ABSTRACT
Lead oxide (PbO) is known as an important industrial material. It is a semiconductor that has two crystalline forms, litharge (tetragonal crystalline structure) and massicot (orthorhombic crystalline structure). In this research, lead oxide nanoparticles (PbO-NPs) have been synthesized through the utilization of gelatin as a stabilizer, while its cytotoxic effects were investigated on Neueo2A cancer cell line. The generation of PbO-NPs was confirmed by the UV-Vis spectroscopy, PXRD, TG-DTA, EDX, and FESEM. The results of diffraction patterns of synthesized nanoparticles show the formation of both form α-PbO-NPs (tetragonal) and β-PbO-NPs (orthorhombic). The particles size of synthesized PbO-NPs was calculated to be around 10–20 nm. The cytotoxic activity of the synthesized PbO-NPs indicated that concentrations under 30 μg/mL have insignificant toxicity.
GRAPHICAL ABSTRACT
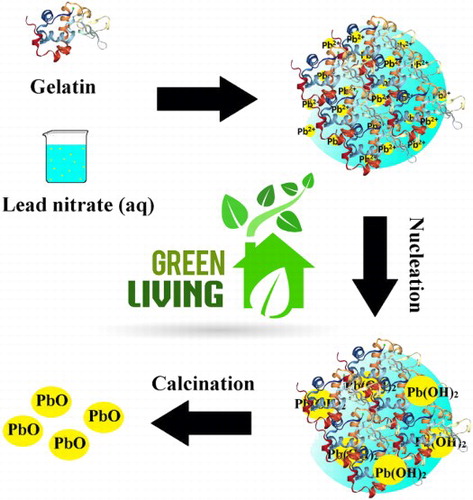
1. Introduction
Lead oxide (PbO) is known as an important industrial material, which has been widely utilized in batteries ( Citation1), gas sensors ( Citation2), pigments ( Citation3), ceramics ( Citation4), and glass industry ( Citation5). The colors that contain lead-based pigments seem to have interesting properties including rustproof, anti-bacterial, and anti-algae, which are extensively employed in shipbuilding, construction skeleton, and road construction ( Citation6,Citation7). PbO is a semiconductor that has two crystalline forms, litharge (tetragonal crystalline structure) and massicot (orthorhombic crystalline structure) ( Citation8,Citation9). The color of litharge crystals is red with α-PbO form, which has shown to be stable at low temperatures; while massicot crystals are yellow with a β-PbO form and seem to be stable at high temperatures. The conversion phase of α-PbO to β-PbO occurs at approximately 490°C. Lead oxides are useful photovoltaic materials ( Citation10) that mostly applied as optical active semiconductors with the band gaps of 1.92 and 2.7 eV ( Citation11,Citation12). Recently, PbO nanostructures synthesized through the usage of several methods including thermal decomposition ( Citation12,Citation13), Sol–gel pyrolysis ( Citation14) thermal decomposition under the microwave radiation ( Citation15), chemical deposition ( Citation16), and solvothermal method ( Citation17). Since the mentioned procedures are usually expensive, time-consuming, and toxic, it is crucial to discover fast, low-cost, and environmental-friendly methods, in which biosynthesis could be considered as one of them ( Citation18–22). Animal or plant-based extracts inherently have functional groups with high affinity towards metal ions, aforementioned characteristics as a stabilizer could be deduced from its interactions. Knowing how to apply biopolymers in the synthesis of nanoparticles helps to control size, shape and other properties more efficiently ( Citation23). Obtaining more knowledge in biosynthetic methods could revolutionize preparation and designing of new nanomaterials including metal/metal oxides ( Citation24).
Lead is widely used in industrial applications while it is known as a toxic compound and its oral and inhaled consumption is quite harmful; hence, exposure to lead is a significant concern for general health ( Citation25–28). Lead-containing compounds can cause different types of undesirable outcomes such as genetic toxicity ( Citation29), oxidative stress ( Citation27,Citation30), and neurological effects ( Citation31). As it is obvious, lead oxide have a great impact on the environment, it is necessary to find solutions for lead pollution. Nanotechnology and biosynthesis could be employed to produce metal oxide nanoparticles ( Citation32,Citation33). Biosynthesis of nano-sized lead oxide (PbO-NPs) could enhance its properties while reducing toxic substances during the synthetic procedure ( Citation34). In this research, PbO-NPs have been synthesized through the utilization of natural gelatin-based stabilizer and more importantly, the influence of preparation method on cytotoxicity of PbO-NPs was explored on Neueo2A cancer cell line.
2. Materials and methods
2.1. Synthesis of PbO-NPs
The PbO-NPs were biosynthesized by application of gelatin as capping agents. Gelatin (4.0 g) was added to 100 ml of distilled water and the suspension was then positioned in a water bath with the temperature of 60°C for 90 min until a clear and yellow solution was induced. Then, Pb(NO3)2 was slowly added to the solution (5.0 g) and was stirred in the water bath that had a temperature of 75°C, until the formation of a lemon color resin. The resulted resin was dried in an oven at 90°C for 2 h and then, it was calcinated at 450°C, 500°C, 550°C, and 600°C for the duration of 90 min, separately. The final resultant was observed to be an orange powder, known as PbO-NPs.
2.2. Characterization of PbO-NPs
The crystalline structure of the synthesized PbO-NPs has been determined through the powder X-ray diffraction (PXRD, INEL model Equinox 3000, France). The UV-Vis studies on these nanoparticles have been carried out through the usage of UV-Vis spectrophotometer (Rayleighuv-2100 model, Chine). The field emission-scanning electron microscope (FESEM) of PbO-NPs were obtained by the implementation of MIRA3 TESCAN. The thermogravimetric analyses of PbO-NPs has been performed through the utilization of thermal gravimetric analysis (TG-DTA, BAHR STA 503 model, Germany).
2.3. Cell line and cell culture
The Neuroblastoma (Neuro2A) cell line was obtained and prepared from Pasteur Institute of Iran. Cells were incubated in the modified Dulbecco’s media (1 g/l glucose, 2 mM glutamine) with 10% FBS, 100 μg/ml of Streptomycin, and 100 U/ml of Penicillin, at a temperature of 37°C and the atmosphere of CO2 with 5% moisture.
2.4. Cytotoxicity evaluation of PbO-NPs
The cytotoxic activity of prepared PbO-NPs was measured by using 3-(4,5-Dimethylthiazol-2-yl)-2,5-diphenyltetrazolium bromide (MTT). Briefly, 200 µl of the cell suspension was added into a 96-well tissue culture plate (1 × 104 Cell/well) and incubated for 24 h, at 37°C. Then, 50 µl of the synthesized nanoparticles (0-500 µg/ml) was poured into each well, while the plate was incubated for a time period of 24 h. In this project, we marked the cell suspension, which held the culture medium, as the control. Afterwards, 20 µl of the PBS buffer that contained MTT (5 mg/ml) was appended to each well, while the plate was incubated for 4 h at 37°C. At the end, 100 µl of DMSO was added to each well and their optical absorbance was measured at 570 nm, which was done through the application of a micro-plate reader. Cell viability was expressed as a percent relative to the control.
2.5. Statistical analysis
The statistical analysis has been performed by the GraphPad Prism 5, while each value has been presented as mean ± SD. The statistical comparisons of multi-group data have been analyzed through the usage of two-way ANOVA, where the values of *p < .05 were considered statistically significant. All of the mentioned tests have been performed in triplicates.
3. Results and discussion
PbO-NPs have been synthesized by adding lead nitrate solution to gelatin in water as the solvent. Gelatin is a protein with three spiral chains that include positive and negative charges. In addition, it contains hydrophobic groups that is interacting with water to create stable levels through the formation of space bridges; therefore, gelatin can act as a green stabilizer for the synthesis of nanoparticles ( Citation23). The synthesized PbO-NPs mechanism through the utilization of gelatin is presented in . As it is obvious, gelatin acting as a stabilizer, hence, different functional groups such as carboxylic acids and hydroxyls were interacting with Pb2+ in order to form a homogenized solution. In the next step, nucleation of Pb2+ ion proceeds by the formation lead hydroxide nuclei. Gelatin prevents the construction of large nuclei. Therefore, uniform nanoparticle would be obtained after calcination.
The formation of PbO was confirmed by FT-IR spectroscopy (). The broad peak observed between 1300–1400 cm−1 in FT-IR spectra of α- and β-PbO attributed to Pb-O stretching vibrations ( Citation35).
The electronic spectra of the synthesized nanoparticles have displayed a wide peak in 250-260 nm at the calcination temperatures of 450°C, 500°C, 550°C, and 600°C (). It is indicated by the achieved results of the study that the optimum condition of calcination seems to be 550°C for the duration of 2 h. By increasing the temperature, the alpha form of lead oxide converts to beta and therefore, it is expected that the spectra would display a red shift. As a result, as the temperature is increased from 450°C to 600°C, the wavelength is repositioned from 252 to 260 nm.
The particle size of synthesized nanoparticles has been measured through the band-gap of electron spectrum by using the Tauc equation ( Citation36,Citation37): (αhυ) = A (hυ-Eg)nWhere A stands as the absorption coefficient; hυ would be the photon energy and Eg stands as the band-gap. As illustrated in the , the calculated values of band-gap for the synthesized PbO-NPs have been observed to be 4.38, 4.39, 4.30, and 4.02 eV at the calcination temperatures of 450, 500, 550, and 600°C, respectively. There is an inverse relationship between band-gap and particle size in a way that increases the band-gap will result in a smaller particle size. The calculated values of band-gap are much larger than the ordinary lead oxide band-gap (band-gap of α-PbO and β-PbO are 1.92 and 2.7 eV, respectively) ( Citation16); this fact clearly illustrates that the synthesized powder is in nanoscale and its alpha form is quiet smaller than the beta.
The PXRD analysis was done at a scan rate of 2°/min. The PXRD pattern of the synthesized PbO-NPs at different calcination temperatures is demonstrated in . All of the peaks with Miller indices of (010), (110), (220), (11), (002), (200), (202), (011), (020), (112), (121), (202), (022), (113), (311), (222), (004), (400), (204), (313), (024), and (124) can be indexed to the crystal structure of PbO-NPs ( Citation38,Citation39). The diffraction patterns of α-PbO-NPs and β-PbO-NPs have confirmed the tetragonal and orthorhombic structures, respectively, which are in accordance to the JCPDS (16). Also, the PXRD spectra () has displayed the tetragonal and orthorhombic structures at 450°C and 500°C, respectively; therefore, there exist both forms of α and β-PbO in the particular temperature between 450°C and 500°C. It is vital to mention that due to the changes in its phase, the characteristic peaks of α-PbO are less visible at 500°C. Typically, the phase change of α-PbO to the form of β-PbO is induced at 489°C, and the pure form of α- can be reached only in the range of 240–260°C ( Citation16).
The thermal analysis of TGA-DTA (thermogravimetric analysis-differential thermal analysis) regarding the synthesized sample was taken to obtain the proper calcination temperature to reach the pure phase (). The test was performed in the temperature interval of 20–1000°C in the atmospheric air. On the DTA curve, the temperature range of 80–235°C has indicated the gradual dehydration and discharge of crystalline and absorbed water, while the first weight loss (35%) has been observed in this temperature range on the TGA curve. The second weight loss (16%) has been induced through 235–460°C, which is related to the decomposition of gelatins chemical bond. The third weight loss (7%) seemed to be related to lead oxidation in the temperature interval of 460–480°C, and the last weight loss (6%) has been associated with the final oxidation of lead. As it is demonstrated in , there have been no weight changes between 550°C and 880°C, indicating that PbO-NPs are stable in these particular temperatures.
The FESEM image of the synthesized nanoparticles has displayed that the particles are in nanoscale and uniform in shape (). This image has also shown that in optimal conditions, the size of particles ranges from 10 to 20 nm. Also, the EDX graph of the nanoparticles only displays the presence of lead and oxygen elements in the synthesized nanoparticle, which is due to its high purity ().
The investigation on the cytotoxic characteristics of PbO-NPs has been explored on Neuro2a cells by MTT assay ( Citation40). This test has been completed by different doses of PbO-NPs from 0 to 500 μg/ml (). As it has been observed in the results, concentrations of synthesized nanoparticles that were below 30 μg/ml seemed to contain lower toxicity. From this, it can be deduced that PbO-NPs at concentrations below 30 μg/ml are harmless and industrially applicable with low risk ( Citation41).
4. Conclusion
The PbO-NPs is one of the most industrially used metal nanoparticles. Throughout this study, we have tried to produce PbO-NPs in a facile, cheap, and nontoxic way, in other words, the synthesis process has been accomplished by a “green” approach. Accordingly, PbO-NPs have been synthesized by applying gelatin as a capping agent. Spectral studies, including UV-Vis spectroscopy, PXRD, TG-DTA, EDX, and FESEM indicated that PbO-NPs had a uniform particle distribution size range from 10 to 20 nm. In accordance to the results of the investigation on the toxicity of the synthesized nanoparticles, these nanoparticles seem to be at low-risk regarding their usage in the industry as long as they are applied in concentrations below 30 μg/ml.
Disclosure statement
No potential conflict of interest was reported by the authors.
Notes on contributors
Abdolhossein Miri, Ph.D. of Pharmacognosy, Associate Professor at Faculty of Pharmacy of Zabol University of Medical Sciences with research interests in Synthesis and application of nanomaterials in the field of pharmacy and drug delivery, published 50+ peer-reviewed international papers.
Mina Sarani, MSc in inorganic chemistry and expert responsible for the research and development department at Zabol University of Medical Sciences. She acts in green synthesis of different nanomaterials and investigation of their applications in medicine and industrial.
Alireza Hashemzadeh, a postdoctoral researcher in the nanoscience center at Mashhad University of Medical Sciences, the specialist in design and synthesis of new inorganic nanoparticles, porous and mesoporous materials for different purposes. He has 14 published works in this regard.
Zahra Mardani, assistant professor of Inorganic Chemistry at Urmia University with research interests in designing and preparing of inorganic complexes and nanomaterials and investigation of their chemical and medical properties, published 35+ peer-reviewed international papers.
Majid Darroudi, assistant professor of Nanoscience at Mashhad University of Medical Sciences with research interests in green synthesis of different nanomaterials and investigation of their applications in medicine and pharmacy, published 90+ peer-reviewed international papers with 2 chapter books in both fields.
ORCID
Alireza Hashemzadeh http://orcid.org/0000-0001-7460-3312
Zahra Mardani http://orcid.org/0000-0002-3749-5399
Majid Darroudi http://orcid.org/0000-0002-2624-7242
References
- Sonmez, M.; Kumar, R. Hydrometallurgy 2009, 95, 53–60. doi: 10.1016/j.hydromet.2008.04.012
- Šljukić, B.; Banks, C.E.; Crossley, A.; Compton, R.G. Analytica. Chimica Acta. 2007, 587, 240–246. doi: 10.1016/j.aca.2007.01.041
- Senvaitiene, J.; Smirnova, J.; Beganskiene, A.; Kareiva, A. Acta. Chimica Slovenica 2007, 54.
- Jaffe, B.; Roth, R.; Marzullo, S. J. res. Natl. Bureau Stand. 1955, 55, 239–254. doi: 10.6028/jres.055.028
- Dumbaugh, W.H.; Lapp, J.C. J. Am. Ceram. Soc. 1992, 75, 2315–2326. doi: 10.1111/j.1151-2916.1992.tb05581.x
- Ragg, M. Trans. Electrochem. Soc. 1933, 64, 59–68. doi: 10.1149/1.3504552
- Blair, T. J. Power Sources 1998, 73, 47–55. doi: 10.1016/S0378-7753(97)02781-X
- Eya, D.; Ekpunobi, A.; Okeke, C. Pac. J. Sci. Technol. 2006, 7, 114.
- Klein, C.A. J. Appl. Phys. 1968, 39, 2029–2038. doi: 10.1063/1.1656484
- Darwish, A.; El-Zaidia, E.; El-Nahass, M.; Hanafy, T.; Al-Zubaidi, A. J. Alloys Compd. 2014, 589, 393–398. doi: 10.1016/j.jallcom.2013.11.218
- Bangi, U. K.; Han, W.; Yoo, B.; Park, H.-H. J. Nanoparticle res. 2013, 15, 2070. doi: 10.1007/s11051-013-2070-y
- Salavati-Niasari, M.; Mohandes, F.; Davar, F. Polyhedron 2009, 28, 2263–2267. doi: 10.1016/j.poly.2009.04.009
- Cimen, E.; Gumus, I.; Arslan, H. J. Mol. Struct. 2018, 1166, 397–406. doi: 10.1016/j.molstruc.2018.04.024
- Karami, H.; Ghamooshi-Ramandi, M. Int. J. Electrochem. Sci. 2013, 8, 7553–7564.
- Li, S.; Yang, W.; Chen, M.; Gao, J.; Kang, J.; Qi, Y. Mater. Chem. Phys. 2005, 90, 262–269. doi: 10.1016/j.matchemphys.2004.02.022
- Mythili, N.; Arulmozhi, K. Int. J. Sci. Eng. Res. 2014, 5, 412–416.
- Gao, P.; Liu, Y.; Bu, X.; Hu, M.; Dai, Y.; Gao, X.; Lei, L. J. Power Sources 2013, 242, 299–304. doi: 10.1016/j.jpowsour.2013.05.077
- Suresh, D.; Nethravathi, P.; Kumar, M.P.; Naika, H.R.; Nagabhushana, H.; Sharma, S. Mater. Sci. Semicond. Process. 2015, 40, 759–765. doi: 10.1016/j.mssp.2015.06.088
- Darroudi, M.; Sarani, M.; Oskuee, R.K.; Zak, A.K.; Hosseini, H.A.; Gholami, L. Ceram. Int. 2014, 40, 2041–2045. doi: 10.1016/j.ceramint.2013.07.116
- Rahdar, A.; Aliahmad, M.; Hajinezhad, M.R.; Samani, M. J. Mol. Struct. 2018, 1173, 166–172. doi: 10.1016/j.molstruc.2018.06.092
- Zare, E.; Pourseyedi, S.; Khatami, M.; Darezereshki, E. J. Mol. Struct. 2017, 1146, 96–103. doi: 10.1016/j.molstruc.2017.05.118
- Sone, B.T.; Manikandan, E.; Gurib-Fakim, A.; Maaza, M. Green Chem. Lett. Rev. 2016, 9, 5–90.
- Darroudi, M.; Hakimi, M.; Sarani, M.; Oskuee, R.K.; Zak, A.K.; Gholami, L. Ceram. Int. 2013, 39, 6917–6921. doi: 10.1016/j.ceramint.2013.02.026
- Hasanzadeh, L.; Kazemi Oskuee, R.; Sadri, K.; Nourmohammadi, E.; Mohajeri, M.; Mardani, Z.; Hashemzadeh, A.; Darroudi, M. Life Sci. 2018, 212, 233–240. doi: 10.1016/j.lfs.2018.10.010
- Goodsell, D.; Bionanotechnology, S. Lessons from Nature; John Wiley & Sons, 2004.
- Martinez-Haro, M.; Green, A.J.; Mateo, R. Environ. Res. 2011, 111, 530–538. doi: 10.1016/j.envres.2011.02.012
- Kordas, K.; Roy, A.; Vahter, M.; Ravenscroft, J.; Mañay, N.; Peregalli, F.; Martínez, G.; Queirolo, E.I. Environ. Res. 2018, 166, 507–515. doi: 10.1016/j.envres.2018.06.028
- de Freitas, C.U.; De Capitani, E.M.; Gouveia, N.; Simonetti, M.H.; de Paula e Silva, M.R.; Kira, C.S.; Sakuma, A.M.; de Fátima Henriques Carvalho, M.; Duran, M.C.; Tiglea, P.; de Abreu, M.H. Environ. Res. 2007, 103, 338–344. doi: 10.1016/j.envres.2006.09.004
- Kaehler, T. Clin. Chem. 1994, 40, 1797–1799.
- Tandon, S.K.; Singh, S.; Prasad, S.; Srivastava, S.; Siddiqui, M.K.J. Environ. Res. 2002, 90, 61–66. doi: 10.1006/enrs.2002.4386
- Schadler, L.S. Nanocomposite Sci. Technol. 2003, 77–153.
- Diallo, A.; Beye, A.C.; Doyle, T.B.; Park, E.; Maaza, M. Green Chem. Lett. Rev. 2015, 8, 30–36.
- Rokade, A.A.; Patil, M.P.; Yoo, S.I.; Lee, W.K.; Park, S. S. Green Chem. Lett. Rev. 2016, 9, 216–222. doi: 10.1080/17518253.2016.1234005
- Narayanan, R. Green Chem. Lett. Rev. 2012, 5, 707–725. doi: 10.1080/17518253.2012.700955
- Güngör, A.; Genç, R.; Özdemir, T. J. Turkish Chem. Soc., Sect. A: Chem. 4, 1017–1030.
- Nourmohammadi, E.; Kazemi Oskuee, R.; Hasanzadeh, L.; Mohajeri, M.; Hashemzadeh, A.; Rezayi, M.; Darroudi, M. Ceram. Int. 2018.
- Hashemzadeh, A.; Amini, M.M.; Najafi, E.; Khavasi, H.R. Res. Chem. Intermed. 2017, 43, 5741–5753. doi: 10.1007/s11164-017-2960-1
- Arulmozhi, K.; Mythili, N. AIP Adv. 2013, 3, 122122. doi: 10.1063/1.4858419
- Mythili, N.; Arulmozhi, K. Appl. Phys. A 2015, 118, 261–267. doi: 10.1007/s00339-014-8671-1
- Charbgoo, F.; Ramezani, M.; Darroudi, M. Biosens. Bioelectron. 2017, 96, 33–43. doi: 10.1016/j.bios.2017.04.037
- Darroudi, M.; Sarani, M.; Oskuee, R.K.; Zak, A.K.; Amiri, M.S. Ceram. Int. 2014, 40, 2863–2868. doi: 10.1016/j.ceramint.2013.10.026