ABSTRACT
Azidation of plant seed oils was re-investigated using methods reported in the literature, to re-examine if triacylglycerol backbone, important for maintaining biodegradability in plant oil products is retained in the final azidated oil. Reaction of NaN3 with epoxidized Adenopus breviflorus oil (EADBO) using NH4Cl as catalyst (Method A), gave acidolysis products and mixture of products containing triacylglycerol backbone. Reaction of EADBO with NaN3 in water using an ionic liquid, 1-methyl imidazolium tetrafluoroborate ([Hmim]BF4−), as catalyst (Method B), generated a product containing only triacylglycerol backbone while product of reaction of EADBO with NaN3 in DMF, using [Hmim]BF4− catalyst (Method C) gave highest yield but did not contain any triacylglycerol backbone. Thus, Method B was best for environmentally friendliness of its azidated product. Azido compounds generally prepared from petrochemicals may now be prepared from plant oil source using method B for preparation of biodegradable vicinal hydroxyl triglyceride which is very versatile in surfactant industries.
GRAPHICAL ABSTRACT
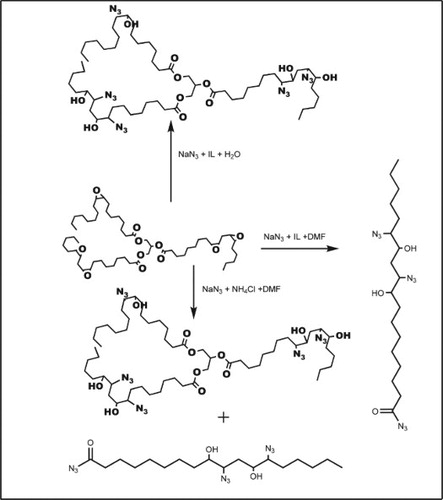
Introduction
At present, most of the industrial chemicals are derived from fossil fuels, and their production is accompanied by the emission of undesirable greenhouse gases. The availability of fossil fuels is decreasing, while their prices are increasing. In such a scenario, plant oils can be good, renewable, and functionally equivalent alternatives to fossil fuel-based raw materials. This is because plant oils can be readily modified to match the different end use-requirements of many industrial products ( Citation1). The renewability, non-toxicity, sustainability, cost-effectiveness, availability, and biodegradability of the plant oil-based chemical products are the major driving forces for choosing plant oils as an alternative.
Compounds containing the azide group has been found to be a useful intermediate in organic syntheses ( Citation2). Such compounds are good starting materials for preparing nitrogen heterocycles and they also serve as good synthons in click reactions ( Citation3). While many pure organic compounds have been azidated ( Citation4–8), there have been some reports on the synthesis of azido derivatives from pure fatty acids ( Citation3, Citation9–11). However, only a few studies on the azidation of complex unsaturated systems in plant oils (Citation 3, Citation12,Citation13) have been reported. To replace petrochemicals in this regard, this work reports the preparation of azido compounds from a plant seed oil source, Adenopus breviflorus benth oil via epoxy cleavage. The azido compound prepared would be an appealing straight forward access to preparation of biodegradable vicinal hydroxyl triglyceride which is very versatile in the surfactant industries
Adenopus beviflorus (ADB), a member of the curcubitae family, is grown in the middle belt of Nigeria. The percentage of oil in its seeds is moderately high, about 56%, and consists mainly of unsaturated fatty acids (85.1%), with a predominance of linoleic acid ( Citation14). The preponderance of unsaturated fatty acids in the oil makes it amenable to epoxidation and the epoxidised oil is required for the preparation of azidated oil. In this study, we have investigated the azidation of fatty acid chain of epoxidized Adenopus breviflorus benth seed oil (EADBO). Generally, azidation may be attained by a ring-opening nucleophilic addition of NaN3 to the epoxy group. Such derivatives obtainable from plant oils would extend the applications of the oils in polymer syntheses, especially through click reactions. Click reaction which is the Huisgen (3 + 2) dipolar cycloaddition between an azide and a terminal alkyne is an appealing reaction that has been used extensively in materials synthesis ( Citation3). Furthermore, we have also re-investigated the azidation of plant seed oils using some of the methods reported in the literature, in order to check if the triacylglycerol backbone is retained in the final azidated oil. This is because the triacylglycerol backbone is important for maintaining the biodegradability of the plant oil products.
Materials and methods
Materials
All the reagents were obtained from Sigma Aldrich, Germany, and used as received. Adenopus breviflorus benth oil (ADBO) seeds were obtained from a local farm in Ado-Ekiti, Ekiti state, Nigeria. Improper seeds were removed manually by hand picking. The good seeds were washed with water, air-dried, and milled using Christy mill. Oven-dried (103°C) milled samples were extracted by Soxhlet extractor using n-hexane. The solvent was removed over rotary evaporator. The extracted crude oil was refined by agitating with 18 M NaOH (1:30 (g/g) of alkali:crude oil) for 15 min. The resulting mixture was heated from 75°C to 80°C in order to break the soap stock. The neutral oil was separated from the mixture by centrifugation.
Characterization
The Fourier transform infrared (FTIR) spectra were recorded on Bruker vertex FTS 700 FTIR spectrophotometer. Ten scans were collected in the range 4000–400 cm−1. 1H nuclear magnetic resonance (NMR) and 13C NMR spectra were collected on a Bruker Avance 400 (Bruker Instruments, Karlsruhe, Germany) NMR spectrometer operating at 400.6 MHz. The following parameters were used for the gated decoupling pulse sequence for data collection: number of scans, 256; acquisition time, 1.366 s; and pulse width 10.3 µs. The free induction decay was transformed and zero filled to 300 K to give a digital resolution of 2 Hz/point.
Elemental analyses of the samples were carried out on a Vario EL CHNS elemental analyzer (Elementar Company, Germany). Differential scanning calorimetry (DSC) measurements were carried out on a Mettler Toledo, DSC 822e thermal analyzer. Briefly, 5 mg sample was weighed into an aluminum pan with a pinhole lid, hermetically sealed, and heated at 10°C min−1 from −50 to 80°C. The molecular weight distributions were determined by gel permeation chromatography (GPC) performed on Waters 515 HPLC pump aided with a Knauer Smartline RI Detector 2300, using two 5 µm mixed C columns and one PLgel 1000A0 (5 µm resin) column. The samples were eluted with tetrahydrofuran at a flow rate of 1 mL/min at 25°C. Polystyrene was used as the standard.
Epoxidation of Adenopus breviflorus benth oil (ADBO)
ADBO (16.8 g, iodine value = 120 mg KOH/g, oxirane value = 0; IR (KBr film cm−1): 3008, 2934 (HC = CH), 1747 (–CH2 COO−), 1650 (C=C), 1240, 1158, and 1106 (triglyceride ester triplet), 724 (C–H bending)) was dissolved in 200 mL ethyl methyl ketone and stirred vigorously after the addition of 65.6 g (780 mM) NaHCO3 and 5 g tetrabutylammonium chloride, which was used as a phase transfer catalyst (PTC). Following this, a solution of OxoneTM, (monopotassium peroxosulfate; 132 g, 215 mM) in 750 mL water was added dropwise in two parts at an interval of 1 h. To protect the reaction from light, the reaction flask was wrapped in a foil. Once the addition of OxoneTM was complete, the reaction was allowed to proceed for another 2 h, after which the reaction mixture was diluted with water (1 L) and extracted thrice using 100 mL diethyl ether every time. The combined organic extract was washed with water and dried over anhydrous Na2SO4. The solvent was removed in vacuo. The epoxidized ADBO had the following specifications: iodine value = 0.3 mg KOH/g, oxirane value = 4.2, IR (KBr film cm−1): 824 (C–O–C oxirane), 1650 (C=C), 1747 (CH2 COO−), 1240, 1158, and 1106 (triglyceride ester triplet), and 724 (C–H bending).
Synthesis of ionic liquid 1-methylimidazolium tetrafluoroborate
1-Methylimidazolium tetrafluoroborate ([Hmim]BF4−) was synthesized as described in a previous report ( Citation15).
Azidation of Adenopus breviflorus benth oil with NaN3 using NH4Cl as a catalyst (Method A)
EADBO (10.5 g, 42 mM) was dissolved in 30 mL dimethylformamide and heated at 60°C with vigorous stirring for 5 min after the addition of 4.494 g (84 mM) NH4Cl. Then, 5.46 g (84 mM) of NaN3 was added to one portion with continuous stirring, and the reaction was allowed to proceed till such time that no signals from the oxirane ring were detected in the IR and NMR spectra. The supernatant was decanted; the residue was extracted twice with ethyl acetate and added to the supernatant. The organic extract was washed with water and dried over anhydrous Na2SO4. The organic solvent was removed in vacuo after this. The product showed the following IR frequencies (KBr film cm−1): 3400 (CH OH), 2100 (CH N3 ), 1740 (CH2 COO−), and 1670 (CON3).
Azidation of Adenopus breviflorus benth oil with NaN3 in water using [Hmim]BF4− as a catalyst (Method B)
To 10 mL of deionized water in a 50 mL round-bottom flask, 10.2 g of [Hmim]BF4− and 5.5 g of EADBO was added. To this, 0.98 g (15 mmol) NaN3 dissolved in 10 mL water was added while stirring continuously and heated at 65°C for two and half days. The mixture was cooled to room temperature and extracted twice with ethyl acetate in a separatory funnel. The combined ethyl acetate extract was successively washed with saturated sodium chloride solution and water, dried over anhydrous Na2SO4 and filtered. The solvent was evaporated using a rotary evaporator. The product showed the following IR frequencies (KBr film cm−1): 3450 (CH OH), 2100 (CH N3 ), and 1747 (CH2 COO−).
Azidation of Adenopus breviflorus benth oil with NaN3 in dimethyl formamide using [Hmim]BF4− as a catalyst (Method C)
EADBO (93.75 g, 750 mmol epoxy), NaN3 (73.9 g, 1.125 mmol), [Hmim]BF4− (14.16 g, 15.1 wt%) and dimethyl formamide (DMF, 181 mL) were added into a 500 mL round-bottom flask. A condenser was attached to the flask, and the entire system was placed on a magnetic stirrer. The reaction was allowed to proceed at 95°C for 60 h. After this, the reaction mixture was cooled to room temperature, dissolved in 800 mL ethyl acetate and washed five times successively with saturated sodium chloride solution and distilled water in a separating funnel, to remove the catalyst and the unreacted azide. The ethyl acetate layer was dried over anhydrous Na2SO4, and the solvent was removed using a rotary evaporator. The product showed the following IR frequencies (film cm−1): 3200–3450 (CH OH), 2100 (CH N3 ) and 1742 (COOCH2).
Results
Epoxidation
Large scale conversion of vegetable oils to their corresponding epoxides is usually carried out using hydrogen peroxide and formic acid or acetic acid ( Citation16–19). These conversions are highly exothermic, and therefore, pose inherent safety concerns. Corrosion caused by the acidic nature of percarboxylic acids and the acids formed by the reduction of peracids during the epoxidation is also a matter of concern. The instant separation of the epoxides from the acids, so as to avoid the oxirane ring opening, is also challenging. Oxirane ring opening may result in low oxirane values, which is not desirable. Nowadays, oil industries strive to produce epoxidized oil with the highest possible level of oxirane. We have already reported ( Citation14) that Adenopus breviflorus benth oil consists of 61.3% linoleic acid, 13.8% oleic acid, 14.1% stearic acid, and 10.8% palmitic acid. Peracid epoxidation of such highly unsaturated oil is inconvenient as the reactions are usually very exothermic and therefore carry along with them inherent concerns about safety ( Citation20). Herein, we used Curci’s biphasic method for the epoxidation of Adenopus breviflorus benth oil which has high linoleic acid content. In the Curci’s method, dioxirane which has been proved to be a very powerful and versatile oxygen atom transfer reagent is generated in-situ and used directly ( Citation20). This method employs ethyl methyl ketone as the solvent and ethyl methyl dioxirane as the oxidant. The epoxy ring formation was confirmed by FTIR and 1H NMR spectroscopy. The FTIR spectra showed a characteristic C–O–C oxirane stretching band at 824 cm−1. There were no bands around 1650 and 3008 cm−1 corresponding to C=C absorption, indicating the absence of vinyl unsaturation. However, for both ADBO and EADBO, carbonyl absorption at 1742 cm−1, characteristic triglyceride ester triplet at 1240, 1158, and 1106 cm−1, and a sharp absorption at 724 cm−1 corresponding to the C–H bending in saturated carbon chains more than seven carbon atoms long, could be observed. The superiority of the dioxirane epoxidation of triglyceride over the peracid epoxidation was evident from the absence of the hydroxyl band in the IR spectra of the EADBO. Peracid epoxidation results in oxirane ring opening to form the corresponding diols ( Citation20). In the 1H NMR spectrum of EADBO (), the signals between 2.8–3.1 ppm, which integrates to 11.34 hydrogens, correspond to the epoxy rings. The percentage of epoxidation was calculated to be 94.5% with respect to the vinyl signals between 5.3–5.5 ppm in the 1H NMR spectrum of ADBO. These signals integrated into 12 hydrogens. Both the regions were integrated using the methane proton of glycerol at 5.23 as basis.
Azidation
The conversion of the epoxide to azidohydrin with NaN3 can be attained using NH4Cl as a catalyst ( Citation21), or [Hmim]BF4− as a catalyst in water ( Citation3), or [Hmim]BF4− as a catalyst in DMF ( Citation12). Although many groups have reported the successful grafting of the azide moiety at the unsaturated sites in plant oils, they did not particularly pay attention to the retention of the triglyceride backbone, which is very important for the biodegradability of the resulting chemical derivatives. This factor is important because environmental friendliness, and hence, biodegradability, is one of the major reasons for the increasing shift toward demand for oil-based products.
In all the three methods (Method A, Method B, and Method C), EADBO undergoes nucleophilic addition with NaN3 to yield the corresponding azidohydrin derivative. The FTIR spectra of the azidated oils show the azide stretching band at 2100 cm−1 and a broad band between 3200 and 3500 cm−1 for the hydroxyl groups formed during the azidation. However, the product formed in Method A showed another band around 1670 cm−1, which could be attributed to the carbonyl of an acyl azido group. Thus, it is evident that when the reaction is carried out using Method A, NaN3 reacts simultaneously with the ester bond of the triglyceride and the oxirane ring (Scheme 1). The reaction was monitored over time, and it was found that the acidolysis was incomplete even after 3 days, as the ester band at around 1740 cm−1 was still observed.
Scheme 1. Reaction of epoxidised Adenopus breviflorus benth oil with NaN3 and NH4Cl + DMF (Method A), (ii) ([Hmim]BF4−) in H2O (Method B) and (iii) ([Hmim]BF4−) in DMF (Method C).
![Scheme 1. Reaction of epoxidised Adenopus breviflorus benth oil with NaN3 and NH4Cl + DMF (Method A), (ii) ([Hmim]BF4−) in H2O (Method B) and (iii) ([Hmim]BF4−) in DMF (Method C).](/cms/asset/bd755f8e-7386-4926-bb8f-9a219aeab8d1/tgcl_a_1737251_f0007_ob.jpg)
The 1H NMR spectra of the products obtained by the different methods are presented in . Generally, azidohydrin signals appear as a complex pattern between 3.1 and 4.0 ppm, wherein, the signals between 3.16 to 3.25 ppm and 3.4 to 4.0 ppm correspond to (CH-N3) and (CH-OH). The signals from the product AZOB (azidated oil by method B) obtained when EADBO was reacted with NaN3 without any catalyst revealed that only a marginal conversion to the azide took place, as the epoxy peaks in the range 2.8– 3.1 ppm remained substantially at 84.8% (). However, the spectra of the products obtained using the other methods did not show any signals for epoxy but showed signals corresponding to the azidohydrin moiety. A comparison of the integral of the azide signals at around 3.18 ppm and that of the epoxy signals in the spectrum of EADBO was used to estimate the percentage of azidation in the products obtained by the different methods. While azidation of EADBO with NaN3 in the presence of [Hmim]BF4– in water resulted in 25.4% azidation, AZOC (azidated oil by method C), that in the presence of [Hmim]BF4- in DMF resulted in 90.5% azidation, AZOD (azidated oil by method D). The azidation of EADBO with NaN3 and ammonium chloride also resulted in only 27.9% azidation, AZOE (azidated oil by method E).
Figure 2. Proton NMR of products of EPKCO and NaN3 in water (AZOB), EPKCO and NaN3 in water and ionic liquid (AZOC), EPKCO and NaN3 in DMF and ionic liquid (AZOD) and EPKCO and NaN3 and NH4Cl (AZOE).
The 13C NMR spectra of the products obtained by the different methods are shown in . The epoxy signals occurring in the region 54–58 ppm in the EADBO (W) were not present in the other spectra (X, Y, and Z), indicating their reaction with NaN3 to yield the corresponding azidohydrin containing carbinol (signals around 70–73 ppm) and the azide-bearing carbon (signals from 65 to 67 ppm). Of particular interest in the 13C NMR spectra are the signals at 62.1 and 68.9 ppm that correspond to the glycerol moiety. These signals would be absent if the triglyceride chain is totally hydrolyzed. In this regard, it was found that the product obtained from the reaction of EADBO with NaN3 in DMF and [Hmim]BF4– did not have the triglyceride chain (, Y). The high percentage of azidation observed in this product could be partly explained by the degradation of the glycerol moiety. The absence of the glycerol moiety eliminated any steric hindrances, and the reaction of the epoxies with NaN3 to produce azidohydrin could proceed easily. Thus, the product by Method C would have poor degradability and therefore, is not an environmentally friendly product. The reaction of EADBO with NaN3 in DMF or NH4Cl caused simultaneous opening of the oxirane rings as well as degradation of the ester bond of the triglyceride. This can be confirmed on the basis of the fact that the spectrum (, Z) contained a signal at 162.6 ppm, ascribable to the –CON3 moiety that is introduced after the reaction of azide with the ester of triglyceride. The signals at 62.1 and 68.9 ppm due to glycerol in the same spectra suggest that the acidolysis of the triglyceride chain was incomplete, and some of the triglyceride backbone was still retained at the end of the reaction. The product obtained by Method A is, therefore, be more environmentally friendlier than that obtained by Method C. , X, shows the 13C NMR spectrum of EADBO after the reaction with NaN3 in water in the presence of [Hmim]BF4−. The signal from the azide-bearing carbon appears at 67 ppm, while those from carbinol appear at 70 and 73 ppm. The signals from the glycerol moiety appear at 62.1 and 68.9 ppm; No signals appear around 162 ppm, indicating the absence of hydrolysis of the triglyceride chain. Therefore, in terms of biodegradability and environmental friendliness, the product obtained by Method B is the best among the three methods investigated. Based on the information obtained from the 13C NMR spectra, the reaction in Method B can be proposed to proceed via the pathway shown in Scheme 1. The product obtained from Method B, however, has the lowest percentage of azidation among the three methods investigated. This is expected because the steric hindrance imparted by the triglyceride backbone prevents the easy access of NaN3 to the internal epoxides.
Figure 3. 13C NMR spectra (40–180 ppm) of EADBO (W), products of EADBO and sodium azide in water and ionic liquid catalyst (X), products of EADBO and sodium azide in DMF and ionic liquid catalyst (Y) and products of EADBO and sodium azide in DMF and NH4Cl catalyst (Z).
The oxirane value, nitrogen content, molecular weights obtained from GPC, and some other characteristics are given in . The oxirane value, a measure of the epoxy groups in oils, was 4.2 for the EADBO but less than 1.0 for the azidated oil. The reaction of NaN3 with the epoxides on the fatty acid chain of the oil was reflected by the molecular weights determined by GPC (). These were higher for the azidated oil (Mw = 1757–1881g/mol) as compared with those of the corresponding epoxidized oil (Mw = 1473 g/mol). The polydispersity index (PDI) of the azidated oil ranged from 1.06–2.01. The nitrogen content in the azidated oil was determined by the elemental analyzer, and the value was in good agreement that obtained by the NMR measurements. The nitrogen content increased in the order AZOM < AZOE < AZOD. The color of the azidated oils varied from light brown to dark brown. The number of azide functionality (Nf) per triglyceride unit was calculated using the equation Nf = (Mn × %Nitrogen/42) × 100, as reported by Hong et al. ( Citation13), where Mn is the number-average molecular weight obtained from GPC, 42 is the molecular mass of the azide group and %nitrogen is the nitrogen content obtained from the elemental analysis. It is evident from that Nf increased in the order
Table 1. Some characteristics of azidated oils derived from EADBO.
AZOM < AZOE < AZOD, as expected.
The DSC melting and crystallization curves of the ordinary, epoxidized, and azidated oils are presented in and . The oils show one to three melting peaks between −40°C and 50°C and one crystallization peak between −20°C and −7°C. The different peaks for the different oils can be ascribed to the different crystalline structures of the oils.
Conclusion
A water-based reaction employing an ionic liquid, [Hmim]BF4−, as a catalyst was found to be the most effective in the NaN3-mediated azidation of the epoxides of a plant oil, ADBO. The triglyceride structure of the starting oil was fully retained in the product, thereby rendering the product biodegradable and hence, environmentally friendly. The light brown azidated ADBO had a molecular weight of 1835 g/mol, as determined from GPC, and PDI of 1.05. The nitrogen content, as obtained by the elemental analysis, was 11.18% while the number of azide functionality was 4.60. Azido compounds generally prepared from petrochemicals may now be prepared from plant oil source using method B. The azido compound prepared is an appealing straight forward access to preparation of biodegradable vicinal hydroxyl triglyceride which is very versatile in the surfactant industries
Disclosure statement
No potential conflict of interest was reported by the author(s).
Notes on contributors
T. Akintayo Emmanuel is a professor in the Chemistry department of Ekiti State University, Ado-Ekiti, Ekiti State, Nigeria.
O. Akintayo Cecilia is a professor in the Industrial Chemistry department of the Federal University Oye, Oye-Ekiti, Ekiti State, Nigeria.
I.O. Oluwaleye is a professor in the Mechanical Engineering department of Ekiti State University, Ado-Ekiti, Ekiti State, Nigeria.
O. Ajaja is a professor in the Mechanical Engineering department of Ekiti State University, Ado-Ekiti, Ekiti State, Nigeria.
S. Beuermann is a professor in the Technische Universitat Clausthal, Clausthal - Zellerfeld, Germany.
Additional information
Funding
References
- Anders, S.C.; Jenny, L.Y.; Allan, G.G.; Sten, S.; Hofvander, P. Eur. J. Lipid Sci. Technol. 2011, 113, 812–831. doi: 10.1002/ejlt.201100032
- Stefan, B.; Carmen, G.; Kerstin, K.; Zimmermann, V. Angew. Chem. Int. Ed. 2005, 44, 5188–5240. doi: 10.1002/anie.200400657
- Biswas, A.; Sharma, B.K.; Willett, J.L.; Advaryu, A.; Erhan, S.Z.; Cheng, H.N. J. Agric. Food Chem. 2008, 56, 5611–5616. doi: 10.1021/jf800123t
- Saito, S.; Takahashi, N.; Ishikama, T.; Moriwake, T. Tetrahed. Lett. 1991, 32 (59), 667–670. doi: 10.1016/S0040-4039(00)74855-6
- Metzger, J.O.; Furmeier, S. Eur. J. Org. Chem.. 1999, 4, 661–664. doi: 10.1002/(SICI)1099-0690(199903)1999:3<661::AID-EJOC661>3.0.CO;2-V
- Sabitha, G.; Babu, R.S.; Kumar, M.J.; Yadav, J.S. Synthesis 2002, 15, 2254–2258. doi: 10.1055/s-2002-34848
- Wu, K.; Yujie, L.; Ning, J. Molecules 2016, 21 (3), 352. doi: 10.3390/molecules21030352
- Goswamiand, M.; de Bruin, B. Eur. J. Org. Chem.. 2017, 8, 1152–1176. doi: 10.1002/ejoc.201601390
- Lie, K.; Jie, M.S.F.; Lao, H.B. Chem. Phys. Lipids 1987, 45, 65–74. doi: 10.1016/0009-3084(87)90040-5
- Lie, K.; Jie, M.S.F.; Alamu, M.S. Chem. Phys. Lipids 2001, 111, 29–35. doi: 10.1016/S0009-3084(01)00140-2
- Hong, J.; Dragana, R.; Djavan, H.; Xianei, W.; Zoran, S.P. Eur. J. Lipid Sci. Technol. 2015, 117, 266–270. doi: 10.1002/ejlt.201400461
- Guo, G.; Jian, S.; Chen, Z.; Yun, L.; Cheng-Mei, L. Green Chem. 2016, 18, 1278. doi: 10.1039/C5GC01919B
- Hong, J.; Luo, Q.; Wan, X.; Petrovic, Z.S.; Shah, B.K. Biomacromolecules 2012, 13, 261–266. doi: 10.1021/bm201554x
- Akintayo, E.T.; Bayer, E. Biores. Technol. 2002, 85, 95. doi: 10.1016/S0960-8524(02)00073-1
- Hua-Ping, Z.; Fan, Y.; Jie, T.; Ming-Yuan, H. Green Chem. 2003, 5, 38–39. doi: 10.1039/b209248b
- Gunstone, F.D.; Hamilton, R.J., Eds.; Oleochemical Manufacture and Applications; Sheffield Academic Press: Sheffield, 2001, 14–24.
- Petrovi, Z.S.; Zlatani, A.; Lava, C.C.; Sinadinovi- Fiser, S. Eur. J. Lipid Sci. Technol. 2002, 104, 293–299. doi: 10.1002/1438-9312(200205)104:5<293::AID-EJLT293>3.0.CO;2-W
- Dinda, A.; Patwarthan, A.V.; Goud, V.V.; Pradhan, N.N. Biores. Technol. 2008, 99, 3737–3744. doi: 10.1016/j.biortech.2007.07.015
- Milchert, E.; Smagowicz, A. J. Am. Oil Chem. Soc. 2009, 86, 1227–1233. doi: 10.1007/s11746-009-1455-7
- Curci, R.; Fiorentino, M.; Troisi, L. J. Org. Chem. 1980, 45, 4758. doi: 10.1021/jo01311a040
- Akintayo, E.T.; Ziegler, T.; Akintayo, C.O. Ind. Crops Prod. 2006, 24, 166–170. doi: 10.1016/j.indcrop.2006.03.008