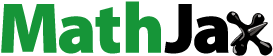
ABSTRACT
Using deseeding nutshell of Eucommia ulmoides as raw material, Eucommia ulmoides rubber (EUR) was directly extracted by high-power ultrasound with high efficiency and the extracting process was accomplished within half an hour at a relatively low temperature. The extract was identified as EUR (trans-polyisoprene) by FT-IR and 1H NMR. The effects of extraction solvents, liquid-to-material ratio, ultrasonic temperature, time, and power on the extraction yields of EUR were investigated, as well as their molecular weights. The procedure parameters were also optimized by the response surface method. The results show that petroleum ether is the most suitable solvent. The highest yield of EUR can reach 89.13% under the following optimized parameters: liquid-to-material ratio 10 (mL/g), ultrasonic power 243 W, ultrasonic time 23 min, and ultrasonic temperature 47°C. The ultrasonic extraction under investigated ultrasonic power shows no significant impact on its molecular weight, except for an extremely long time at high ultrasonic power.
GRAPHICAL ABSTRACT
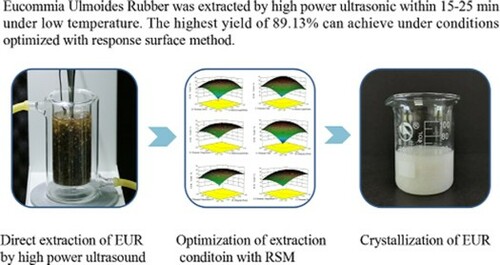
1. Introduction
Eucommia ulmoides rubber (EUR) exists in various tissues of Eucommia ulmoides oliver such as fruit nutshell, bark, leaf, root, etc. It is mainly composed of trans-1,4-polyisoprene () (Citation1). Unlike natural rubber (NR), EUR exhibits trans-conformation, its structure is more regular than that of natural rubber, thus can easily crystallize. EUR demonstrates the property of plastic at ambient temperature, whereas it behaves as rubber when it is highly vulcanized or heated to a higher temperature. The unique rubber-plastic duality of EUR (Citation2) brings about a broad application prospect (Citation3–8).
The traditional extraction methods of EUR mainly include a mechanical method, a pretreatment-solvent extraction method, and so forth. Mechanical method (Citation9), namely, the raw material is subjected to intensified mechanical force to destroy the plant tissue containing EUR, and facilitate separation of EUR from crushed tissue. The primary disadvantage of the method is severe EUR loss. Pretreatment-solvent extraction method (Citation10–16) includes two stages: pretreating raw material with acid/alkali, fungi, or enzymes, which aims to decompose the cellulose, hemicellulose, and lignin encaging EUR; extracting EUR with solvents such as benzene, toluene, petroleum ether, etc. The use of strong alkali, NaOH, results in environmental pollution, and the decomposition of tissue with the bacterium is a time-consuming process. The following solvent extraction is carried out by extracting EUR at refluxing temperature (ca. 70∼90°C) after the pretreated material is washed and dried. The process generally will take several hours to achieve a high extracting yield, and the reflux of solvent may bring about the release of a volatile organic compound and potential danger. In addition, the disadvantage of the method lies in additional procedures such as washing, drying, and transfer of pretreated raw material between two stages. At present, some eco-friendly green pretreatment technologies have been reported (Citation17–19), including pretreatment with the steam explosion, focused microwave, and ionic liquids. However, the common feature of these methods is that the pretreatment of raw material and EUR extraction process is carried out in sequence, which is laborious and time-consuming. Currently, ultrasound was extensively employed in organic synthesis (Citation20–23) and the extraction of various natural products due to its ‘cavitation effect’ and ‘mechanical effect’ (Citation24), e.g. Yuan et al. (Citation25) pretreated Eucommia ulmoides nutshell with low-power ultrasound at first, then extracted EUR with solvent. In this paper, we attempt to develop a simple and effective method to utilize ultrasound, in which the pretreatment and extraction of EUR were carried out in a single step. Unexpectedly, it was found that the use of high-power ultrasound can achieve the goal with satisfactory yields in a relative short time at low temperatures, and the factors affecting extraction yields were screened by single factor test, and then extraction parameters were optimized with the response surface method (RSM) (Citation26, Citation27).
2. Materials and methods
2.1. Plant material and chemical reagents
The nutshell of Eucommia ulmoides was collected from the western Hunan province of China. The collected nutshell raw material was dried to constant weight at 90°C. Petroleum ether (b.p. 60∼90°C), 1,2-dichloroethane, chloroform, and tetrahydrofuran were of analytical grade and purchased from Sinopharm Chemical Reagent Co. LTD. All reagents were used without further purification.
2.2. Direct ultrasonic extraction
The deseeded nutshell (10.0 g) was dispersed in a solvent in a tall-form beaker, ultrasonic cell grinder (Ningbo Xinzhi Biotechnology Co., Ltd.) was employed to treat the mixture under various power at a constant temperature. Direct extraction was performed under the following conditions: ultrasonic power ranging from 100 to 600 W, ultrasonic time from 5 to 50 min, extraction temperature from 15 to 55°C, and liquid-to-material ratio from 8 to 12 mL/g. After extraction, brown liquid containing EUR was filtered with 250 mesh filtration fabric, left at 30°C for 24 h, then filtered with filter paper at 55°C to remove tiny suspended solids. The filtrate containing EUR was cooled to below −10°C for 1 h, and the viscous crystalline EUR was separated and dried to constant weight at 50°C. To determine the actual weight percentage of EUR in raw material, it was treated firstly for 6 h with cellulase and hemicellulase to destroy plant tissues, then extracted using 50 mL petroleum ether at 65°C for 2 h. All data were expressed as the mean of three replicate trials.
The EUR yield (%) was calculated as follows:
(1)
(1) where m is the weight of EUR (g) and M is the weight of EUR in the nutshell (g).
2.3. Experimental design
At the outset, the experimental design was based on a single factor, then RSM was used to optimize the direct ultrasonic extraction conditions for the extraction of EUR. Liquid-to-material ratio (A, mL/g), ultrasonic power (B, W), ultrasonic time (C, min), and ultrasonic temperature (D, °C) were preferred as independent variables. The Box-Behnken design (BBD), with four variables and three levels, was constructed. The yields of EUR were designated as the response-dependent values. The variables and their levels, with both coded and actual values, are presented in .
Table 1. Response surface factor level table.
Based on experimental data from BBD, regression analysis was carried out, and the second-order polynomial model was performed as follows:
(2)
(2) where Y is the yield of EUR (%), b0, bi, bii, and bij are constants, linear, square, and interaction coefficients, respectively. Xi and Xj are the coding values of variables i and j, respectively.
2.4. Characterization of the extract
1H-NMR spectrum was recorded on Bruker AvanceCores (400 MHz), TMS was used as the internal standard, and CDCl3 as solvent. Gel permeation chromatography (GPC) was carried out using Agilent PL-GPC50, tetrahydrofuran (THF) as mobile phase, and monodispersed polystyrene as standard for the calculation of molecular weight.
2.5. Statistical analysis
Design-Expert version 8.0.6 was used to analyze the experimental data. The analysis of variance (ANOVA) was used to evaluate the significant terms in the models. The various statistical analysis parameters, including lack-of-fit test, p-value, F-value, determination coefficient (R2), adjusted determination coefficient (Radj2), and coefficient of variation (C.V. %), were preferred to evaluate the adequacy of the models.
3. Results and analysis
3.1. Direct ultrasonic extraction
The dried nutshells of Eucommia ulmoides were firstly deseeded with a mechanical device to avoid extracting out the oils present in seeds. In comparison, the overall content of EUR was determined by full extraction with solvents after being treated with cellulase and hemicellulase, which could destroy the plant tissue to facilitate extraction. It was estimated that EUR occupied 15.0% of a dry-deseeded nutshell. The direct ultrasonic extractions were carried out in a jacketed beaker, where the raw material was soaked in a solvent. An ultrasonic probe was immersed in the mixture of liquid and material to conduct extraction ((a). After extraction, the extracting EUR solution should be filtered thoroughly through filter fabric and filter paper to remove tiny residues produced from the pulverization of tissue by ultrasound. As shown in (b), white viscous EUR can well crystallize from solvent. The contents of hydrocarbon in these EUR were determined using the stoichiometric reaction of the double bond with bromine, the purities of these EUR were estimated at 95∼96% according to the calculation from the weights of brominated products (Citation28).
3.2. Characterization of the extract
The extract was identified by FT infrared spectroscopy. As shown in , the pattern of absorption peak coincides with that of authentic polyisoprene, in which the characteristic peak of stretching vibration of C=C bond appears at 1663 cm−1, the peaks at 1383 cm−1 and 1447 cm−1 can be assigned to in-plane bending vibration of C-H, and peaks at 2853, 2915, 2964 cm−1 originate from stretching vibration of C-H in methyl and methylene group.
shows the 400 MHz 1H-NMR spectrum of the extract. The pattern of the spectrum is consistent with the standard 1H-NMR spectrum of polyisoprene. The difference between cis- and trans-polyisoprene arises from methyl signal at 1.59∼1.61 ppm, which is ascribed to the methyl group of trans-structure. Furthermore, no methyl signal appears at 1.67 ppm corresponding to cis-configuration, indicating very few amounts of cis-polyisoprene present in the extracting product. According to the calculation from peak areas of respective signals, the content of trans-polyisoprene in extracting product is above 97% (Citation29).
As shown in , all GPC chromatograms of sample 1–4 exhibit the distribution of single peak, and retention times of maximum shift to right, indicating decreasing molecular weights from sample 1 to sample 4. The calculated value of molecular weights (Mw and Mn) and molecular weight distribution (MWD) are shown in . It can be seen that the molecular weights of EUR decrease slightly with the increase of ultrasonic power from 100 W to 300 W when the ultrasonic time is 15 min. Unexpectedly, the molecular weights of EUR decrease markedly when the extraction proceeds for 50 min at 300 W, indicating that the ultrasound possessed enough energy to destroy the macromolecular chain of EUR, especially underhigher ultrasonic power for a long time. Accompanied by decreasing molecular weight, The molecular weight distribution (MWD) becomes broader, as a result of the production of chain fragments with extremely lower molecular weight. The mechanical force of ultrasound can be responsible for the above variation. It can break down the covalent bond of the polymer when the strength is greater than a critical level for a given polymer, as other mechanical forces do, e.g. shearing and milling. With respect to the fracture pattern of the covalent bond of EUR, it is assumed that the degradation of EUR occurs probably in terms of procedure, as shown in , that is, a carbon-carbon single bond in a structural unit is easier to be broken down due to its lower bond energy (∼257KJ/mol) compared with carbon-carbon double bond (∼522 KJ/mol), then resulting free radicals undergo disproportion reaction of capturing hydrogen from other methylene and rearrange to produce a saturated ending and an unsaturated ending.
Table 2. Effect of ultrasound power and time on molecular weights and MWD.
3.3. Single-factor experimental analysis
In this study, the effects of four key parameters including liquid-to-material ratio, ultrasonic power, ultrasonic time, and ultrasonic temperature on extraction yields were selected under investigation. Petroleum ether, chloroform, 1,2-dichloroethane, and tetrahydrofuran were examined as solvents with other fixed extraction conditions as follows: liquid-to-material ratio, 10 mL/g; ultrasonic power, 300 W; ultrasonic time, 15 min; and ultrasonic temperature, 45°C. The results showed the order of EUR yields from high to low was as follows: petroleum ether > 1,2-dichloroethane > chloroform > tetrahydrofuran. The EUR yield for petroleum ether reached 88.20%. Therefore, petroleum ether was selected as the optimal ultrasonic extraction solvent in the following experiments.
Fixing the ultrasonic power, 200 W; ultrasonic time, 5 min; and ultrasonic temperature, 35°C; the influence of the ratio of liquid to the material on the yield EUR is shown in (a). The yields of EUR increase quickly with the increasing ratio of liquid to material from 8 to 10 mL/g, while above 10 mL/g, the yield of EUR decreases slightly. A larger ratio of liquid to material indicates a greater concentration difference between the interior plant cells and the exterior solvent, and the diffusion of EUR occurred more quickly, which could lead to an enhancement of the yield (Citation30). However, when the ratio of liquid to material increases markedly, the ultrasonic energy per unit volume decrease, resulting in decreasing extraction yield (Citation31). Therefore, the ratio of liquid to the material was selected from 9 to 11 mL/g.
Figure 7. Effects of different ultrasonic extraction parameters on the yields of EUR. (a) liquid-to-material ratio (mL/g); (b) ultrasonic power (W); (c) ultrasonic time (min); (d) extraction temperature (°C).
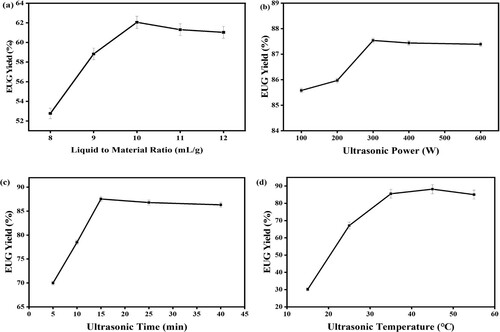
The effect of ultrasonic power on the yield of EUR was studied from 100 to 600 W, other fixed extraction conditions were described as follows: ultrasonic time, 15 min; liquid-to-material ratio, 10 mL/g; and ultrasonic temperature, 35°C. As shown in (b), the yields of EUR increase steadily and reach a maximum value at 300 W, the extraction yield decreases slightly with a higher power. The effect of ultrasound power on the molecular weight is considered comprehensively, and 200 W was selected as the optimal ultrasonic power according to the purpose of saving energy.
The direct ultrasonic extractions were carried out for a certain time, ranging from 5 to 40 min, to investigate the effect of ultrasonic time on the yield of EUR, the other extraction conditions were as follows: ultrasonic power, 300 W; liquid to material ratio, 10 mL/g; and ultrasonic temperature, 35°C. The results are presented in (c). When the extraction time is 5 min, the extraction proceeds incompletely, resulting in a lower extraction yield. The extraction yields of EUR increase sharply from 5 min to 15 min. The excessive increase of ultrasonic time will lead to a slight decrease in the extraction yield, which presumably can attribute to the production of EUG with extremely lower molecular weight, as a result of the action of ultrasonic. The portion of EUG is very soluble in the solvent and cannot crystallize completely under a specified temperature from the solvent. Hence, 10∼25 min was a suitable ultrasonic time for the extraction of EUR from the stem of the deseeding nutshell of Eucommia ulmoides.
To investigate the effect of ultrasonic temperature on the yield of EUR, extraction was carried out from 15 to 55°C, while other extraction conditions were as follows: ultrasonic power, 300 W; liquid-to-material ratio, 10 mL/g; and ultrasonic time, 15 min. As shown in (d), the yields of EUR increase remarkably from 15 to 45°C, while above 45°C, the yields decrease slowly. The high ultrasonic extraction temperature could increase the diffusion coefficient and solubility of EUR, therefore increasing the EUR yield. Therefore, an ultrasonic temperature range of 35-55°C was considered to be optimal in the present experiments.
3.4. Optimization with response surface method
Based on the single factor test and the consideration of GPC test results, the direct ultrasonic extraction was optimized according to the design principle of RSM. Herein four factors, liquid-to-material ratio, ultrasonic power, ultrasonic time, and ultrasonic temperature, were selected as the response surface variables and EUR yield as the response value. Data processing and analysis were performed with Design-Expert software to determine the optimal ultrasonic extraction scheme. The levels of response surface factors are shown in .
Based on ANOVA for the response surface reduced quadratic model given in , multiple fitting regression analysis with Design Expert software was used to obtain the four-element quadratic regression model equation of the EUR Yield (Y) to liquid-to-material ratio (A), ultrasonic power (B), ultrasonic time (C), and ultrasonic temperature (D):
(3)
(3)
Table 3. ANOVA for response surface reduced quadratic model.
The ANOVA on the model shows that the regression model is very different (P < 0.0001), and the misfitting term is not significant (P = 0.1153 > 0.05), the determination coefficient of the regression model equation R2 = 0.9649, indicating the difference between the predicted value and the measured value is small enough to confirm precision and reliability of experimental value. Therefore, this model is adequate to predict and analyze the optimal extraction condition of EUR (Citation32). The three-dimensional response surface and corresponding two-dimensional contour plots of EUR yield are shown in .
By keeping two of four independent factors constant, the response surface constructed by the other two factors explicitly demonstrates the interaction between investigated factors. The shape of the contour can directly reflect the interaction, namely, the ellipse contour indicates strong interaction, and a circle contour means weak (Citation33). It can also be evaluated by the P-value in . It can be seen that only cross-product coefficient (AC) is significant (P < 0.05), the other cross-product coefficients (AB, AD, BC, BD, CD) are insignificant, thus they are abbreviated from quadratic regression model equation; the steep angle of the response surface indicates the influence of the factors, more steep angle means greater influence toward experimental results, as revealed by F-value in , i.e. ultrasonic time and ultrasonic power are the most crucial factors. According to the operability of the optimal extraction conditions of EUR, experiments were carried out under the liquid-to-material ratio of 10 mL/g, ultrasonic power of 243 W, ultrasonic time of 23 min, ultrasonic temperature of 47°C, and the average yield of EUR was 89.13%. In comparison, the estimated value by RSM is 89.44%, which is in good agreement with experimental results. Therefore, optimization with RSM for the extraction of EUR is accurate and reliable.
4. Conclusion
By combining pretreatment and extraction into one step, EUR can be extracted from deseeded nutshell of Eucommia ulmoides by high-power ultrasound. The procedure could be accomplished satisfactorily at a low temperature (25∼50°C) in a short time (15∼30 min). With the optimization by response surface method, the actual extraction yield can reach 89.13% under the optimal process parameters. The variation of their molecular weights shows that extraction with extremely high power or/and a long time will result in a significant decrease of molecular weight due to the fracture of the covalent bond of EUR under severe ultrasonic action. Therefore, in practical use, the power and time of direct ultrasonic extraction should be controlled to avoid obvious negative effects on the molecular weight of EUR.
Disclosure statement
No potential conflict of interest was reported by the author(s).
Additional information
Funding
References
- Li, M.H.; Liu, L.; Lan, T.; Wang, B.L.; Gao, P.; He, W. The Characteristics and Research Progress of Natural Polymer Eucommia Ulmoides Gum. Appl. Chem. Eng. 2018, 47 (05), 1026–1029.
- Guo, Y.Z.; Shi, X.T.; Zhang, J.B.; Lang, Y. Review of Research and Development of Eucommia Ulmoides Gum in China. Sci. Technol. Innov. 2019, 15, 189–190.
- Ni, Q.G.; Wu, J.H.; Kong, P.; Wang, Y.; Li, Y.B.; Li, Y.J.; Peng, X.C. Inverse Vulcanization Polymer-Modified Eucommia Ulmoides Gum with Enhanced Shape Memory Capability and Sound Absorption Property. ACS Appl. Poly. Mater. 2022, 4 (7), 4689–4698.
- Zhang, H.C. Elasticity and Damping Regulation and Molecular Basis of Eucommia Ulmoides Gum; Shanghai: East China Normal University, 2021.
- Zhang, W.; Huang, Z.C.; Zhao, R.F.; Liang, H.; Ma, Y.F.; Shen, Y.G.; Zhong, H.G.; Chen, Z.J.; Zhang, J.C.; Chen, W.H. Efficacy Evaluation of Eucommia Glue Splint Fixation of Rabbit Fracture Model. Chin. Tissue Eng. Res. 2022, 26, 1–7.
- Zhang, Y.; Kang, H.L.; Fang, Q.H. Preparation and Properties of Conductive Anticorrosion Coatings with Epoxy Resin Modified by Eucommia Rubber/Carbon Nanotubes. Synth. Rubber Ind. 2022, 45 (01), 54–59.
- Wang, Z.Y.; Li, D.H.; Kang, H.L.; Fang, Q.H. Preparation and Properties of Graphene/Eucommia Ulmoides Gum Absorbing Materials. Funct. Mater. 2021, 52 (10), 10016–10022.
- Wei, X.N.; Peng, P.; Peng, F.; Dong, J.E. Natural Polymer Eucommia Ulmoides Rubber: A Novel Material. J. Agr. Food Chem. 2021, 69 (13), 3797–3821.
- Kim, J.S.; Lee, Y.Y.; Kim, T.H. A Review on Alkaline Pretreatment Technology for Bioconversion of Lignocellulosic Biomass. Biores. Technol. 2016, 199, 42–48.
- Zhou, P.; Peng, Z.Y. Extraction of Eucommia ulmoides Gum by Acetic Acid Pretreatment. Biol. Process. 2018, 16 (04), 61–64.
- Zhang, X.J.; Zhou, L.H.; Zhang, G.F.; Tan, H.; Ji, C. Extraction of Eucommia Ulmoides gum from Leaves and Bark. J. Guizhou Univ. Technol. (Natural Science Edition) 2001, 06, 11–14.
- Qian, S.Y. The Effect of Inonotus Obliquus Pretreatment on the Extraction of Eucommia Ulmoides Gum and Lignocellulose Saccharification Efficiency; Hangzhou: Zhejiang University of Technology, 2020.
- Zhang, X.J.; Cheng, C.; Zhang, M.M.; Lan, X.Y.; Wang, Q.H.; Han, S.F. Effect of Alkali and Enzymatic Pretreatments of Eucommia Ulmoides Leaves and Barks on the Extraction of Gutta Percha. J. Agr. Food Chem. 2008, 56 (19), 8936–8943.
- Liang, N.N.; Wang, Y.W.; Xia, L.; Xin, Z.X.; Zhang, X.J. Structure and Extraction Efficiency of Natural Eucommia Ulmoides Gum Extracted by Compound Enzymatic Hydrolysis. J. Qingdao Univ. Sci. Technol. (Natural Science Edition) 2014, 35 (04), 383–386.
- Xie, L.; Tao, H.; Zhang, X.J.; Zhang, L.L.; He, Y.J.; Tian, Y.X.; Wu, Q.L.; Ji, C. Optimization of Ultrafiltration Process for Enzymatic Extraction of Eucommia Ulmoides Wing Pericarp. Chin. Pharm. 2021, 32 (13), 1557–1564.
- Liu, G.H.; Zhang, Y.K.; Xiao, M.F.; Peng, X.J. Study on Extraction of Eucommia ulmoides gum by Cellulase Pretreatment. Forest Chem. Ind. 2010, 30 (02), 77–82.
- Wei, J.J.; Xin, D.L.; Cheng, X.; Quan, X.Y.; Zhang, J.H. Effect of Steam Explosion on Extraction of Bioactive Components and Gutta-Percha from Bark of Eucommia Ulmoides Oliver. Chem. Ind. Forest Prod. 2019, 39 (01), 88–94.
- Zhang, Y.; Wang, S.S.; Li, H.; Xiang, C.Q. Focused Microwave Assisted Extraction of Eucommia Ulmoides gum from Eucommia Ulmoides Seed Shell. Nat. Prod. Res. Dev. 2016, 28 (06), 904–909 + 942.
- Lin, C.X.; Yi, H.; Chen, L.; Liu, Y.F.; Ye, X.X.; Lv, Y.C.; Liu, M.H. Highly Efficient Extraction of Eucommia Ulmoides Gum by IL-Organic Solvent Biphasic System. Ind. Crops Prod. 2022, 180, 114735.
- Leila, M.; Mina, Z. Ultrasound-Promoted Green Synthesis of 1,4-Dihydropyridines Using Fuctionalized MWCNTs as a Highly Efficient Heterogeneous Catalyst. Green Chem. Lett. Rev. 2018, 11 (3), 197–208.
- Neda, M.D.; Mohammad, G. Ultrasound-Promoted Green Approach for the Synthesis of Multisubstituted Pyridines Using Stable and Reusable SBA-15@ADMPT/H5PW10V2O40 Nanocatalyst at Room Temperature. Green Chem. Lett. Rev. 2020, 13 (3), 192–205.
- Mohammad, A.G.; Mohammad, H.A.B. Ultrasound-Assisted One-Pot Multi-Component Synthesis of 2-Pyrrolidinon-3-Olates Catalyzed by Co3O4@SiO2 Core–Shell Nanocomposite. Green Chem. Lett. Rev. 2016, 9 (3), 156–165.
- Ali, A.M.; Dekamin, G. A Facile and Environment-Friendly Polyethylene Glycol 600-Mediated Method for the Synthesis of Densely Functionalized 2-Aminothiophene Derived Undersonication. Green Chem. Lett. Rev. 2017, 10 (4), 315–323.
- Dong, J.E.; Ma, B.L.; Liu, L.; Ma, L.; Zhang, B.Y. Study on Ultrasonic Extraction of Effective Components from Eucommia Ulmoides Leaves. J. Northwest For. Univ. 2003, 18 (03), 66–68.
- Yuan, Y.M.; Cao, Y.P. Ultrasonic Assisted Extraction of Gutta-Percha and Flavonoids from Eucommia. J. Food Sci. Technol. 2014, 32 (2), 67–71.
- Chen, J.Y.; Wang, W.T.; Dong, Y.Y.; Zhang, J.C.; Hu, K.X.; Ma, Q. Response Surface Optimization of Dandelion Inulin Extraction Process and MALDI-TOF MS Analysis. Food Ind. Technol. 2022, 43 (01), 205–212.
- Wang, J.D.; Liu, B.; Chang, Y.L. Optimization of Supercritical Fluid Extraction of Geniposidic Acid from Plantain Seeds Using Response Surface Methodology. Green Chem. Lett. Rev. 2014, 7 (3), 309–316.
- Li, Z.Y. Determination of Rubber Hydrocarbon Content. In Analysis and Test of Natural Rubber; Li, Z.Y., Ed.; China Agricultural University Press: Beijing, China, 2007; pp 89–90.
- SH/T 1832-2020. Determination of the microstructure of isoprene rubber-1H-NMR method.
- Zhao, Z.Y.; Zhang, Q.; Li, Y.F.; Dong, L.L.; Liu, S.L. Optimization of Ultrasound Extraction of Alisma orientalis Polysaccharides by Response Surface Methodology and Their Antioxidant Activities. Carbohydr. Polym. 2015, 119, 101–109.
- Szydłowska-Czerniak, A.; Dianoczki, C.; Recseg, K.; Karlovits, G.; Szłyk, E. Determination of Antioxidant Capacities of Vegetable Oils by Ferric-ion Spectrophotometric Methods. Talanta 2008, 76 (4), 899–905.
- Li, L.; Quan, Q.G.; Shen, Y.X.; He, F.L.; Shao, J.H.; Yan, X.Y. Extraction and Stability of Puerarin from Boveground Parts of Pueraria lobata. Food Ind. Technol. 2016, 37 (09), 235–239.
- Qiu, X.M. Study on Extraction and Antioxidant Activity of Polyphenols from Porphyra haitanensis by Response Surface Methodology. J. Minnan Norm. Univ. (Natural Science Edition) 2019, 32 (02), 81–89.