ABSTRACT
The oil and gas industry is often challenged with serious problems like high cost of oil field chemicals and environmental toxicity issues of commonly used synthetic oil field chemicals, and this has dragged the attention of researchers to the search for more cost effective and environmentally friendly oil field chemicals. Oil field chemicals formulated from various renewable sources (such as plant extracts) have been recognized as an alternative with great environmental advantages, cost advantage, and availability compared to their synthetic counterparts. Cashew nut shell liquid (CNSL), a byproduct of the cashew industry, stands out as a unique renewable starting material amongst others due to its peculiar structural feature, low cost, and availability. It consists of naturally occurring substituted phenolic compounds that can participate in diverse reactions for the manufacture of numerous useful products. A large number of chemicals and products have been developed starting from CNSL by taking advantage of the reactive sites namely phenolic hydroxyl, aromatic ring, the acid functionality, and unsaturation(s) in the C15 alkyl side chain. This update gives highlights on the composition, extraction, isolation, and reactivity of CNSL. It also focuses on the oil field application of CNSL and its derivatives.
GRAPHICAL ABSTRACT
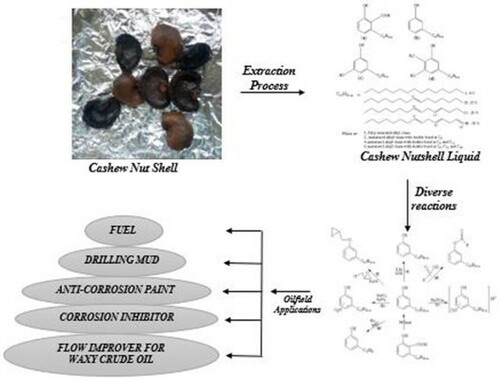
Disclosure statement
No potential conflict of interest was reported by the author(s).