2015.1137976
- Noor, E.A. Int. J. Electrochem. Sci. 2007, 2, 996–1017.
- Abboud, Y.; Tanane, O.; El Bouari, A.; Salghi, R.; Hammouti, B.; Chetouani, A.; Jodeh, S. Corros. Eng. Sci. Tech. 2016, 51, 557–565.
- Saleh, R.M.; Ismael, A.A.; El Hosary, A. Brit. Corros. 1982, 17, 131–135. doi: 10.1179/000705982798274345
- Bentrah, H.; Rahali, Y.; Chala, A. Corros. Sci. 2014, 82, 426–431. doi: 10.1016/j.corsci.2013.12.018
- de Barros, I.B.; Kappel, M.A.A.; dos Santos, P.M.; Veiga Junior, V.F.; D’Elia, E.; Bastos, I.N. Mater. Res. 2016, 19, 187–194. doi: 10.1590/1980-5373-MR-2015-0494
- Okafor, P.C.; Ebenso, E.E. Pigm. Resin Technol. 2007, 36, 134–140. doi: 10.1108/03699420710748992
- Eddy, N.O.; Odoemelam, S.A.; Odiongenyi, A.O. Green Chem. Lett. Rev. 2009, 2, 111–119. doi: 10.1080/17518250903170868
- Pereira, S.S.A.A.; Pegas, M.M.; Fernandez, T.L.; Magalhaes, M.; Schontag, T.G.; Lago, D.C.; Senna, L.F.; D’Elia, E. Corros. Sci. 2012, 65, 360–366. doi: 10.1016/j.corsci.2012.08.038
- Rodriguez-Clemente, E.; Gonzalez-Rodriguez, J.G.; Valladares-Cisneros, M.G.; Chacon-Nava, J.G. Green Chem. Lett. Rev. 2015, 8, 49–58. doi: 10.1080/17518253.2015.1071435
- Eddy, N.O.; Ekwumemgbo, P.A.; Mamza, P.A.P. Green Chem. Lett. Rev. 2009, 2, 223–231. doi: 10.1080/17518250903359941
- Murthy, Z.V.P.; Vijayaragavan, K. Green Chem. Lett. Rev. 2014, 7, 209–219. doi: 10.1080/17518253.2014.924592
- Asipita, S.A.; Ismail, M.; Majid, M.Z.A.; Majid, Z.A.; Abdullah, C.; Mirza, J. J. Clean. Prod. 2014, 67, 139–146. doi: 10.1016/j.jclepro.2013.12.033
- Raja, P.B.; Ghoreishiamiri, S.; Ismail, M. Surf. Rev. Lett. 2015, 22, No. 3, 1550040. doi: 10.1142/S0218625X15500407
- Raja, P.B.; Ismail, M.; Ghoreishiamiri, S.; Mirza, J.; Ismail, M.C.; Kakooei, S.; Rahim, A.A. Chem. Eng. Commun. 2016, 203, 1145–1156. doi: 10.1080/00986445.2016.1172485
- Ismail, M.; Raja, P.B.; Salawu, A.A. Recent Developments in Materials Science and Corrosion Engineering Education, Scopus: Abu Dhabi, 2015, pp 118–146 Chapter 7.
- Ibrahim, T.H.; Chehade, Y.; Zour, M.A. Int. J. Electrochem. Sci. 2011, 6, 6542–6556.
- Okafor, P.C.; Ikpi, M.E.; Uwah, I.E.; Ebenso, E.E.; Ekpe, U.J.; Umoren, S.A. Corros. Sci. 2008, 50, 2310–2317. doi: 10.1016/j.corsci.2008.05.009
- da Rocha, J.C.; Gomes, J.A.C.P ;D’Elia, E. Corros. Sci. 2010, 52, 2341–2348. doi: 10.1016/j.corsci.2010.03.033
- Sharma, S.K.; Mudhoo, A.; Jain, G.; Sharma, J. Green Chem. Lett. Rev. 2010, 3, 7–15. doi: 10.1080/17518250903447100
- Souza, E.C.C.A.; Ripper, B.A.; Perrone, D.; D’Elia, E. Mater. Res. 2016, 19, 1276–1285. doi: 10.1590/1980-5373-mr-2015-0740
- de Alencar, M.F.A.; de Oliveira, L.R.F.; da S. Gomes, R.; Gomes, F.F.S.; de A. Neto, J.A.M.; J.M.F. Júnior; da Silva, R.C.B. 5th North-Northeast Congress of Chemistry, Natal, RN, April 8–12, 2013.
- Menezes, A.P.S.; da Silva, J.; Rossato, R.R.; Santos, M.S.; Decker, N.; da Silva, F.R.; Cruz, C.; Dihl, R.R.; Lehmann, M.; Ferraz, A.B.F. Ecotoxicol. Environ. Saf. 2015, 114, 9–16. doi: 10.1016/j.ecoenv.2015.01.001
- Pocá, A.M.P.C. Biomassa, Óleo essencial, perfil fitoquímico e nutrientes da carqueja sob influência de fontes e doses de nitrogênio. MSc Dissertation, Universidade Federal do Paraná, Paraná, BR, 2005.
- Singleton, V.L.; Orthofer, R.; Lamuela-Raventós, R.M. Methods Enzymol. 1999, 299, 152–178. doi: 10.1016/S0076-6879(99)99017-1
- Akalezi, C.O.; Ogukwe, C.E.; Ejele, E.A.; Oguzie, E.E. Int. J. Corros. Scale Inhib. 2016, 5, 132–146. doi: 10.17675/2305-6894-2016-5-2-3
- Torres, V.V.; Rayol, V.A.; Magalhães, M.; Viana, G.M.; Aguiar, L.C.S.; Machado, S.P.; Orofino, H.; D’Elia, E. Corr. Sci. 2014, 79, 108–118. doi: 10.1016/j.corsci.2013.10.032
- El-Awady, A.A.; Abd-El-Nabey, B.A.; Aziz, S.G. J. Electrochem. Soc. 1992, 139, 2149–2154. doi: 10.1149/1.2221193
- Karthikaiselvi, R.; Subhashini, S. J. Assoc. Arab. Univ. Basic Appl. Sci. 2014, 16, 74–82.
- de Souza, F.S.; Gonçalves, R.S.; Spinelli, A. J. Braz. Chem. Soc. 2014, 25, 81–90.
- Simões-Pires, C.A.; Queiroz, E.F.; Henriques, A.T.; Hostettmann, K. Phytochem. Anal. 2005, 16, 307–314. doi: 10.1002/pca.826
- Livero, F.A.R.; da Silva, L.M.; Ferreira, D.M.; Galuppo, L.F.; Borato, D.G.; Prando, T.B.L.; Lourenço ;. Stefanello, M.E.A.; Acco, A. Naunyn-Schmiedeberg’s Arch. Pharmacol. 2016, 389, 985–998. doi: 10.1007/s00210-016-1262-2
- Banerjee, S.; Srivastava, V.; Singh, M.M. Corr. Sci. 2012, 59, 35–41. doi: 10.1016/j.corsci.2012.02.009
- Mobin, M.; Rizvi, M. Carbohydr. Polym. 2017, 160, 172–183. doi: 10.1016/j.carbpol.2016.12.056
- Zhang, F.; Pan, J.; Claesson, P.M. Electrochim. Acta 2011, 56, 1636–1645. doi: 10.1016/j.electacta.2010.10.033