ABSTRACT
Utilization of waste-derived materials for industrially pertinent chemical transformations is highly challenging and offers sustainable solution to waste management. Despite several difficulties in the manufacture of aryl iodides (AIs), which are the fundamental substrates in organic synthesis, we report here, an added oxidant/metal/catalyst/additive and problematic solvent-free versatile and straightforward protocol for the synthesis of AIs using molecular iodine (I2) in water extract of pomegranate ash (WEPA). These transformations were performed at room temperature (rt) and the reactions takes place in 5–20 min to give 83–99% yields of AIs. WEPA was characterized by using XPS, EDX, XRF, XRD, and FTIR analysis and a plausible mechanism has been established based on these analyses along with some control experiments. Further, the products were purified by recrystallization technique. This work with intrinsic sustainability, high substrate feasibility, utilization of biorenewable catalystytic media, ease of execution and separation of products, and added oxidant/catalyst/metal and additive-free conditions may become one among the forefront sustainable procedures in making AIs. The use of waste-derived WEPA as catalystytic media for this iodination is the novelty of this method and may be used these AIs for further in-process transformations (e.g. cross-couplings, nucleophilic reactions, etc.) to access fine chemicals.
GRAPHICAL ABSTRACT
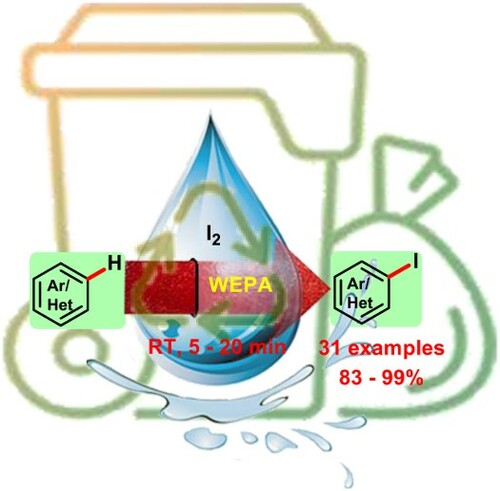
1. Introduction
The population of the world was predicted as 9.7 and 11.0 billion people by 2050 and 2100 from present 7.7 billion (Citation1,Citation2). The growing population owing to the utilization of food, energy, feed, fiber, etc. can create enormous amount of waste. According to the World Bank report ∼2017 metric tons of waste have been produced globally in 2018 and may reach ∼2586 and ∼3401 metric tons by 2030 and 2050 (Citation2,Citation3). The content of organic matter of this waste is 40–50% and its disposal in landfill has several environmental problems like liberation of greenhouse gases (GHGs), emanation of odor, contamination of surface as well as ground water, transmissions via vector such as birds, mammals and insects (Citation2,Citation4). Hence, there is a large demand in the conversion of these wastes by physical or chemical means into useful products including biofuels, animal feed and feedstocks of biochemical and chemical relevance (Citation2,Citation4,Citation5). Furthermore, the diminishing delivery associated with the increasing demand for fossil-based depleting substances impetus in pursuit for biorenewable sources of chemical substances (Citation6–10). In this connection the utilization of waste-derived products as useful feedstocks, auxiliaries and solvents is the challenging, sustainable, highly economic, and convenient processes in chemical synthesis.
Recently, the extracts of ashes of biorenewable wastes are considered as emerging green media/catalysts in the evolution of significant synthetic transformations such as Suzuki–Miyaura, Ullmann, Sonogashira, Henry, Dakin, Knoevenagel, and Michael addition reactions (Citation2). These extracts are also explored in the construction of bisenols, coumarins, C–P bonds, allylsulfides, organic disulfides, and peptide bonds (Citation2). The ashes of biorenewable wastes are also reported as sustainable catalysts in the production of biodiesel (Citation2,Citation11) and chiral tert-butylsulfinylaldimines (Citation12). The sturdy attributes of these media/catalysts include the strategic ejection of commercial and depleting resources-based bases and solvents, effective utilization of waste as biorenewable resources, and sustainable media – catalyst system. The choice of the water as solvent is a strategic method to minimize the use of volatile organic compounds (VOCs), which are the major culprits of environmental pollution and volatile solvents occupy about 85% of chemicals utilized by the pharma industry can merely be recovered at the rate, 50–80% (Citation13).
Nature, especially marine organism has evidenced a tremendous number of organic iodine compounds (Citation14,Citation15). Most of these natural iodides and their synthetic analogues were turned out to be biologically indispensable (Citation15,Citation16). Medicinal applications of radioiodinated organic compounds are also familiar in radio imaging towards the assessment and treatment of diseases like thyroid disorders (Citation17), cancers, and neurological defects (Citation18). Additionally, organic halides are the highly reactive and fundamental substrates in numerous organic transformations including C–C and C-heteroatom couplings, organometallations, hypervalent iodine syntheses, and free-radical initiations (Citation19,Citation20). Despite the most reactivity of organic iodides, particularly, aryl iodides (AIs) (caused by the easily frangible C–I bond over C–Br and C–Cl bonds of aryl bromides and chlorides), the commercially less availability and relatively high cost of AIs restricted their widespread aptness in chemical synthesis (Citation19,Citation21). The commercial scarcity of AIs is due to the limited methods and reagents that exist for their preparation than aryl bromides and aryl chlorides, perhaps, making the C–I bond is complicated than C–Br and C–Cl. Molecular iodine (Citation22,Citation23), N-iodosuccinimide (NIS) (Citation24), ICl (Citation25), dichloroiodates (Citation26), KI (Citation27), and polyhalogenated reagents (Citation28,Citation29) are frequently used for AIs synthesis. Although the substitution reactions of aryl diazonium salts, arylboronic acids, and aryl bromides are the other infrequently used methods to access AIs, these require metals or harsh conditions (Citation24) are less atom economic in the green chemistry standpoint.
Besides several environmental benefits, iodine is the simplest, inexpensive, and readily available reagent, but, the general low reactivity of I2 in aromatic electrophilic substitutions is very unfortunate and hence, the use of an oxidant is obligatory (Citation30). In this vein, I2–H5PV2Mo10O40–O2–CH3CN (Citation31), I2–poly-vinylpyrrolidone supported H2O2–H3PW12O40–DCM (Citation32), I2–Fe(NO3)3-1.5 N2O4–charcoal–DCM (Citation33), I2–NO2–CH3CN (Citation22), I2–H2O2–H2O (Citation34), I2–Al2O3–benzene (Citation35), I2–Ag2SO4–EtOH (Citation36), I2–PhI(OCOCF3)2–pyridine–DCM (Citation37), I2–SiO2·Fe(NO3)3·9H2O–DCM (Citation38), I2–(Bu4N)2S2O8–CH3CN (Citation39), I2–(NH4)2Ce(NO3)6 (Citation40), I2–Pb(OAc)4–AcOH-Ac2O (Citation41), I2–NaNO2–HCl–trifluoroethanol (Citation42), IBX–I2–TFA–DMSO/TFA/CH3CN/AcOH (Citation43), I2–AgOTf/AgOAc/AgOMs/AgOTs–CH3CN/DCM (Citation44), I2–NaNO2–air–silica-supported H2SO4–CH3CN (Citation45), and (PhIO)n–NH4I–K3PO4–MeOH (Citation23) systems are noticed as synthetically useful for AIs access. Some of the recent methods in aryl iodinations using I2 as a source of iodine are shown in Scheme 1. In addition to the obligations of oxidant and (or) catalyst the reported procedures necessitate hazardous/problematic solvents, additives, high temperature, or large reaction times, and thus it is necessary to develop new protocols to access the AIs at mild and environment friendly conditions. Moreover, the iodination with molecular iodine reveals the natural process of making organic iodides (Citation46).
Therefore, we directed to develop an economical and green protocol for easy synthesis of AIs and found WEPA is advantageous as bioresource-based catalyst and media in this pursuit. In continuation to our research on WEPA (Citation12,Citation47–52), we disclose here an added catalyst, oxidant, volatile/hazardous solvent, metal and additive-free, versatile protocol for the preparation of AIs in WEPA. Furthermore, in pursuance of the green chemistry philosophy, the use of aqueous media and renewable substances greatly reduce the ecological impacts caused by the application of problematic solvents and depleting materials (usually VOCs) (Citation12,Citation52–54). The application of biorenewable and waste-derived WEPA for a very quick synthesis of (hetero)aryl iodides by avoiding non-renewable resources-based oxidants, catalysts, promoters, and solvents is the novelty and the innovative development of this work.
2. Materials and methods
2.1. General information
Aryl/heteroaryl substrates used in the current conversion are obtained from AVRA synthesis, Alfa Aesar, Sigma-Aldrich, Spectrochem, and Sd-fine were employed as they obtained. Silica gel-based thin layer chromatography (TLC) (Merck) was used to predict the reaction progress using UV light visualization. The (hetero)aryl iodides are settled their structures using 1H & 13C NMR and MS spectral data. 1H & 13C NMR spectra were obtained using Bruker Avance (Billerica, MA, USA) 400/100 MHz spectrometer. X-ray fluorescence (XRF) analysis was performed using wavelength-dispersive XRF spectrometry (Philips PW-2440 Magix-Pro® model, Eindhoven, The Netherlands). Scanning electron microscopy (SEM) and energy dispersive X-ray (EDX) data were obtained using JEOL JST-IT-500 (Tokyo, Japan) instrument. FTIR of WEPA has been measured using Bruker (Billerica, MA, USA) ALPHA-II ECO-ATR model compact FTIR spectrometer. The X-ray diffraction (XRD) patterns were recorded using Rigaku MiniFlex600 (Tokyo, Japan) instrument. The X-ray photoelectron spectra (XPS) were recorded using Thermo Scientific (USA) ESCALB 250 model instrument with the monochromatic Al Kα X-ray source.
2.2. Preparation of WEPA
WEPA was made adopting our recent publications (Citation12,Citation47–49). Pomegranate peels were obtained from a fresh pomegranates collection. Washed the peels with distilled water and dried under shade was made into small pieces. The pieces of peels were made to ash and 10 g of ash was macerated in 100 mL distilled water, stirred at rt for 2 h has been filtered to give a light pale yellow colored extract called WEPA. WEPA was characterized for the available chemical constituents using its XPS, EDX, XRF, XRD, and FTIR data () (please see the discussion in Section 3.1).
2.3. General procedure for the synthesis of AIs
WEPA (1.5 mL), I2 (1.05 mmol), and EtOH (0.2 mL) in a reaction flask were allowed to stir at rt for 5 min. Added all at once the aryl/heteroaryl substrate (1) (1 mmol) to a mixture containing 0.3 mL EtOH and 1.0 mL of WEPA and the resultant mixture was subjected for stirring at rt for appropriate time as indicated by TLC. Added 10 mL cold water and the precipitate obtained has been separated by filtration was washed using cold water. Pure products are obtained via recrystallization using water–ethanol mixture. The structures of products are detected using 1H & 13C NMR and Mass spectral data and the copies of 1H & 13C NMR spectra have been provided in supplementary material.
2.3.1. 4-Iodoaniline (2ab) (Citation24)
Dark brown liquid; Yield: 95%; 1H NMR (400 MHz, CDCl3): δ 7.40 (d, J = 8.8 Hz, 2H), 6.47 (d, J = 8.8 Hz, 2H), 3.02 (brs, 2H) ppm; 13C NMR (100 MHz, CDCl3): δ 146.0, 137.9, 117.3, 79.4 ppm; MS (EI): 220 (M + 1).
2.3.2. 4-Bromo-2-iodoaniline (2ag) (Citation55)
Pale red solid; Yield: 99%; 1H NMR (400 MHz, CDCl3): δ 7.66 (d, J = 2.3 Hz, 1H), 7.14 (dd, J = 2.3, 8.6 Hz, 1H), 6.54 (d, J = 8.6 Hz, 1H), 4.03 (brs, 2H) ppm; 13C NMR (100 MHz, CDCl3): δ 145.9, 140.3, 132.0, 115.5, 109.8, 84.0 ppm; MS (EI): 298 (M + 1).
2.3.3. 2-Chloro-4-iodoaniline (2ah) (Citation24)
Colorless solid; Yield: 95%; 1H NMR (400 MHz, CDCl3): δ 7.43 (d, J = 2.0 Hz, 1H), 7.21 (dd, J = 2.0, 8.6 Hz, 1H), 6.42 (d, J = 8.6 Hz, 1H), 3.96 (s, 2H) ppm; 13C NMR (100 MHz, CDCl3): δ 142.6, 137.1, 136.3, 120.2, 117.4, 77.9 ppm; MS (EI): 254 (M + 1).
2.3.4. 4-Amino-3-iodobiphenyl (2ak) (Citation55)
Pale yellow solid; Yield: 91%; 1H NMR (400 MHz, CDCl3): δ 7.89 (d, J = 1.8 Hz, 1H), 7.49 (d, J = 7.8 Hz, 2H), 7.43-7.25 (m, 3H), 7.31-7.23 (m, 1H), 6.80 (d, J = 8.3 Hz, 1H), 4.14 (brs, 2H) ppm; 13C NMR (100 MHz, CDCl3): δ 146.0, 139.6, 137.3, 133.2, 128.7, 128.1, 126.7, 126.4, 114.7, 84.5 ppm; MS (EI): 296 (M + 1).
2.3.5. 4-Fluoro-2-iodoaniline (2an) (Citation56)
Brown solid; Yield: 94%; 1H NMR (400 MHz, CDCl3): δ 7.31 (d, J = 2.9, 8.1 Hz, 1H), 6.86-6.79 (m, 1H), 6.57 (dd, J = 4.9, 8.9 Hz, 1H), 3.87 (brs, 2H) ppm; 13C NMR (100 MHz, CDCl3): δ 154.7 (d, J = 240.2 Hz), 143.1 (d, J = 1.3 Hz), 124.5 (d, J = 24.6 Hz), 115.8 (d, J = 22.0 Hz), 114.4 (d, J = 7.3 Hz), 82.6 (d, J = 8.4 Hz) ppm; MS (EI): 238 (M + 1).
2.3.6. 4-Iodoanisole (2ap) (Citation24)
Colorless solid; Yield: 98%; 1H NMR (400 MHz, CDCl3): δ 7.55 (d, J = 8.2 Hz, 2H), 6.68 (d, J = 8.2 Hz, 2H), 3.78 (s, 2H) ppm; 13C NMR (100 MHz, CDCl3): δ 159.4, 138.1, 116.3, 82.6, 55.3 ppm; MS (EI): 235 (M + 1).
2.3.7. 4-Iodophenol (2ar) (Citation24)
Colorless semi solid; Yield: 94%; 1H NMR (400 MHz, CDCl3): δ 7.51 (d, J = 8.9 Hz, 2H), 6.62 (d, J = 8.9 Hz, 2H), 4.99 (brs, 1H) ppm; 13C NMR (100 MHz, CDCl3): δ 155.2, 138.4, 117.8, 82.7 ppm; MS (EI): 221 (M + 1).
2.3.8. 1-Iodo-2-naphthol (2at) (Citation57)
Pale brown solid; Yield: 99%; 1H NMR (400 MHz, CDCl3): δ 7.93 (d, J = 8.4 Hz, 1H), 7.78-7.71 (m, 2H), 7.55 (t, J = 8.3 Hz, 1H), 7.38 (t, J = 8.3 Hz, 1H), 7.26 (d, J = 8.0 Hz, 1H), 6.79 (brs, 1H) ppm; MS (EI): 271 (M + 1).
2.3.9. 2-Bromo-4-iodophenol (2au) (Citation58)
Pale yellow solid; Yield: 98%; 1H NMR (400 MHz, CDCl3): δ 7.68 (d, J = 2.0 Hz, 1H), 7.42 (dd, J = 2.0, 8.6 Hz, 1H), 6.71 (d, J = 8.6 Hz, 1H), 5.52 (brs, 1H) ppm; 13C NMR (100 MHz, CDCl3): δ 152.3, 139.6, 138.0, 118.1, 111.3, 82.0 ppm; MS (EI): 299 (M + 1).
2.3.10. 2-Hydroxy-5-iodoacetophenone (2av) (Citation59)
Pale yellow solid; Yield: 90%; 1H NMR (400 MHz, CDCl3): δ 12.18 (brs, 1H), 8.01 (d, J = 2.3 Hz, 1H), 7.71 (dd, J = 2.3, 8.9 Hz, 1H), 6.78 (d, J = 8.9 Hz, 1H), 2.62 (s, 3H) ppm; 13C NMR (100 MHz, CDCl3): δ 203.4, 162.0, 144.7, 139.0, 121.8, 120.9, 79.6, 26.7 ppm; MS (EI): 263 (M + 1).
2.3.11. 5-Iodoacetovanillone (2ax) (Citation60)
Pale yellow solid; Yield: 93%; 1H NMR (400 MHz, CDCl3): δ 7.91 (d, J = 2.0 Hz, 1H), 7.48 (d, J = 2.0 Hz, 1H), 6.63 (brs, 1H), 3.94 (s, 3H), 2.54 (s, 3H) ppm; MS (EI): 293 (M + 1).
2.3.12. 5-Iodovanillin (2ay) (Citation61)
Colorless solid; Yield: 96%; 1H NMR (400 MHz, CDCl3): δ 9.77 (s, 1H), 7.82 (d, J = 1.7 Hz, 1H), 7.38 (d, J = 1.7 Hz, 1H), 6.68 (brs, 1H), 3.98 (s, 3H) ppm; 13C NMR (100 MHz, CDCl3): δ 189.5, 151.4, 146.5, 136.2, 131.0, 108.6, 80.4, 56.5 ppm; MS (EI): 279 (M + 1).
2.3.13. 2,4-Dihydroxy-3-iodoacetophenone (2az) (Citation62)
Yellow solid; Yield: 93%; 1H NMR (400 MHz, CDCl3): δ 13.76 (brs, 1H), 7.66 (d, J = 8.7 Hz, 1H), 6.62 (d, J = 8.7 Hz, 1H); 6.18 (brs, 1H), 2.61 (s, 3H) ppm; 13C NMR (100 MHz, CDCl3): δ 202.3, 163.5, 161.7, 132.6, 114.0, 106.8, 26.0 ppm; MS (EI): 279 (M + 1).
2.3.14. 4-Iodopyrazole (2ba) (Citation63)
Colorless solid; Yield: 95%; 1H NMR (400 MHz, CDCl3): δ 10.49 (brs, 1H), 7.60 (s, 2H) ppm; 13C NMR (100 MHz, CDCl3): δ 138.8, 56.5 ppm; MS (EI): 195 (M + 1).
2.3.15. 2-Amino-5-iodopyridine (2bb) (Citation64)
Pale yellow solid; Yield: 96%; 1H NMR (400 MHz, CDCl3): δ 8.22 (d, J = 1.7 Hz, 1H), 7.63 (dd, J = 1.7, 8.7 Hz, 1H), 6.36 (d, J = 8.7 Hz, 1H), 4.52 (brs, 2H) ppm; 13C NMR (100 MHz, CDCl3): δ 157.2, 153.6, 145.4, 110.9, 77.8 ppm; MS (EI): 221 (M + 1).
2.3.16. 2-Amino-5-bromo-3-iodopyridine (2bd) (Citation65)
Pale yellow solid; Yield: 94%; 1H NMR (400 MHz, CDCl3): δ 8.05 (d, J = 2.0 Hz, 1H), 7.95 (d, J = 2.0 Hz, 1H), 5.07 (brs, 2H) ppm; 13C NMR (100 MHz, CDCl3): δ 156.4, 148.4, 148.1, 107.2, 77.6 ppm; MS (EI): 299 (M + 1).
3. Results and discussion
3.1. Characterization of WEPA
The XPS (Citation48) (see supplementary material) and EDX analysis ((d)) indicates the presence of large quantities of K and O in WEPA. XRF analysis of dried WEPA showed () the major constituents as K2O (66.513%), Cl (19.393%), SO3 (3.892%), Na2O (2.197%), MgO (1.895%), SiO2 (1.926%), and CaO (2.112%) along with other minor metallic and non-metallic components and the quantities of constituents are found to be similar with our recent XRF analysis () (Citation12,Citation51) provides crucial information on the qualities of the chemical constituents of WEPA. The XRF data resembles the XPS data of WEPA for the elements present in it (Citation48). Further, the EDX data of freshly prepared WEPA ((d)) was also found to show good agreement with our reported EDX data at different time periods (Citation12,Citation48,Citation49) and this can indicate the chemical constituents (about both the quantity and quality) are constant in WEPA. The SEM images ((a–c)) of pale yellow powder received after evaporation of WEPA have been showing a uniform, smooth, and well-defined plate-like morphology of its surface (Citation12,Citation66,Citation67).
Table 1. Comparison of XRF analysis of WEPA used in present investigation with our recent reportsTable Footnotea.
The XRD analysis () indicates the presence of K, O, Mg, Cl, Ca, and C in WEPA. The appearance of peaks in XRD at 2θ = 25.69°, 28.39°, 30.21°, 32.21°, 36.41°, 40.55°, and 50.21o signifies the presence of K2O (Citation11,Citation68,Citation69). Two peaks at 2θ = 37.79° and 66.41° indicate CaO (Citation11,Citation70). XRD peaks at 42.95° and 73.65° represents MgO (Citation71) and two peaks at 2θ = 12.77° and 46.05° correspond to Na2O (Citation72,Citation73). The peaks at 28.39° and 39.11° represents KCl (Citation74) and two peaks at 2θ = 29.69° and 34.19o represents K2CO3 (Citation68) in WEPA.
The peaks at 1616, 1450, and 1365 cm–1 in the FTIR spectrum of WEPA () represents C = O groups (Citation11,Citation75). The peaks at 1130, 1060, and 817 cm–1 represent the K–O bond (Citation11,Citation55). The peaks at 1573, 701, and 609 cm–1 indicate Ca–O bond asymmetric and symmetric stretching (Citation11,Citation75). The absorption at 879 cm−1 corresponds to Mg–O–Mg (Citation11,Citation75). The shoulder peaks around 2200 cm–1 represent adsorbed CO2 on metal ions surface (Citation11,Citation75). The FTIR and XRD analysis of WEPA used for this investigation also resembles the XRF, EDX, and XPS analysis and also with the reported EDX, XRD, and FTIR data (Citation12,Citation48,Citation49). Hence, the comparable results at different time intervals using a variety of analytical methods may reveal the presence of chemical constituents is constant in WEPA and are indicated by its XRF data. Further, the titration of WEPA with oxalic acid was indicated the ∼0.09 N base is present in WEPA.
3.2. Investigation of WEPA for iodination of (hetero)arenes
We started the present quest using 2-bromoaniline (1aa) (1 mmol), WEPA (1.5 mL), and 0.55 mmol of I2, and was identified the creation of 29% of 2-bromo-4-iodoaniline (2aa) in 20 min at rt (, entry 1). The increase of I2 to 1.05 mmol significantly increased the yield of 2aa to 61% and further increase of I2 to 1.55 mmol showed no improvement (, entries, 2 & 3). The increase of the amount of WEPA to 2.0, 2.5, and 3.0 mL has produced the yields of 2aa as 67%, 75%, and 76% in 20, 10, and 10 min using 1.05 mmol of I2 (, entries, 4–6). These results indicated that this iodination requires 1.05 mmol of I2 and 2.5 mL WEPA. At this stage the 0.5 mL addition of ethanol resulted in nearly quantitative yields (99%) of 2aa (, entry 7), it may be because of sufficient solubility of both the 1aa and I2. Further, no sole effect of EtOH was concluded by reacting 1aa and I2 in 3 mL ethanol (, entry 8).
Table 2. Optimization of reaction conditionsTable Footnotea.
With these optimized reaction criteria, we explored the potency of this method to a wide array of substrates and exemplified the results in . Anilines, phenols, and aryl methyl ethers with an array of functional groups were made into iodoaromatics under present conditions (, products, 2ab–2ba). Anilines with the substitution on aromatic ring or ‘N’ atom were found as best substrates because of the presence of most activating nitrogen towards electrophilic substitutions. In this regard, free amine, N,N-dialkyl and N-acetyl groups along with methyl, chloro, bromo, acetyl, phenyl, fluoro, and benzo substituents on aromatic systems were offered high to about quantitative yields (91–99%) of iodinated derivatives in short time (5–15 min) (, products, 2ab–2ao). Trace amounts of regioisomers were found in case of aniline (1ab) and 1-naphthylamine (1al). The I2–(Bu4N)2S2O8–CH3CN system (Citation39) was required large reaction time (24 h) to produce 4-N,N-dimethylamono-1-iodobenzene (2ac) in 88% from N,N-dimethylamonobenzene, however, this method produces 96% of 2ac from the same substrate in just 10 min ().
Table 3. Iodination of aromatics or heteroaromatics using I2 in WEPATable Footnotea.
Aryl methyl ethers such as anisole (1ap) and 6-bromo-2-methoxynaphthalene (1aq) have also given excellent yields (98% and 83%) of their iodinated analogues, 2ap and 2aq in 15 min. No trace of regioisomers was identified in these cases.
Phenols with electron releasing (bromo), neutral (hydrogen), and electron-withdrawing (benzo, acetyl, and formyl) groups were readily converted to iodinated products in 5–20 min in 92–99% yields (, products, 2ar–2az). Formation of negligible amounts of isomeric iodides was observed with phenol (1ar) and 1-naphthol (1as). The formation of 92% of 4-iodophenol (2ar) from 1ar using I2–(Bu4N)2S2O8–CH3CN system (Citation39) required 30 h, while the present methods are very advantageous to deliver the 94% of 2ar in 5 min. The substrates with mono electron-withdrawing group substituents, such as chlorobenzene, benzaldehyde, acetophenone, and nitrobenzene did not deliver iodination products under these mild conditions.
Finally, the heteroaromatics such as pyrazole (1ba), 2-aminopyridine (1bb), 2-amino-3-bromopyridine (1bc), 2-amino-5-bromopyridine (1bd), and 4-N,N-dimethylaminopyridine (1be) were also found to form the monoiodinated products (2ba–2be) with high rates (5–10 min) in excellent yields (94–99%) under current conditions.
In most of these reactions, the mono-iodination products are formed with high position selectivity and hence WEPA catalyzed oxidative iodination of (hetero)arenes are significantly regioselective. However, no selectivity over mono-iodination has resulted with I2/H2O2 (Citation34) and the formation of large quantities of regioisomers on certain substrates was reported with I2/Al2O3 (Citation35) and I2/(NH4)2Ce(NO3)6 (Citation40). Furthermore, the iodo(hetero)arenes are separated by simple crystallization technique by eliminating the routine extraction and column chromatography thereby avoiding the use of organic solvents (as extracting and eluting solvents) and other chemical substances. From the global sustainability point-of-view, the development of nature mimicking/nature derived systems for conducting the reactions at ambient conditions by avoiding/reducing the use of volatile organics is highly useful (Citation2,12,76–80). Hence, this protocol is highly beneficial for the synthesis (hetero)aryl iodides, since, it uses naturally derived material as catalyst and works at depleting source-based oxidant/solvent/additive-free ambient conditions.
3.3. Mechanistic features
Based on the XPS, EDX, FTIR, XRD, and XRF investigations, the dried WEPA consists of high amounts of K2O (∼66.5%) and KCl (∼19%) (Section 2.1). It is also known that the K2O is highly reactive with water to produce KOH (Citation12,Citation51,Citation56). Based on these facts the WEPA contains KOH and KCl in large (relative) quantities. Towards understanding the influence of these substances on the present conversion as well as the mechanism of present C–H iodination, some controlled experiments have been conducted (). Initially, the reaction of 2-bromoaniline (1aa) with I2 in the presence of K2O in a 2.5 mL and 0.5 mL mixture of water and EtOH was produced the desired product, 2-bromo-4-iodoaniline (2aa) with 73% yield in 10 min. Further, 1aa on reacting with I2 using KOH in water and ethanol mixture (2.5 mL & 0.5 mL) was also provided 2aa in 76% yield in 10 min. Surprisingly, the addition of KCl has improved the yields in both the cases to >95% in 10 min and it is well documented that the enhancement of the catalytic activity of bases in the presence of KCl (Citation55,Citation57,Citation58). This study supports the involvement of K2O (as KOH) and KCl of WEPA in the C–H iodination of (hetero)arenes and it may provide a large breadth of research directed to study most of the K2O or KOH influenced reactions by the addition of KCl.
From these evidences and some reported iodinations using base I2/Al2O3 (Citation35) or (and) I2/NaOH (Citation59) the possible iodination pathway of aromatics or heteroaromatics using I2 in WEPA has been sketched in Scheme 3. The oxides (MxOy; mostly K2O and some other minor oxides) of WEPA or base of WEPA (M + Bˉ; mostly KOH and some other minor hydroxides) are responsible for the generation of electrophilic iodine intermediate [M(x-1)Oy]ˉ I+ (mostly KOI) or Bˉ I+ (mostly HOI) along with MI. Then, the I+ species participates in nuclear electrophilic substitution of aromatics or heteroaromatics (1) to give aryl/heteroaryl iodide (2). The complex base and substance system of WEPA is assumed to be accountable for the activation of aromatic/heteroaromatic systems towards their participation in substitution, thus making the iodination very rapid.
3.4. Comparison of reported methods with the present protocol
presents a comparison of various C–H iodination protocols with the present method and it clearly displays several advantages of the present protocol over the reported methods.
Table 4. Comparison of reported iodinations of aromatics/heteroaromatics using I2 as iodine source.
4. Conclusions
In summary, WEPA has been demonstrated as a biorenewable waste-derived media for the nuclear iodination of aromatics/heteroaromatics using I2 under added metal and external oxidant/additive/catalyst-free ambient conditions. The present method shows remarkable assets as significant regioselectivity (preferential formation of mono-iodination products over the other possibilities), wide substrate viability, quick reaction times (5–20 min), ease of operation, internal sustainability, evading chemical waste caused by the use of added substances and up to 99% yields of iodinated products. The nature and position of substituents showed less influence on the present iodination process. A plausible mechanism has been illustrated using some control experiments along with XPS, EDX, XRF, XRD, and FTIR studies. The (hetero)aryl iodides are purified using crystallization technique by avoiding the work-up and column chromatography. Since these reactions are operated using safer catalytic media of bioresource at ambient conditions employing challenging iodination reagent (such as I2) at very mild conditions, this protocol establishes a novel room for the aryl iodination. The synthesized (hetero)aryl iodides may also be investigated for further in-process transformations including C–C homo/cross-couplings, C–hetero atom couplings, nucleophilic reactions, organometallations, etc. Hopefully, the remarkable attributes of the present method with a systematic study of WEPA to be a biorenewable materials for significant chemical transformation such as the synthesis of (hetero)aryl iodides can gain the attention of the scientific community for further investigations of this green technique in synthetic organic chemistry, energy, and materials related applications.
Supplemental Material
Download MS Word (2.4 MB)Acknowledgements
Authors thank CSIR, New Delhi for financial support (Grant No. 02(0196)/14/EMR-II) and fellowship to B. R. Naidu [No. 09/1076(0003)/2018-EMR-I], and J. Lakshmidevi grateful to SERB, New Delhi for the INSPIRE Fellowship (ID: IF150772). M. M. Hanafiah thanks the Universiti Kebangsaan Malaysia for funding (No: DIP-2019-001; GUP-2020-034).
Disclosure statement
No potential conflict of interest was reported by the author(s).
Additional information
Funding
References
- Sodj, M. Desa, U.N. World Population Prospects 2019: Highlights; United Nations Department for Economic and Social Affairs: New York, NY, 2019.
- Venkateswarlu, K. Ashes from Organic Waste as Reagents in Synthetic Chemistry: A Review. Environ. Chem. Lett 2021, 19, 3887–3950.
- Millati, R.; Cahyono, R.B.; Ariyanto, T.; Azzahrani, I.N.; Putri, R.U.; Taherzadeh, M.J. Sustainable Resource Recovery and Zero Waste Approaches; Elsevier: New York, 2019.
- Khanal, S.K.; Varjani, S.; Lin, C.S.K.; Awasthi, M.K. Waste-to-Resources: Opportunities and Challenges. Bioresour. Technol 2020, 317, 123987.
- Abdel-Shafy, H.I.; Mansour, M.S.M. Solid Waste Issue: Sources, Composition, Disposal, Recycling, and Valorization. Egypt. J. Pet 2018, 27, 1275–1290.
- Ahmad, M.S.; Cheng, C.K.; Bhuyar, P.; Atabani, A.E.; Pugazhendhi, A.; Chi, N.T.L.; Witoon, T.; Lim, J.W.; Juan, J.C. Effect of Reaction Conditions on the Lifetime of SAPO-34 Catalysts in Methanol to Olefins Process – A Review. Fuel 2021, 283, 118851.
- Embong, N.H.; Hindryawati, N.; Bhuyar, P.; Govindan, N.; Rahim, M.H.A.; Maniam, G.P. Enhanced Biodiesel Production via Esterifcation of Palm Fatty Acid Distillate (PFAD) Using Rice Husk Ash (NiSO4)/SiO2 Catalyst. Appl. Nanosci 2021, In Press. https://doi.org/https://doi.org/10.1007/s13204-021-01922-4.
- Peixoto, M.L.B.; Silva, C.H.L.; Godoi, M. Generation of New Carbon–Carbon and Carbon–Heteroatom Bonds Mediated by Agro-Waste Extracts: A Review. Environ. Chem. Lett 2021, In Press. https://doi.org/https://doi.org/10.1007/s10311-021-01343-3.
- Malek, M.N.F.A.; Pushparaja, L.; Hussin, N.M.; Embong, N.H.; Bhuyar, P.; Rahim, M.H.A.; Maniam, G.P. Exploration of Efficiency of Nano Calcium Oxide (CaO) as Catalyst for Enhancement of Biodiesel Production. J. Microbiol. Biotech. Food Sci 2021, 11, e3935.
- Ma’arof, N.A.N.B.; Hindryawati, N.; Khazaai, S.N.M.; Bhuyar, P.; Rahim, M.H.A.; Maniam, G.P. Exploitation of Cost-effective Renewable Heterogeneous Base Catalyst from Banana (Musa paradisiaca) Peel for Effective Methyl Ester Production from Soybean Oil. Appl. Nanosci 2021, In Press. https://doi.org/https://doi.org/10.1007/s13204-021-01926-0.
- Pathak, G.; Das, D.; Rajkumari, K.; Rokhum, L. Exploiting Waste: Towards a Sustainable Production of Biodiesel Using Musa Acuminate Peel Ash as a Heterogeneous Catalyst. Green Chem. 2018, 20, 2365–2373.
- Naidu, B.R.; Lakshmidevi, J.; Naidu, B.S.S.; Venkateswarlu, K. Water Extract of Pomegranate Ash as Waste-originated Biorenewable Catalyst for the Novel Synthesis of Chiral tert-Butanesulfinyl Aldimines in Water. Mol. Catal 2021, 511, 111719.
- Appa, R.M.; Lakshmidevi, J.; Prasad, S.S.; Naidu, B.R.; Narasimhulu, M.; Venkateswarlu, K. HClO4·SiO2-Catalyzed Mechanochemical Protocol: An Effective, Economical and Eco-Friendly Preparation of N-(Tert-Butylsulfinyl)imines. ChemistrySelcet 2018, 3, 11236–11240.
- Wang, L.; Zhou, X.; Fredimoses, M.; Liao, S.; Liu, Y. Naturally Occurring Organoiodines. RSC Adv. 2014, 4, 57350–57376.
- Gribble, G.W. Biological Activity of Recently Discovered Halogenated Marine Natural Products. Mar. Drugs 2015, 13, 4044–4136.
- Gribble, G.W. Naturally Occuring Organohalogen Compounds — A Comprehensive Survery. In Progress in the Chemistry of Organic Natural Products; Herz, G., Kirby, G.W., Moore, R.E., Steglich, W., Tamm, C., Eds.; Springer Science & Business Media: Berlin, 2012; pp 1–496.
- Griggs, W.-S.; Divgi, C. Radioiodine Imaging and Treatment in Thyroid Disorders. Neuroimag. Clin. N. Am 2008, 18, 505–515.
- Pimlott, S.L.; Sutherland, A. Molecular Tracers for the PET and SPECT Imaging of Disease. Chem. Soc. Rev 2011, 40, 149–162.
- Cannon, K.A.; Geuther, M.E.; Kelly, C.K.; Lin, S.; MacArthur, A.H.R. Hydrodehalogenation of Aryl Chlorides and Aryl Bromides Using a Microwave-assisted, Copper-catalyzed Concurrent Tandem Catalysis Methodology. Organometallics 2011, 30, 4067–4073.
- Neilson, A.H., Ed. Organic Bromine and Iodine Compounds, in Handbook of Environmental Chemistry, Springer: Berlin, 2003; pp 1–334.
- Ackermann, L. Modern Arylation Methods; John Wiley & Sons: city, 2009.
- Ren, Y.-L.; Shang, H.; Wang, J.; Tian, X.; Zhao, S.; Wang, Q.; Li, F. Nitrogen Dioxide-Catalyzed Electrophilic Iodination of Arenes. Adv. Synth. Catal 2013, 355, 3437–3442.
- Satkar, Y.; Yera-Ledesma, L.F.; Mali, N.; Patil, D.; Navarro-Santos, P.; Segura-Quezada, L.A.; Ramírez-Morales, P.I.; Solorio-Alvarado, C.R. Iodine(III)-mediated, Controlled Di- or Monoiodination of Phenols. J. Org. Chem 2019, 84, 4149–4164.
- Racys, D.T.; Warrilow, C.E.; Pimlott, S.L.; Sutherland, A. Highly Regioselective Iodination of Arenes via Iron(II)-Catalyzed Activation of N-Iodosuccinimide. Org. Lett 2015, 17, 4782–4785.
- Johnsson, R.; Meijer, A.; Ellervik, U. Mild and Efficient Direct Aromatic Iodination. Tetrahedron 2005, 61, 11657–11663.
- Elmi, S.; Heggen, P.; Holmelid, B.; Malthe-Sørensen, D.; Sydnes, L.K. Iodination of Anilines with Sodium Dichloroiodate. Org. Prep. Proced. Int 2016, 48, 385–392.
- Kandepi, V.V.K.M.; Narender, N. Ecofriendly Oxidative Nuclear Halogenation of Aromatic Compounds Using Potassium and Ammonium Halides. Synthesis. (Mass) 2012, 44, 15–26.
- Mitra, S.S.; Sreekumar, K. Polymer-Bound Benzyltriethylammonium Polyhalides: Recyclable Reagents for the Selective Iodination of Amines and Phenols. React. Funct. Polym 1997, 32, 281–291.
- Kajigaeshi, S.; Kakinami, T.; Moriwaki, M.; Tanaka, T.; Fujisaki, S.; Okamoto, T. Halogenation Using Quaternary Ammonium Polyhalides. XIV. Aromatic Bromination and Iodination of Arenes by Use of Benzyltrimethylammonium Polyhalides-Zinc Chloride System. Bull. Chem. Soc. Jpn 1989, 62, 439–443.
- March, J. Advanced Organic Chemistry, 4th ed.; Wiley-Interscience: city, New York, 2000.
- Branytska, O.V.; Neumann, R. An Efficient, Catalytic, Aerobic, Oxidative Iodination of Arenes Using the H5PV2Mo10O40 Polyoxometalate as Catalyst. J. Org. Chem 2003, 68, 9510–9512.
- Pourali, A.R.; Ghanei, M. Direct Iodination of Aromatic Compounds with Polyvinylpyrrolidone Supported Hydrogen Peroxide (PVP-H2O2) and Potassium Iodide or Molecular Iodine. Chin. J. Chem 2006, 24, 1077–1079.
- Firouzabadi, H.; Iranpoor, N.; Shiri, M. Direct and Regioselective Iodination and Bromination of Benzene, Naphthalene and Other Activated Aromatic Compounds Using Iodine and Bromine or Their Sodium Salts in the Presence of the Fe(NO3)3·1.5N2O4/Charcoal System. Tetrahedron Lett. 2003, 44, 8781–8785.
- Gallo, R.D.C.; Gebara, K.S.; Muzzi, R.M.; Raminelli, C. Efficient and Selective Iodination of Phenols Promoted by Iodine and Hydrogen Peroxide in Water. J. Braz. Chem. Soc 2010, 21, 770–774.
- Pagni, R.M.; Kabalka, G.W.; Boothe, R.; Gaetano, K.; Stewart, L.J.; Conaway, R.; Dial, C.; Gray, D.; Larson, S.; Luidhardt, T. Reactions of Unsaturated Compounds with Iodine and Bromine on γ Alumina. J. Org. Chem 1988, 53, 4477–4482.
- Sy, W.-W. Iodination of Aromatic Amines with Iodine and Silver Sulphate. Synth. Commun 1992, 22, 3215–3219.
- Benhida, R.; Blanchard, P.; Fourrey, J.-L. A Mild and Effective Iodination Method Using Iodine in the Presence of Bis-(Trifluoroacetoxy)iodobenzene. Tetrahedron Lett. 1998, 39, 6849–6852.
- Tilve, R.D.; Alexander, V.M.; Khadilkar, B.M. Iodination of Activated Arenes Using Silfen: An Improved Protocol. Tetrahedron Lett. 2002, 43, 9457–9459.
- Yang, S.G.; Kim, Y.H. A Practical Iodination of Aromatic Compounds Using Tetrabutylammonium Peroxydisulfate and Iodine. Tetrahedron Lett. 1999, 40, 6051–6054.
- Das, B.; Krishnaiah, M.; Venkateswarlu, K.; Reddy, V.S. A Mild and Simple Regioselective Iodination of Activated Aromatics with Iodine and Catalytic Ceric Ammonium Nitrate. Tetrahedron Lett. 2007, 48, 81–83.
- Krassowska-Świebocka, B.; Luliński, P.; Skulski, L. Aromatic Iodination of Activated Arenes and Heterocycles with Lead Tetraacetate as the Oxidant. Synthesis. (Mass) 1995, 27, 926–928.
- Iskra, J.; Murphree, S.S. Rapid Aerobic Iodination of Arenes Mediated by Hypervalent Iodine in Fluorinated Solvents. Tetrahedron Lett. 2017, 58, 645–648.
- Moorthy, J.N.; Senapati, K.; Kumar, S. IBX–I2 Redox Couple for Facile Generation of IOH and I+: Expedient Protocol for Iodohydroxylation of Olefins and Iodination of Aromatics. J. Org. Chem 2009, 74, 6287–6290.
- Xu, C.; Chen, H.; Sugiyama, Y.; Zhang, S.; Li, H.-P.; Ho, Y.-F.; Chuang, C.-Y.; Schwehr, K.A.; Kaplan, D.I.; Yeager, C.; Roberts, K.A.; Hatcher, P.G.; Santschi, P.H. Novel Molecular-Level Evidence of Iodine Binding to Natural Organic Matter from Fourier Transformation Ion Cyclotron Resonance Mass Spectrometry. Sci. Total Environ 2013, 449, 244–252.
- Appa, R.M.; Prasad, S.S.; Lakshmidevi, J.; Naidu, B.R.; Narasimhulu, M.; Venkateswarlu, K. Palladium-catalysed Room-Temperature Suzuki-Miyaura Coupling in Water Extract of Pomegranate Ash, a Bio-derived Sustainable and Renewable Medium. Appl. Organomet. Chem 2019, 33, e5126.
- Lakshmidevi, J.; Appa, R.M.; Naidu, B.R.; Prasad, S.S.; Sarma, L.S.; Venkateswarlu, K. WEPA: A Bio-derived Medium for Added Base, π-Acid and Ligand Free Ullmann Coupling of Aryl Halides Using Pd(OAc)2. Chem. Commun 2018, 54, 12333–12336.
- Lakshmidevi, J.; Vakati, V.; Naidu, B.R.; Raghavender, M.; Rao, K.S.V.K.; Venkateswarlu, K. Pd(5%)-KIT-6, Pd(5%)-SBA-15 and Pd(5%)-SBA-16 Catalysts in Water Extract of Pomegranate Ash: A Case Study in Heterogenization of Suzuki-Miyaura Reaction Under External Base and Ligand Free Conditions. Sustain. Chem. Pharm 2021, 19, 100371.
- Sravani, B.; Raghavendra, P.; Chandrasekhar, Y.; Reddy, Y.V.M.; Sivasubramanian, R.; Venkateswarlu, K.; Madhavi, G.; Sarma, L.S. Immobilization of Platinum-Cobalt and Platinum-Nickel Bimetallic Nanoparticles on Pomegranate Peel Extract-treated Reduced Graphene Oxide as Electrocatalyst for Oxygen Reduction Reaction. Int. J. Hydrogen Energy 2020, 45, 7680–7690.
- Appa, R.M.; Lakshmidevi, J.; Naidu, B.R.; Venkateswarlu, K. Pd-Catalyzed Oxidative Homocoupling of Arylboronic Acids in WEPA: A Sustainable Access to Symmetrical Biaryls Under Added Base and Ligand-free Ambient Conditions. Mol. Catal 2021, 501, 111366.
- Appa, R.M.; Naidu, B.R.; Lakshmidevi, J.; Vantikommu, J.; Venkateswarlu, K. Added Catalyst-free, Versatile and Environment Beneficial Bromination of (Hetero)aromatics Using NBS in WEPA. SN Appl. Sci 2019, 1, 1281.
- Venkateswarlu, K.; Rao, K.U. Cu(OAc)2-Porphyrins as an Efficient Catalytic System for Base-Free, Nature Mimicking Chan-Lam Coupling in Water. Appl. Organomet. Chem 2021, 35, e6223.
- Sheldon, R.A. The E Factor 25 Years On: The Rise of Green Chemistry and Sustainability. Green Chem. 2017, 19, 18–43.
- Pathak, G.; Rajkumari, K.; Rokhum, L. Wealth from Waste: M. Acuminate Peel Waste-Derived Magnetic Nanoparticles as a Solid Catalyst for Henry Reaction. Nanoscale Adv 2019, 1, 1013–1020.
- Husin, H.; Asnawi, T.M.; Firdaus, A.; Husaini, H.; Ibrahim, I.; Hasfita, F. Solid Catalyst Nanoparticles Derived from Oil-Palm Empty Fruit Bunches (OP-EFB) as a Renewable Catalyst for Biodiesel Production. IOP Conf. Ser. Mater. Sci. Eng 2018, 358, 012008.
- Reddy-Noone, K.; Jain, A.; Verma, K.K. Liquid-phase Microextraction and GC for the Determination of Primary, Secondary and Tertiary Aromatic Amines as Their Iodo-derivatives. Talanta 2007, 73, 684–691.
- Ahmed, A.; Dhara, S.; Singha, R.; Nuree, Y.; Sarkar, P.; Ray, J.K. Palladium Catalyzed One-pot Synthesis of 2-(Pyridine-4-yl) Quinolones via a Multicomponent Unprecedented Reaction of Pyridine-4-Carbaldehyde, 2-Iodoaniline and Triethylamine. RSC Adv. 2014, 4, 53137–53141.
- Edgar, K.J.; Falling, S.N. An Efficient and Selective Method for the Preparation of Iodophenols. J. Org. Chem 1990, 55, 5287–5291.
- Georgiades, S.N.; Clardy, J. Preparation of Psammaplysene-based Library. Org. Lett 2006, 8, 4251–4254.
- Racys, D.T.; Sharif, S.A.I.; Pimlott, S.L.; Sutherland, A. Silver(I)-catalyzed Iodination of Arenes: Tuning the Lewis Acidity of N-iodosuccinimide Activation. J. Org. Chem 2016, 81, 772–780.
- Lee, K.; Dudley, M.W.; Hess, K.M.; Lynn, D.G.; Joerger, R.D.; Binns, A.N. Mechanism of Activation of Agrobacterium Virulence Genes: Identification of Phenol-binding Proteins. Proc. Natl. Acad. Sci. USA 1992, 89, 8666–8670.
- Patil, A.M.; Kamble, D.A.; Lokhande, P.D. A Metal-free Iodination of Aryl Ethers and Phenols Using I2. ChemistrySelect 2017, 2, 8418–8422.
- Batu, G.; Stevenson, R. Synthesis of Isotubaic Acid (Rotenic Acid). J. Org. Chem 1979, 44, 3948–3949.
- Sammelson, R.E.; Casida, J.E. Synthesis of a Tritium-labeled, Fipronil-based, Highly Potent, Photoaffinity Probe for the GABA Receptor. J. Org. Chem 2003, 68, 8075–8079.
- Nouzarian, M.; Hosseinzadeh, R.; Golchoubian, H. Ionic Liquid Iodinating Reagent for Mild and Efficient Iodination of Aromatic and Heteroaromatic Amines and Terminal Alkynes. Synth. Commun 2013, 43, 2913–2925.
- Leboho, T.C.; van Vuuren, S.F.; Michael, J.P.; de Koning, C.B. The Acid-catalysed Synthesis of 7-Azaindoles from 3-Alkynyl-2-aminopyridines and Their Antimicrobial Activity. Org. Biomol. Chem 2014, 12, 307–315.
- Jayakumar, S.; Bhuyar, P.; Pugazhendhi, A.; Rahim, M.H.A.; Maniam, G.P.; Govindan, N. Effect of Light Intensity and Nutrients on the Lipid Content of Marine Microalga (Diatom) Amphiprora sp. for Promising Biodiesel Production. Sci. Total Environ 2021, 768, 145471.
- Khazaai, S.N.M.; Bhuyar, P.; Rahim, M.H.A.; Alwi, M.H.F.M.; Yiting, S.; Maniam, G.P. Rapid Determination of Diesel/Biodiesel Blend Ratio Using Refractive Index, Density, and Kinematic Viscosity Measurements. Biomass Conv. Bioref 2021, In Press. https://doi.org/https://doi.org/10.1007/s13399-021-01921-z.
- Nurhayati, M.; Linggawati, A.; Anita, S.; Amri, T.A. Preparation and Characterization of Calcium Oxide Heterogeneous Catalyst Derived from Anadara Granosa Shell for Biodiesel Synthesis. KnE Eng 2016, 2016, 1–8.
- Abrinaei, F. Nonlinear Optical Response of Mg/MgO Structures Prepared by Laser Ablation Method. J. Eur. Opt. Soc.-Rapid Publ 2017, 13, 15.
- Wu, X.; Zhang, Y.; Zhang, J.; Liu, R.; Yang, J.; Yang, B.; Xu, H.; Ma, Y. High Pressure X-ray Diffraction Study of Sodium Oxide (Na2O): Observations of Amorphization and Equation of State Measurements to 15.9 GPa. J. Alloys Compd 2020, 823, 153793.
- Hartmann, P.; Bender, C.L.; Sann, J.; Dürr, A.K.; Jansen, M.; Janek, J.; Adelhelm, P. A Comprehensive Study on the Cell Chemistry of the Sodium Superoxide (NaO2) Battery. Phys. Chem. Chem. Phys 2013, 15, 11661–11672.
- Lubkowski, K.; Smorowska, A.; Grzmil, B.; Kozlowska, A. Controlled-Release Fertilizer Prepared Using a Biodegradable Aliphatic Copolyester of Poly(Butylene Succinate) and Dimerized Fatty Acid. J. Agric. Food Chem 2015, 63, 2597–2605.
- Kiran, N.; Baker, A.P.; Wang, G.-G. Synthesis and Luminescence Properties of MgO: Sm3+ Phosphor for White Light-Emitting Diodes. J. Mol. Struct 2017, 1129, 211–215.
- Fan, M.; Wu, H.; Shi, M.; Zhang, P.; Jiang, P. Well-dispersive K2O–KCl Alkaline Catalyst Derived from Waste Banana Peel for Biodiesel Synthesis. Green Energy Environ 2019, 4, 322–327.
- Naidu, M.S.R.; Bensusan, H.B. Reinvestigation of the Orientation of Halogen Substitution in Imidazoles by Nuclear Magnetic Resonance Spectroscopy. J. Org. Chem 1968, 33, 1307–1309.
- Prasad, S.S.; Naidu, B.R.; Hanafiah, M.M.; Lakshmidevi, J.; Marella, R.K.; Lakkaboyana, S.K.; Venkateswarlu, K. Porphyrin N-Pincer Pd(II)-Complexes in Water: A Base-free and Nature-inspired Protocol for the Oxidative Self-coupling of Potassium Aryltrifluoroborates in Open-air. Molecules 2021, 26, 5390.
- Aziz, N.I.H.A.; Hanafiah, M.M.; Ali, M.Y.M. Sustainable Biogas Production from Agrowaste and Effluents – A Promising Step for Small-scale Industry Income. Renew. Energy 2019, 132, 363–369.
- ‘Ainaa’ Idris, S.A.; Hanafiah, M.M.; Khan, M.F.; Hamid, H.H.A. Indoor Generated PM2.5 Compositions and Volatile Organic Compounds: Potential Sources and Health Risk Implications. Chemosphere 2020, 255, 126932.
- Marella, R.K.; Madduluri, V.R.; Lakkaboyana, S.K.; Hanafiah, M.M.; Yaaratha, S. Hydrogen-free Hydrogenation of Nitrobenzene via Direct Coupling with Cyclohexanol Dehydrogenation Over Ordered Mesoporous MgO/SBA-15 Supported Cu Nanoparticles. RSC Adv. 2020, 10, 38755–38766.
- Madduluri, V.R.; Marella, R.K.; Hanafiah, M.M.; Lakkaboyana, S.K.; Suresh babu, G. CO2 Utilization as a Soft Oxidant for the Synthesis of Styrene from Ethylbenzene Over Co3O4 Supported on Magnesium Aluminium Spinel: Role of Spinel Activation Temperature. Sci. Rep 2020, 10, 22170.