ABSTRACT
1,2,3-triazole is a five-member nitrogen-containing heterocyclic compound with diverse biological activities. A number of 1,2,3-triazole-containing FDA-approved drugs are available on the market, and many other pharmaceutically significant potential molecules are under clinical investigation. Therefore, it is fairly reasonable to anticipate that there will be an increase in demand for new triazole-containing drugs in the coming future. Because of its broad pharmacological applications, it has recently attracted the attention of scientists worldwide. This review provides a concise summary of the synthetic techniques for triazole compounds from various strategies and their medical importance as a lead structure for the enhancement of therapeutic molecules.
1. Introduction
N-heterocyclic compounds are abundant in nature and play key roles in many physiologically important substances such as vitamins, nucleic acids, medicines, dyes, and agrochemicals. They are well-known in the fields of organic and medicinal chemistry, which are growing quickly, as well as in the pharmaceutical industry (Citation1–7).
Developing a novel therapeutic agent is a challenging task for medicinal chemists. Because they are valuable in a variety of applications, including propellants, explosives, pyrotechnics, and particularly chemotherapy, heterocyclic systems with a high nitrogen content have been synthesized more often during the last decade. Due to their importance in bioactivity and synthetic processes, triazoles and their attached heterocyclic derivatives have garnered a lot of interest in recent years. Azolic derivatives, including thiazole, triazole, oxadiazole, and thiadiazole, are pharmacologically active substances that have undergone extensive research for a variety of biological functions as a result of their successful application in medicinal chemistry (Citation8). The potential of 1,2,3-triazoles as ‘linkers’ has been showcased through the synthesis and assessment of a new range of 1,2,3-triazole-based hybrids and conjugates. These innovative compounds are being explored as primary candidates for various biological applications. Their efficacy spans a broad spectrum, including roles as agents against cancer, microbes, tuberculosis, viruses, diabetes, malaria, leishmaniasis, and in neuroprotection (Citation9). The exploration of the biological importance and synthesis of 1,2,3-triazole derivatives has emerged as a dynamic and promising area of research. This field has witnessed significant advancements, both in terms of understanding the biological activities associated with these derivatives and developing efficient synthetic methodologies. Researchers have delved into the diverse biological significance of 1,2,3-triazole derivatives, uncovering their potential as bioactive compounds with applications in medicinal chemistry. These derivatives have demonstrated a wide range of pharmacological activities, including antimicrobial, anticancer, anti-inflammatory, antiviral, and antifungal properties. The ability of 1,2,3-triazole derivatives to interact with biological targets has spurred interest in their potential as therapeutic agents for various diseases (Citation10, Citation11). Furthermore, the synthesis methodologies of 1,2,3-triazole derivatives have undergone refinement and innovation, with a focus on enhancing efficiency, yield, and sustainability. Advances in synthetic strategies, including click chemistry and green chemistry approaches, have contributed to the development of diverse derivatives with improved biological activities. The interdisciplinary nature of the research has fostered collaborations between synthetic chemists, biochemists, and pharmacologists, leading to a comprehensive understanding of the structure–activity relationships governing the biological effects of these compounds (Citation12, Citation13). Moreover, the exploration of structure–activity relationships (SAR) has played a crucial role in understanding the impact of molecular modifications on the biological properties of 1,2,3-triazole derivatives. This knowledge has informed the design and optimization of new derivatives with enhanced pharmacological profiles (Citation14). However, over the past decade, there has been significant progress in unraveling the biological importance of 1,2,3-triazole derivatives and advancing synthetic methodologies for their preparation. The convergence of these efforts has positioned this field as a key player in drug discovery and development, offering a rich avenue for future research and potential applications in the pharmaceutical industry.
Triazole has the chemical formula C2H3N3, consisting of a heterocyclic ring with three nitrogen atoms and two carbon atoms. Also known as pyrrodiazole, it occurs in two isomeric forms: compounds 1 and 2 (). The 1,2,3-triazole has an aromatic property because all its atoms are sp2 hybridized and the accessible 6π electrons are delocalized in the ring. The synthesis of 1-2-3-triazoles is a well-established approach, such as the 1,4-disubstituted and 1,5-disubstituted 1,2,3-triazoles produced by Huisgen’s 1,3-dipolar cycloaddition reaction of organic azides with alkynes, whereas it is a difficult task for biological systems to create molecules that contain a 5-membered cyclic structure with three adjacent nitrogen atoms. Because of this, it is important to come up with synthetic ways to get triazoles and their derivatives (Citation15, Citation16).
These triazoles are used in a variety of sectors, including healthcare, agrochemicals, and materials science. Their quality as ligands for iron and other metals has made them useful as protective coatings in radiators and cooling systems. The triazole ring is a structural component found in several medications that have antiviral, antiretroviral, anti-inflammatory, anxiolytic, sedative, antidepressant, tranquilizer, anti-allergic, antifungal, anticancer, and other characteristics () (Citation17). Some pesticides that are used to manage weeds and insects also include this ring. Triazoles are often used as precursors because they are stable in a broad range of chemical reactions, including redox, acid–base hydrolysis, moisture, illumination, and biological conditions (Citation18).
2. Pharmacological activities of 1,2,3-triazole derivatives
New chemical constituents and physiologically potent compounds are in high demand. Developing better medications in a short period of time is a constant challenge for modern medicinal chemists. The synthesis of novel patentable compounds that include enhanced activity and selectivity, drug-likeness, and favorable pharmacokinetic characteristics is similarly intriguing. Due to their great stability in both oxidizing and reducing conditions as well as their potential to establish hydrogen bonds, which boost their solubility and promote binding towards biomolecular targets, 1,2,3-triazole moieties are enticing prototypes. It has been shown that triazole derivatives have a broad variety of biological features through several mechanisms of action, such as enzymatic activity, receptor-mediated mechanisms, etc (Citation19–24).
2.1. Anti-cancer activity
Derivatized 1,2,3-triazoles have been researched for an extended period, primarily for their capability in chemotherapy for cancer as shown in . Several organizations have conducted concentrated studies in this specific field. By creating a number of 1,2,3-triazole-tethered 1,2-benzisoxazoles, Rangappa et al. reported an outstanding cytostatic effect against human AML cells. Using the MTT test, compound 13 was found to be the most effective antiproliferative agent against MV4-11 cells, with an IC50 of 2 μM (Citation25). The Miller team developed a series of compounds 14 using a ‘click chemistry’ technique and tested their antiproliferative properties (Citation26). Compound 15 was developed by Grymel et al. and demonstrated an IC50 of 46 μM against MCF-7 cells. When compared to betulin, which exhibited 30% (MCF-7) and 90% (HCT-116) antiproliferative effects after 48 h of incubation, betulin was found to be more cytotoxic to cells (Citation27). With an IC50 of 1.02-74.28 μM, the 1,2,3-triazole with compound 16 demonstrated a wide-ranging ability to inhibit cell growth across six cancer cell lines, as demonstrated by Luan et al (Citation28). Gholampour et al. synthesized new 1,4-naphthoquinone-1,2,3-triazole hybrids and tested their anticancer activity on three human cancer cell lines: MCF-7, HT-29, and MOLT-4. The compounds showed notable cytotoxicity, with compound 17 being the most effective, particularly at 10 and 20 μM concentrations, where it arrested the cell cycle in the G0/G1 phase (Citation29).
2.2. Anti-microbial activity
The biological activity of the triazoles containing various nucleoside derivatives, including theophylline, was evaluated by Ruddarraju et al. Compound 18 had significant antimicrobial efficacy against three distinct bacterial species, namely B. cereus, E. coli, and P. aeruginosa, as evidenced by the minimum inhibitory concentration (MIC) values of 0.0156, 0.03125, and 0.0625 mg/mL, respectively (Citation30). The hybrid compounds 19 and 20, which consist of a 1,2,3-triazole-benzoimidazole structure, demonstrated antibacterial activity against clinical strains of Gram-positive and Gram-negative bacteria that are resistant to antibiotics as shown in . The insertion of iso-propyl into amidine might boost the activity in comparison to the unsubstituted hybrids; however, the cyclization of amidine was harmful to the action in general (Citation31). Souza etal. developed 1,2,3-triazole-2-amino-1,4-naphthoquinone derivatives, demonstrating low cytotoxicity (CC >100μM) and no hemolysis or in vivo toxicity. Among these, one compound exhibited significant potency with an IC value of 0.8 μM. Particularly, compound 21 showed IC values <1 μM, with a selectivity index ranging from 6.8–343 for HepG2 cells, and 13.7–494.8 for Vero cells, indicating potential for therapeutic applications (Citation32). Oramas-Royo et al. examined 1,2,3-triazole-naphthoquinone conjugates for their antimalarial activity against Plasmodium falciparum and their effects on tumor cell lines SKBr-3, MCF-7, and HEL. The standout compound 22 displayed the most potent antimalarial activity with an IC50 of 0.8 μM, while also showing low antiproliferative activity against the tumor cells (Citation33).
2.3. Anti-inflammatory activity
Arachidonic acid is released from membrane-bound phospholipids as part of the very complicated biological process of inflammation, which also involves biotransformation processes involving the enzymes cycloxygenase (COX) and 5-lipoxygenase (5-LOX). Numerous NSAIDs, including ibuprofen, indomethacin, and naproxen, stop the metabolism of AA by stifling COX. Traditional NSAIDs, however, have dangerous side effects such as renal failure, ulcers, and longer bleeding times following injuries when they are used for a lengthy period of time. As a result, the present situation needs urgently novel inflammatory drugs (Citation34).
Angajala et al. used click chemistry to derivatize 1,2,3-triazoles based on oxazolo-pyridine and tested their inhibitory efficacy against Glycogen Synthase Kinase-3 (GSK-3) in vitro. Interestingly, analog 23 had the maximum level of inhibition with an IC50 of 0.19 μM. Most of the drugs that showed strong GSK-3 inhibition were tested for their anti-inflammatory effects in rats with paw edema (Citation35). Naaz et al. used click chemistry to create 1,2,3-triazole derivatives with anti-inflammatory activity in vivo. With strong COX-2 indexes of 0.058 and 0.046, in that order, the two structures 24 and 25 confirmed outstanding inhibition of COX-2 (IC50 0.12μM/IC50 0.12μM) (Citation36) as shown in . Assis et al. conducted a study on the synthesis and evaluation of anti-inflammatory activity and toxicity of phthalimido-alkyl-1H-1,2,3-triazoles. The study revealed that the compound 26 tested was effective in reducing carrageenan-induced paw edema, indicating their potential as anti-inflammatory agents. Additionally, the toxicity studies, including tests against Artemia salina and acute in vivo toxicity, showed no lethality, with an LD50 value of up to 1 g/kg (Citation37).
2.4. Anti-diabetic activity
A common metabolic disorder called diabetes mellitus (DM) is characterized by hyperglycemia, which inhibits the body’s ability to produce insulin. The absence of a proven cure for the illness and the prevalence of DM worldwide have emerged as difficult global challenges. Patients with diabetes mellitus (DM) may develop serious problems like kidney disease, heart disease, nervous system illness, blindness, and limb amputation, due to the chronic dysfunction or failure of numerous physiological organs. There are three primary forms of diabetes, with type 2 diabetes (T2DM) accounting for the majority of cases. The first step in treating T2DM is to lower the amount of D-glucose made by the liver. This can be done by adjusting the activity of digestive enzymes or blocking the enzymes that break down carbohydrates (Citation38, Citation39). A. Iraji et al. developed and synthesized a new class of cyanoacetohydrazide-linked 1,2,3-triazoles and tested their effectiveness as β-D-glucosidase inhibitors. Compound 28 had an IC50 value of 1.0–271.17 μM against β-D-glucosidase, which was significantly lower than the IC50 value of the positive control, acarbose, which was 754.1 μM. Compound 27 (IC50 = 1.00 ± 0.01 μM) with a para chlorobenzyl ring and 28 (IC50 = 1.50 ± 0.01 μM) with an ortho fluorobenzyl pendant group were the most effective β-Dglucosidase inhibitors (Citation40). Dastjerdi et al. prepared derivatives of 1,2,3-triazole-5-carboximidamide, and there activity DPP-4 inhibitory efficacy was assessed. Compound 29 showed stronger inhibitory action than sitagliptin during in vitro testing against DPP-4, with IC50 values of 14.75 μM (Citation41). In order to create effective anti-diabetic drugs, quinozolinone-1,2,3-triazole was created by Saeedi et al. They are novel, powerful β-D-glucosidase inhibitors. All synthetic substances have much more action than the benchmark medication, acarbose. The most active of the title compounds was discovered to be compound 30 as shown in . A docking analysis revealed that the most potent molecule 30, was successfully inserted into the active site of β-D-glucosidase via favorable interactions (Citation42).
2.5. Antitubercular activity
Tuberculosis (TB), an infectious illness produced by bacteria, is still a main threat to global health. Directly monitored therapy with a focus on patient compliance has been the standard approach to treating tuberculosis (TB) for many years. However, the advent of MDR-TB and XDR-TB has created new therapeutic obstacles. Considering this, there has been a lot of focus on finding potential anti-TB drugs. Zhou et al. developed a library of benzofuran salicylic acid compounds based on triazoles using a click chemistry approach. Compound 31 has been found to be a highly active anti-tuberculosis treatment (Citation43). Patpi et al. presented compounds 32–33 shown as potential TB agents with activity against multidrug-resistant strains of Mycobacterium tuberculosis, rifampicin-resistant and drug-susceptible (MTB H37Rv), as well as inhibitory activity against MTB and cytotoxicity towards VERO cells (Citation44) as shown in . Melo de Oliveira et al. synthesized thirty new compounds using CuAAC and N-alkylation. They found that glycerosugars conjugated to 1,2,4-oxadiazole via a 1,2,3-triazole linkage showed strong antiproliferative effects against lung carcinoma (NCIH-460). Notably, compound 34 exhibited significant in vitro inhibition of M. tuberculosis (Mtb H37Rv) with an IC50 of 10 μM (Citation45). Erasmus et al. developed triazole-naphthoquinone hybrids, notably compound 31, which demonstrated significant antimicrobial activity without being toxic to human cells. Compound 35 effectively inhibited Mycobacterium tuberculosis with a MIC90 of 0.5 µM and showed antileishmanial activity with IC50 values of 0.81 and 1.48 µM against Leishmania donovani and L. major, respectively, highlighting its specificity and potency against these pathogens (Citation46).
2.6. Antiviral activity
Viral infections in an organism’s body result in viral diseases. Although there are vaccinations and antiviral medications for curing viral infections, the development of new viruses poses a health hazard to everyone around the globe. It is thus of great importance to create alternative antiviral drugs. Seck and Nguemo highlight the importance of five-membered nitrogen heterocycles, especially those with triazole, imidazole, and thiazole moieties, as coronavirus inhibitors. They review the biological effectiveness and structural characteristics of these compounds in combating coronavirus (Citation47).
Compound 36 received the highest score for a variety of interactions with the biological targets. Cytopathic inhibition and in vitro enzyme inhibition assays in the human Vero E6 cell line showed that the synthesized 1,2,3-triazole-sulfonamide hybrid could be used to treat SARS-CoV-2 and Omicron infections. It could be a strong and safe medicine (Citation48). Andreeva et al. synthesized the 1,2,3-triazolylpyrimidine analog 37, wherein a propylene linkage connects the 1,2,3-triazolylribofuranosyl residue to the 6-methyluracil moiety’s C5 atom. This lead chemical demonstrated both significant antiviral interest (IC50 = 9 μM) and a favorable selectivity index (SI = 16) (Citation49) as shown in . Dantas et al. examined hybrid compounds combining 1H-1,2,3-triazole, naphthoquinone, and phthalimide. They found most compounds were minimally toxic to epithelial cells. Notably, compound 38 effectively inhibited Zika virus (ZIKV) with an IC50 of 146.0 µM and a selectivity index (SI) of 2.3 (Citation50).
2.7. Antifungal activity
One of the most varied organisms in the world is a fungus. The development of novel antifungal and antibacterial chemicals with minimal attraction for human receptors and great selectivity for fungal receptors is necessary since eukaryotes and humans assign several drug-receptor targets (Citation51). Hybrids of 1,2,3-triazoles and tethered coumarin couples were successfully produced in large quantities. A few fungal species were used as test subjects to examine the antifungal impact. Compared to miconazole, compound 39 demonstrated a significant level of antifungal activity (Citation52). 1,2,3-triazole acetamide derivatives as possible SDH inhibitors were identified for plant pathogenic fungal species. The synthesized compound 40 by Yan et al. showed strong antifungal inhibitory properties (Citation53) as shown in .
2.8. Neglected tropical diseases
1,2,3-Triazole compounds are increasingly important in medicinal chemistry, especially for treating Neglected Tropical Diseases (NTDs) caused by kinetoplastid parasites. These include Leishmania donovani (visceral leishmaniasis), Trypanosoma cruzi (Chagas disease), and Trypanosoma brucei (sleeping sickness). Primarily affecting millions in less affluent regions, these diseases are targeted by triazole-based treatments due to their broad spectrum of biological activity. Ongoing research focuses on the potential of both 1,2,3- and 1,2,4-triazole molecules in combating these NTDs, emphasizing their crucial role in global health efforts (Citation54). Exequiel et al. synthesized 1,2,3-triazolylsterol compound 41. These compounds demonstrated significant activity against L. donovani (IC50 0.78 μM), T.brucei (IC50 0.12 μM) and T. cruzi (IC50 0.33 μM) (Citation55). Kalinin et al. synthesized a series of novel triazole ring inhibitors, notably compound 43, which exhibits exceptional inhibitory efficacy against smHDAC8 (IC50 of 0.504 μmol/L), highlighting its potential in treating schistosomiasis. Its structure, showing effective interaction with key smHDAC8 residues, marks a significant advancement over earlier compounds like 42, enhancing both () specificity and potency (Citation56).
3. Synthesis of 1,2,3-triazoles
The ring structure of 1,2,3-triazole has been widely researched due to its ability to potentially interact with several biological approaches. This ring structure has been synthesized using a large range of various synthetic approaches. Most often, one utilizes the Huisgen cycloaddition under heated circumstances (Citation57–59).
The most significant and adaptable way for synthesizing triazoles is the 1,3-dipolar cycloaddition, although there are other approaches documented in the literature (Citation47, Citation48). Other traditional techniques for synthesizing these compounds include beginning with chemical modifications of other heterocycles or intramolecular cyclization of combined bis-hydrazone or semicarbazide atom fragments, rearrangement into triazines, etc. Currently, efforts are underway to develop innovative techniques for synthesizing 1,2,3-triazole derivatives containing distinct substituents at positions 1, 4, and 5 on the molecule (Citation60–65).
Compound 46 was produced by Rengasamy et al. without metal catalytic conditions. Cycloaddition [3 + 2] was used to achieve the desired synthetic result by decarboxylating cinnamic acids 44 and organic azides 45. This method presents a useful synthetic process for 1.4-disubstituted 1,2,3-triazole derivatives because of favorable product formation and easy conditions (Citation66) (Scheme 1).
Microwave-assisted synthesis of derivative 49 has been described by Virgyl, Nicolas et al. The derivatives of 49 were synthesized through a multicomponent reaction of azides, bromides 47, aryl, and alkyl alkynes 49 with excellent yields and regioselectivities (Citation67) (Scheme 2).
Patterson et al. describes an approach for the preparation of 1-substituted 1,2,3-triazoles 51 through a primary amine, 2,2-dimethoxyacetaldehyde 50, and tosyl hydrazide. The main advantage of this synthetic procedure is excellent yields using either aliphatic or substituted aniline. Using this method, Sarah synthesized methyl 4-aminobenzoate derivatized 1,2,3-triazoles in 85% yield using a simple precipitative technique. The methyl 4-(1H-1,2,3-triazol-1-yl) benzoate compound is utilized to prepare the irreversible LSD1 inhibitor (Citation68) (Scheme 3).
The synthesis of compound 54 having aryloxy derivatives by copper-catalyzed one-pot multicomponent consecutive from compound 52 has been described by Asgari et al. In this reaction, excellent yields were obtained by using a H2O/DMF 1:1 (v/v) mixture as a solvent and a 15 mol% CuSO4 catalyst under reflux conditions (Citation69) (Scheme 4).
Saikia et al. described the microwave-assisted three-component synthesis of compound 57. The reaction was conducted between benzyl bromide derivatives 55, NaN3, and substituted alkynes 56 in the presence of an ionic liquid assisted Cu (II) catalyst in methanol under microwave irradiation conditions. The synthetic procedure provides sufficient substrate possibilities, excellent yields, less reaction time in the microwave, and environmentally benign conditions. Also, the reaction amongst electro-releasing groups containing alkyne and benzyl bromide to synthesize triazole derivatives in good yield. With this one-pot protocol, we can synthesize the antiepileptic drug Rufinamide in high yield (Citation70) (Scheme 5).
Li et al. created a library of 5-iodo substituted 1,2,3-triazoles using a liquid iodination method in an air environment. In this reaction, substituted benzyl azides 58 and phenylacetylene 59 react with Et4NI in H2O at room temperature, and 5-iodo 1,2,3-triazoles are obtained in high yield. This process had a high tolerance for functional groups, so it could be used to change proteins as well as nucleosides and carbohydrates. To further its use in double-tagging proteins, it was suggested that this technique could be combined with other kinds of reactions, such as the Sonagashira reaction, to enable multi-functional modifications (Citation71) (Scheme 6).
A novel, effective, and moderately copper-catalyzed approach for synthesizing derivatives 63 from boronic acids 61, sodium azide, and derivatives of 62 was obtained by Garg et al. The aforementioned procedure demonstrates a highly effective synthetic approach for the synthesis of 1,2,3-triazoles. This method involves the utilization of a bio-surfactant solution containing sodium deoxycholate (NaDC) as a micellar medium to facilitate the CuAAC reaction (Citation72) (Scheme 7).
Maiuolo et al. have described the regioselective synthesis of compound 66 through 1,3-dipolar cycloaddition. The Er(OTf)3/[mpy]OTf/H2O catalytic system was employed in a simple process to produce 1,5-disubstituted 1,2,3-triazoles. The reaction occurred between substituted 2-nitrovinyl benzene 64 and azide derivatives 65 in the presence of a catalytic system with water. This 1,3-dipolar cycloaddition proved to be the best catalyst for this reaction, exhibiting remarkable reusability for up to five cycles with no yields loss, showing excellent yield and high regioselectivities (Citation73) (Scheme 8).
Scheme 8. Synthesis of 1,5-disubstituted 1,2,3-triazoles by using [mpy]OTf/H2O/Er(OTf)3 catalytic system.
![Scheme 8. Synthesis of 1,5-disubstituted 1,2,3-triazoles by using [mpy]OTf/H2O/Er(OTf)3 catalytic system.](/cms/asset/4bf597fb-ad90-4cb2-a225-0a8e073ea8d9/tgcl_a_2307989_f0018_ob.jpg)
Using an effective ‘one-pot’ method, Kumar et al. described the preparation of modified derivatives of 1,2,3-triazole compounds 68 and 69 in good yields as a regiospecific isomer. Under moderate conditions, acrylate compounds combine with NaN3 in DMF, and the primary reaction suggests atom-effective tandem azidation, cycloaddition, and aromatization. The methodology’s key strengths include (a) direct synthesis of triazole derivatives from olefins without the requirement for a second step; (b) metal-free conditions; (c) mild reaction conditions; and (d) excellent yields with complete regioselectivity (Citation74) (Scheme 9).
Zheng et al. have developed a highly effective substrate-selective copper-catalyzed technique for the synthesis of derivatives 72 from N-tosyl hydrazones 70 and azides 71. This Cu-catalyzed method allowed a wide variety of substrates and presented a new method to create 1,2,3-triazoles. This method is important for the development of a library of 1,2,3-triazoles. The reactions ran smoothly with high yields. This transition paved to synthesize 1,2,3-triazoles, a basic moiety observed in several natural sources and drugs (Citation75) (Scheme 10).
Ali et al. have developed regioselective derivatives 75 which were synthesized through copper-catalyzed azide–alkyne cycloaddition in the presence of a greener solvent. The use of an ionic liquid medium represents a straightforward method for achieving high yields of regioselective 1,4-disubstituted 1,2,3-triazoles. This approach requires neither bases, reducing agents, nor ligands and can be performed without the need for an inert atmosphere. The cyclization reaction was demonstrated using compound 73 (phenyl azide), and alkynes 74, which yielded 1,4-disubstituted 1,2,3-triazoles in excellent yields (Citation76) (Scheme 11).
The microwave-mediated synthesis of 1,2,3-triazole derivatives 78 has been described by Vergara-Arenas et al. In this reaction, a condensation occurs between substituted aromatic aldehydes 76, nitromethane 77, and sodium azide to give 1,2,3-triazoles with piperidine as a base and heterogeneous catalysts in DMF under microwave irradiation. This method presents a useful synthetic process for 1,2,3-triazole derivatives because of its excellent yields and easy isolation of products. The sites of the catalysts MCM-41, Al-MCM-41, and SZ allowed every step of the multi-component process to perform better: The yield increases as the concentration of acid sites increases (Citation77) (Scheme 12).
A metal-free synthesis of regioselective derivatives 81 and 82 was designated by Reddy et al. The regioselective 1,2,3-triazole derivatives were synthesized at room temperature via the [3+2] cycloaddition of non symmetric compound 79 and 1-alkyl-3-(arylthio)propan-2-ones with a range of aryl, vinyl, and alkyl azides. Excellent yields, a high proportion, and specificity are achieved in the organocatalytic azide−ketone [3 + 2] cycloaddition (OrgAKC) reaction at room temperature using DBU as the catalyst (Citation78) (Scheme 13).
Scheme 13. Synthesis of 1,5 and 1,4-disubstituted 5-arylthiomethyl 1,2,3-triazoles using DBU as a catalyst.
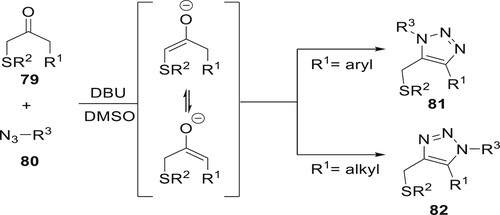
Rajabi-Moghaddam et al. have developed the use of a novel, highly thermally stable catalyst 75 modified by isatoic anhydride as a catalyst in the production of 1,2,3-triazole derivatives 86 in aqueous or organic solvent conditions. Cu(II)MNPs 85 were used as a novel, high-performance catalytic approach in the synthesis of 1,2,3-triazole derivatives. This catalyst can be used in applications for the manufacturing of drugs because it has been completely removed from the reaction. The use of this catalyst in organic processes at high temperatures is made feasible by its remarkable thermal stability (Citation79) (Scheme 14).
Nandi et al. have reported the one-pot synthesis of triazoles by using wet chemical polymers and copper azide nanoparticles. The reaction was achieved at 50°C in methanol. CANP was shown to be an excellent catalyst, with a 95% yield for the click chemistry reaction between substituted benzyl bromide 77 and alkyne 88. A 5-alkynyl 1,4-disubstituted triazole 89 was synthesized when an electron-donating group was attached to the end of an alkyne. A 1,4-disubstituted triazole molecule 90 was synthesized when an electron withdrawing group was attached to the end of an alkyne. Catalytic activity for the reaction of a terminal alkyne with a copper-azide nanoparticle, leading to the production of a triazole derivative, was observed in the retrieved catalyst (without an azide derivative) (Citation80) (Scheme 15).
According to the work of Cui et al., derivatives of 93 and 94 can be synthesized with regioselectivity by CC bond cleavage. They optimized the yield by varying the reaction conditions (solvent and base). The optimal reaction conditions, determined by a series of experiments, were a Cs2CO3-mediated reaction in acetonitrile at room temperature, which resulted in a 90% yield. The primary processes in this transformation were the Regitz diazo shift and the breakage of the C–C link, which resulted in a wide variety of carbonyl-substituted moiety 1,2,3-triazoles. The regioselectivity, functional group tolerance, and mildness of this reaction were all significant properties (Citation81) (Scheme 16).
Highly effective and metal catalyst-free conditions for the preparation of 1,5-diaryl-substituted 1,2,3-triazoles 97 have been created by Kwok et al. To determine the greatest reaction conditions, a model reaction of aryl azide derivatives 95 and aromatic acetylene 96 was studied. Among the few catalysts with which the reaction was performed (KOH, BnNMe3OH, and NMe4OH), tetraalkylammonium hydroxide (NMe4OH) is chosen as the best due to the highest obtained yield (86%). The reaction is unaffected by moisture and ambient oxygen, is easy to experiment with, and does not need a catalyst based on a transition metal (Citation82).
(Scheme 17).
Scheme 17. Synthesis of 1,5-diarylsubstituted 1,2,3-triazoles. Using alkyne dihydropyridines 98 and substituted azides 99, by combining copper acetate and hydrazine hydrate, Bijani et al. successfully constructed a unique, operationally simple, low-cost, and environmentally friendly copper-catalyzed azide alkyne cyclization (CuAAC) process for the synthesis of functionalized triazoles (Citation22) (Scheme 18).
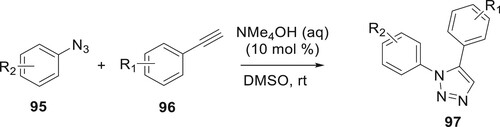
Activated β-cyclodextrin as a heterogeneous support for the copper catalyst immobilization, Cu@βCD-PEG-mesoGO, has been presented. MesoGO was created by synthesizing and functionalizing PEG600-ended β-cyclodextrin with mesoporous silica nanoparticles and graphene oxide. To produce 1,2,3-triazole, Cu@βCD-PEG-mesoGO was used as a ‘green catalyst.’ This reaction was developed by Bahadorikhalili et al. It was reported that during the catalyst recovery test, this catalyst was highly recyclable without significantly losing activity. This catalyst has exceptional qualities, including high efficacy and yield frequency, simple desired product preparation, water utilized as a sustainable and eco-friendly solvent, gentle reaction conditions, and simple catalyst recovery. Alkynes 101 or 102, benzyl bromide 103, and sodium azide were mixed in a three-component reaction using this catalyst to develop the synthesis of 1,2,3-triazole derivatives 1044 or 105 (Citation83) (Scheme 19).
Haque et al. created triazole hybrids based on ferrocene 108. The cyclization reaction is a well-established regioselective technique for producing 1H-1,2,3-triazole-containing fundamental elements for pharmaceutical applications. This approach is very well-liked since it is straightforward, tolerable for bulky functional groups, environmentally benign, and very selective (Citation84) (Scheme 20).
Ali et al. used the well-known ‘click chemistry’ strategy to successfully produce seventeen unique derivatives of 111 with excellent yields. The preparation of 1,2,3-triazole derivatives was completed by performing a Cu(I)-catalyzed Huisgen 1,3-dipolar cycloaddition reaction with compounds 109 and 110 in the occurrence of copper iodide as a catalyst and DHQD2(PHAL) as a ligand in H2O/DCM for 0.5-2 h (Citation85) (Scheme 21).
While some important matters remained unresolved, researchers conducted fundamental mechanistic inquiries to understand how Ag (I) triggers [3+2] cycloaddition. Due to this, Jia and Zhang recently concentrated their research works on emphasizing the crucial role that Ag (I) plays in the three sequential phases such as cycloaddition, deprotonation, and finally protonation under Wang’s condition reaction. Using calculations based on density functional theory (DFT), it was made clear that silver is involved in every stage of the transformation and contributes significantly to the cycloaddition process, which increases the electrophilicity of the isocyanide molecule (Citation86) (Scheme 22).
Bao et al. discovered a synthetic approach for the selective synthesis of 119 and 120 by multi-component reaction between alkynes 117, TMSN3, and ethers by copper catalysis. This condition allowed for the creation of disubstituted 1,2,3-triazoles at 80°C, employing tBuOOH as the oxidant and 118 as both the solvent and the reactant, the desired products were produced with a high yield (86%). In addition, the quantity of cuprous chloride utilized in the synthesis of 119 and 120 may be selectively adjusted to complete the desired product of regulation. This methodology exhibited remarkable regioselectivity, along with exceptional tolerance towards various functional groups and a wide range of substrates (Citation87) (Scheme 23).
Rapid reactions between β-ketoesters and mild bases produce highly reactive enolates, which are said to have numerous applications in organic chemistry. Reactions with 2-unsubstituted ketoesters produced 5-methyl-1,2,3-triazoles in good yields and as a single isomer, whereas reactions with 2-alkyl-substituted β-ketoesters produced 123 in excellent yields and as a single regioisomer. These results were described by Pacifico et al. Various solvents (MeCN and DMSO) were tried out until the optimal ones were found for the process. The maximum yield (98%) was obtained from MeCN when it was heated at 500C. This synthesis resulted in a high yield of 5-hydroxy-1,2,3-triazoles (Citation88) (Scheme 24).
Scheme 24. Synthesis of 5-hydroxy-1,2,3-triazoles and β-ketoesters substituted 5-hydroxy-1,2,3-triazoles
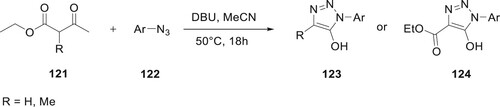
By extending the starting materials and using silver sulfate as a catalyst, Chen et al. demonstrated the high yield production of 127 derivatives through a multi-component reaction involving 125, TMSN3, and 126. At 70°C, 127 were produced utilizing K2CO3 as the base. This procedure offered an efficient way to 127 since it was easy to use, this being compatible with substrates’ functional groups (Citation89) (Scheme 25).
Tosylhydrazones and nitriles would form 1,2,3-triazoles 130 by a cycloaddition using potassium tert-butoxide in xylene at high temperatures, as shown by research from S. Qiu et al. The process is reported without the use of azide and transition-metal-free. When base was added in solvent to accelerate the process, the intermolecular cyclization proceeded easily while the homocoupling of the tosylhydrazone 128 was suppressed (Citation90) (Scheme 26).
Wan et al. created a regioselective synthetic process that uses just molecular iodine to create derivatives of 134 in the absence of metals and azides. In a reaction involving enaminones 131, tosylhydrazine 133, and primary amines 132, triazoles were made by following the formation of C–N and N-N bonds in a cascade and a C–C bond through acyl migration. This reaction included these three components reacting slowly while being assisted by several catalysts. But it was found that I2 in DMSO was the only efficient catalyst to produce derivatives of 134 (Citation91) (Scheme 27).
A well-known technique is the ruthenium assisted azide–alkyne cycloaddition (RuAAC) reaction for the synthesis of substituted 1,2,3-triazoles. Engholm et al. have devised a solid-phase variation of this reaction to synthesize 137. At room temperature, under the influence of the Cp/RuCl(COD) catalyst, 136 was reacted with 135 bound to a solid phase, resulting in the formation of triazole molecule. Under mild conditions, both internal and distal alkynes undergo highly regioselective cycloaddition reactions. This facilitates the liberation of the peptide complex from the resin while maintaining the protection of the side chains. The conversion to 1,2,3-triazoles was almost complete within one hour, allowing for nearly quantitative yields (Citation92) (Scheme 28).
Xavier et al. employed sono-chemistry to catalyze the organocatalytic enamine-azide [3+2] cycloadditions of 138 derivatives with different substituted 139. This new way to produce derivatives of 140 with high productivity and less reaction times in DMSO was found by using sonochemistry, which showed that different aryl azides or β-oxo amides could be used for these reactions (Citation93) (Scheme 29).
Mondal et al. have developed an heterogeneous catalyst based on cerium (III) as a metal 144 for the one-pot synthesis of derivatives of 145, starting from arylamines. Cerium (III) is functionalized with 2-aminothiophenol 141. The polystyrenecerium catalyst was next examined for the response among 143 and 142 derivatives, which were both produced in H2O at room temperature directly from related anilines. The equivalents of 145 were produced in 3 to 4 h with good yield (Citation94) (Scheme 30).
Garg et al. have developed a method that is both environmentally friendly and highly effective for the synthesis of biologically significant 4-aryl-NH-1,2,3-triazoles 148. The method involves a multicomponent reaction beginning with aldehyde 146 and involving nitroalkane 147, sodium azide, and polyethylene glycol 400. The reaction is instead applied to microwave irradiation. Additionally, the catalyst may be reused up to the fifth cycle without significantly decreasing product output. The advantages of using microwave (MW) irradiation as an alternative energy source include low costs, abundant supplies of reagents, the absence of metals, and excellent product yields (Citation95) (Scheme 31).
Using a novel Ru catalyst 141, Arafa and Nayl created an intriguing new approach for the regioselective preparation of 152 in H2O. This approach uses ultrasonic irradiation as an alternate energy source to address the primary challenges associated with using ruthenium for azide–alkyne cycloaddition (AAC) reactions, namely, a longer reaction period, a restricted application, and the need for thermal activation. The catalyst for this reaction was prepared by first obtaining diamide ligands by reacting picolinic acids along with o-phenylene diamines in the presence of triphenyl phosphite. To obtain the Ru-catalyst, the diamide ligands were continuously exposed to ultrasonic irradiation throughout the process. During this period, they were sequentially reacted with ruthenium (in the form of [Ru(DMSO)4]Cl2) and 4-picoline. After careful analysis, it was established that the Ru catalyst, when utilized at a concentration of 0.1 mol%, achieved the desired product in a quantitative yield within a remarkably short time of 7 min. Notably, all the catalysts examined demonstrated exceptional yields for product formation. The scope of the reaction was then evaluated by combining a variety of substrates. And the results ranged from almost quantitative (96%) to nearly perfect (99%) in all cases (Citation96) (Scheme 32).
Sebest et al. produced 1,2,3-triazoles 155 directly from the source of triazoles they have synthesized in deep eutectic solvents (DESs). DESs are thought to be one of the most intriguing newly developed green solvents. They are used to create pharmacological building blocks as well as to serve as an extraction medium for naturally occurring active chemicals. The reaction produced by non-isolated triazoline intermediates, this study providing the optimal reaction conditions to produce 1,2,3-triazoles. Regioselective disubstituted triazoles are formed when the reaction is placed in DES for 24 h at room temperature, but derivatives 155 needed the reaction mixture to be heated. The derivatives of 155 were produced using an environmentally benign procedure, despite the approach’s restricted tolerance (Citation97) (Scheme 33).
Kumar et al. have demonstrated a method for the decarboxylation of cinnamic acid with aryl azide, employing a Cu catalyst and yielding 1,5-disubstituted triazoles 158 as the products. By conducting the reaction at 115°C, utilizing Cu(OTf)2 as the catalyst and DMF as the solvent along with ascorbic acid, significant quantities of disubstituted triazoles were successfully obtained. The authors investigated the reactivity of various substrates of 156 and 157 and found that few functional groups could react efficiently under the studied conditions (Citation98) (Scheme 34).
CuNPs@HT was used by Chetia et al. as an efficient catalyst in the selective synthesis of 161 utilizing alkyl azides 159 and terminal alkynes 160. In this procedure, the catalyst is initially prepared by using hydrotalcite as a solid support. The 1,4-disubstituted-1,2,3-triazoles were made by synthesizing coppers and using them in an azide–alkyne cycloaddition procedure. It is an environmentally friendly technology since the reactions take place at room temperature and ethylene glycol is used as the solvent (Citation99) (Scheme 35).
The synthesis of 165 was described by Yamada et al. This was accomplished by the CuAAC of various 162 with benzyl azide 163 in the presence of copper(I) bromide under oxidative conditions. The reaction of 5-stibanotriazoles 164 with hydrochloric acid resulted in the production of 165 (Citation100) (Scheme 36).
In recent years, visible light has developed rapidly as a renewable energy source. Wu et al. investigated a novel photoredox catalysis route for the preparation of derivatives 169. The scientists showed that photocatalyzed AAC (PcAAC) is feasible (LEDs) by examining a variety of typical photocatalysts, including 168, and others, in various solvents and under various light-emitting diodes. The use of 168 as a catalyst in methylene dichloride as a solvent for six hours while exposed to white LED light aided in the establishment of ideal reaction conditions (Citation101) (Scheme 37).
Wang et al. reported a three-component copper-catalyzed process to generate derivatives of 174 from 170, 171, and 172. Library of 174 compounds could be synthesized successfully at 120°C utilizing Cu(CH3COO)2 as a catalyst. The reaction operates with ease and exhibits strong regioselectivity as well as excellent biocompatibility in the air. Numerous 174 derivatives have been created and have shown in vitro anticancer activity (Citation102) (Scheme 38).
Under the catalysis of DBU 177, Shu et al. reported that aryl azides 176 could successfully undergo cycloaddition with 2-styrylbenzo[d]thiazoles. This method resulted in the regioselective synthesis of 178 derivatives with yields varying from 60 to 80% (Citation103) (Scheme 39).
The observed chemoselectivity was attributed to the electronic influences of the azide ligand and the quantity of the base used in the reaction. Pokhodylo et al. selected the cycloaddition of 180 with cyclic 179 in the presence of an excess of K2CO3 in DMSO. To the best of our knowledge, this is the first time that derivatives 181 have been synthesized. It is interesting to note that a benzo-fuzed sulfone or a five-membered cyclic ketone cannot give the matching triazole compounds. CH3ONa in methanol led mostly to the synthesis of aniline, a byproduct of diazo transfer (Citation104) (Scheme 40).
Kafle et al. demonstrated that aurones can be converted into 183. An extensive series of 2H-triazoles was created with outstanding yields by treating different aurones 182 with NaN3 at high temperatures. Based on preliminary 1H NMR analysis, the reduced productivity was credited to final compound losses during purification. Fascinatingly, thioaurones did not seem to respond under the similar circumstances (Citation105) (Scheme 41).
The first synthesis techniques for asymmetrical 187 are presented by Vroemans et al. 184 is effectively combined with several organic azides 186 and nitroalkanes 185 to produce 177. The equivalent nitroalkane is then oxidatively cyclized through several organic azides to produce asymmetrical tetra-ortho substituted in the second related procedure (Citation106) (Scheme 42).
Silaichev et al. have used 2-cyanoacetamidines and sulfonyl azides in two different ways to make a wide range of new 189 compounds and 1-substituted 5-cyano-1,2,3-triazoles 190. Only 5-cyano-1,2,3-triazoles are produced in the absence of a base. Triazoles are the sole produced by the reaction when sodium ethoxide or DBU is present, causing a 1,5-protic shift in the reaction’s conclusion while still engaging the cyano group. By employing a one-pot synthesis approach involving sodium azide and sulfonyl chlorides, it was possible to achieve the selective and efficient synthesis of both substituted triazoles 3- or 4-methylene-1,2,3-triazole-5-imines. The formation of these products was attributed to the two powerful H-bonds, N and O, present in these molecules (Citation107) (Scheme 43).
Yang et al. reported a unique approach for achieving AAC of cycloheptenones with azides using light. The photochemical conversion produced the more reactive, separated E-isomers of the cycloheptenones. These isomers then went through cycloaddition to make intermediate triazolines. The result of this process was fuzed 1,2,3-triazoles. Intriguingly, throughout the optimization investigation, its regioisomer was commonly found in modest amounts. It investigated the range of cyclic alkenones and azides. From fuzed cycloheptenones derivatives as well as from cyclooctene, 192 triazoles were successfully produced in fair to good yields (Citation108) (Scheme 44).
A magnetic Fe3O4@SiO2 nanocatalyst with a pyridine-tetrazole ligand supported copper (II) acetate was created by Abdalkareem Jasim et al. and utilized to catalyze a one-pot, multi-component synthesis of 196. These three-component reactions can benefit significantly from the use of this nanomaterial as a catalyst. The [MNPs-Py/Tet-Cu(OAc)2] nanocatalyst has an effective magnetization of 43 emu/g, can be reused up to seven times while maintaining catalytic activity and is easily removable from the reaction mixture (Citation109) (Scheme 45).
Mazur et al. have developed and improved a unique catalyst-free synthesis method for 1,2,3-triazolobenzodiazepinones 197 and targeted heterocyclic compounds were synthesized in variable quantities via the Ugi reaction of 2-azidobenzaldehyde, various amines, isocyanides, and acids, followed by microwave-assisted intramolecular azide–alkyne cycloaddition. Unexpectedly, it was discovered that the typically necessary ruthenium-based catalysts have no impact on the IAAC and instead made it more difficult to isolate the target compounds. Catalyst-free conditions assisted by microwaves were efficient for both terminal and non terminal alkynes (Scheme 46).
One of the most effective tandem techniques enables approach to a huge range of heterocyclic derivatives by combining an isocyanide-based multicomponent Ugi reaction. 1,2,3-triazolobenzodiazepines have been synthesized frequently over the past ten years by coupling an intramolecular azide–alkyne cycloaddition with a Ugi four-component reaction (Citation110).
4. Conclusion
In this review, we summarized Click Chemistry as an excellent approach for the synthesis of 1,2,3 triazoles which have diverse biological significances. This review outlines the literature associated with the different synthetic approaches for the target molecules and their medicinal applications. The synthetic routes mentioned here will be beneficial for researchers. Researchers will continue to develop green economics and easy, moderate synthetic techniques. The five-membered aromatic heterocyclic ring structure, containing three nitrogen atoms and possessing high electron density, provides its scaffolds with a diverse range of potential applications in research and development. This has resulted in the creation of curative treatments with low toxicity and superior biological properties for the treatment of human diseases.
Author’s contribution
Conceptualization, M.J.V., M.R., S.B. and V.J.; methodology, V.J., S.B. and M.J.V.; software, L.B., M.R. and K.Y.T.; writing-original draft preparation, M.J.V., S.B. and V.J.; writing – review and editing, M.R., M.M.A., S.A.F. and I.P.; supervision, L.B., and I.P.; funding acquisition, K.Y.T. All authors have read and agreed to the published version of the manuscript.
Acknowledgement
The authors extend their appreciation to the Deanship of Scientific Research at King Khalid University, Saudi Arabia, for funding this work through the Large Program (grant number RGP-2/374/44). The authors are thankful to the Department of Chemistry, Marwadi University, Rajkot, Gujarat, India.
Disclosure statement
No potential conflict of interest was reported by the author(s).
Additional information
Funding
References
- Kerru, N.; Gummidi, L.; Maddila, S.; Gangu, K.K.; Jonnalagadda, S.B. A Review on Recent Advances in Nitrogen-Containing Molecules and Their Biological Applications,”. Molecules 2020, 25 (8), 1909. doi:10.3390/molecules25081909.
- Mekheimer, R.A.; Abuo-Rahma, G.E.D.A.; Abd-Elmonem, M.; Yahia, R.; Hisham, M.; Hayallah, A.M.; Mostafa, S.M.; Abo-Elsoud, F.A.; Sadek, K.U. New S-Triazine/Tetrazole Conjugates as Potent Antifungal and Antibacterial Agents: Design, Molecular Docking and Mechanistic Study,”. J. Mol. Struct. 2022, 1267, 133615. doi:10.1016/j.molstruc.2022.133615.
- El-Reedy, A.A.M.; Soliman, N.K. Synthesis, Biological Activity and Molecular Modeling Study of Novel 1,2,4-Triazolo[4,3-b][1,2,4,5]Tetrazines and 1,2,4-Triazolo[4,3-b][1,2,4]Triazines. Sci. Rep. 2020, 10 (1), 1–18. doi:10.1038/s41598-019-56847-4.
- Kabir, E.; Uzzaman, M. A Review on Biological and Medicinal Impact of Heterocyclic Compounds. Results. Chem. 2022, 4 (8), 100606. doi:10.1016/j.rechem.2022.100606.
- Li Petri, G.; Holl, R.; Spanò, V.; Barreca, M.; Sardo, I.; Raimondi, M.V. Editorial: Emerging Heterocycles as Bioactive Compounds. Front. Chem. 2023, 11 (April), 1–3. doi:10.3389/fchem.2023.1202192.
- Ullah, F.; Ullah, S.; Khan, M.F.A.; Mustaqeem, M.; Paracha, R.N.; Rehman, M.F.U.; Kanwal, F.; Hassan, S.S.U.; Bungau, S. Applications of Heterocyclic Compounds. Encyclopedia 2022. https://encyclopedia.pub/entry/29879.
- Fang, W.Y.; Ravindar, L.; Rakesh, K.P.; Manukumar, H.M.; Shantharam, C.S.; Alharbi, N.S.; Qin, H.L. Synthetic Approaches and Pharmaceutical Applications of Chloro-Containing Molecules for Drug Discovery: A Critical Review. Eur. J. Med. Chem. 2019, 173, 117–153. doi:10.1016/j.ejmech.2019.03.063.
- Heravi, M.M.; Vavsari, V.F. Recent Advances in Application of Amino Acids: Key Building Blocks in Design and Syntheses of Heterocyclic Compounds. Adv. Heterocycl. Chem. 2015, 114, 77–145. https://doi.org/10.1016/BS.AIHCH.2015.02.002.
- Bozorov, K.; Zhao, J.; Aisa, H.A. 1,2,3-Triazole-Containing Hybrids as Leads in Medicinal Chemistry: A Recent Overview. Bioorg. Med. Chem. 2019, 27 (16), 3511–3531. doi:10.1016/j.bmc.2019.07.005.
- Nehra, N.; Tittal, R.K.; Ghule, V.D. 1, 2, 3-Triazoles of 8-Hydroxyquinoline and HBT: Synthesis and Studies (DNA Binding, Antimicrobial, Molecular Docking, ADME, and DFT). ACS Omega 2021, 6, 27089–27100. doi:10.1021/acsomega.1c03668
- Kumar, S.; Khokra, S.L.; Yadav, A. Triazole Analogues as Potential Pharmacological Agents: A Brief Review. Futurure J. Pharm. Sci. 2021, 7 (1), 106. doi:10.1186/s43094-021-00241-3.
- Ferreira, S.B.; Sodero, A.C.; Cardoso, M.F.; Lima, E.S.; Kaiser, C.R.; Silva, F.P.; Ferreira, V.F. Synthesis, Biological Activity, and Molecular Modeling Studies of 1H-1,2,3-Triazole Derivatives of Carbohydrates as Alpha-Glucosidases Inhibitors. J. Med. Chem. 2010, 53 (6), 2364–75. doi:10.1021/jm901265h.
- Gonnet, L.; Baron, M.; Baltas, M. Synthesis of Biologically Relevant 1,2,3- and 1,3,4-Triazoles: From Classical Pathway to Green Chemistry. Molecules 2021 Sep 18, 26 (18), 5667. doi:10.3390/molecules26185667.
- Gupta, O.; Pradhan, T.; Chawla, G. An Updated Review on Diverse Range of Biological Activities of 1,2,4-Triazole Derivatives: Insight Into Structure Activity Relationship. J. Mol. Struct. 2023, 1274, 134487. doi:10.1016/j.molstruc.2022.134487.
- Santiago, J.V.; Burtoloso, A.C.B. Synthesis of Fused Bicyclic [1,2,3]-Triazoles from γ-Amino Diazoketones. ACS Omega 2019, 4 (1), 159–168. doi:10.1021/acsomega.8b02764.
- Forezi, L.D.S.; Lima, C.G.; Amaral, A.A.; Ferreira, P.G.; de Souza, M.C.B.; Cunha, A.C.; da Silva, F.D.C.; Ferreira, V.F. Bioactive 1,2,3-Triazoles: An Account on Their Synthesis, Structural Diversity and Biological Applications. Chem. Records 2021, 21 (10), 2782–2807. doi:10.1002/tcr.202000185.
- Kharb, R.; Sharma, P.C.; Yar, M.S. Pharmacological Significance of Triazole Scaffold. J. Enzyme Inhib. Med. Chem. 2011, 26 (1), 1–21. doi:10.3109/14756360903524304.
- Chen, Z.; Jiang, Y.; Xu, C.; Sun, X.; Ma, C.; Xia, Z.; Zhao, H.Z. Oleanane-Type Triterpene Conjugates with 1H-1,2,3-Triazole Possessing of Fungicidal Activity. Molecules 2022, 27 (15), 1–13. doi:10.3390/molecules27154928.
- Serafini, M.; Pirali, T.; Tron, G.C. Click 1,2,3-Triazoles in Drug Discovery and Development: From the Flask to the Clinic?,”. Adv. Heterocycl. Chem. 2021, 134, 101–148. doi:10.1016/bs.aihch.2020.10.001.
- Horne, W.S.; Yadav, M.K.; Stout, C.D.; Ghadiri, M.R. Heterocyclic Peptide Backbone Modifications in an α-Helical Coiled Coil. J. Am. Chem. Soc. 2004, 126 (47), 15366–15367. doi:10.1021/ja0450408.
- Padhyar, K.T. A Brief Review on Triazole and its Pharmacological Application. Int. J. Curr. Sci. Res. Rev. 2022, 5 (1), 212–25. doi:10.47191/ijcsrr/V5-i1-25.
- Bijani, S.; Iqbal, D.; Mirza, S.; Jain, V.; Jahan, S.; Alsaweed, M.; Madkhali, Y.; Alsagaby, S.A.; Banawas, S.; Algarni, A.; Alrumaihi, F.; Rawal, R.M.; Alturaiki, W.; Shah, A. Green Synthesis and Anticancer Potential of 1,4-Dihydropyridines-Based Triazole Derivatives: In Silico and In Vitro Study. Life 2022, 12 (4), 519. doi:10.3390/life12040519.
- Singh, P.; Kumar, V. Hybrid Drugs: Design and Applications. Pharmaceuticals (Basel) 2023, 26 (16), 1358. doi:10.3390/ph16101358.
- Dheer, D.; Singh, V.; Shankar, R. Medicinal Attributes of 1,2,3-Triazoles: Current Developments. Bioorg. Med. Chem. 2017, 71 (16), 30–54. doi:10.1016/j.bioorg.2017.01.010.
- Ashwini, N.; Garg, M.; Mohan, C.D.; Fuchs, J.E.; Rangappa, S.; Anusha, S.; Swaroop, T.R.; Rakesh, K.S.; Kanojia, D.; Madan, V.; Bender, A.; Koeffler, H.P.; Basappa, S.; Rangappa, K.S. Synthesis of 1,2-Benzisoxazole Tethered 1,2,3-Triazoles That Exhibit Anticancer Activity in Acute Myeloid Leukemia Cell Lines by Inhibiting Histone Deacetylases, and Inducing p21 and Tubulin Acetylation. Bioorg. Med. Chem. 2015, 23 (18), 6157–6165. doi:10.1016/j.bmc.2015.07.069.
- Stefely, J.A.; Palchaudhuri, R.; Miller, P.A.; Peterson, R.J.; Moraski, G.C.; Hergenrother, P.J.; Miller, M.J. N -((1-Benzyl-1 H-1,2,3-Triazol-4-yl)Methyl)Arylamide as a New Scaffold That Provides Rapid Access to Antimicrotubule Agents: Synthesis and Evaluation of Antiproliferative Activity Against Select Cancer Cell Lines. J Med. Chem. 2010, 53 (8), 3389–3395. doi:10.1021/jm1000979.
- Grymel, M.; Pastuch-Gawołek, G.; Lalik, A.; Zawojak, M.; Boczek, S.; Krawczyk, M.; Erfurt, K. Glycoconjugation of Betulin Derivatives Using Copper-Catalyzed 1,3-Dipolar Azido-Alkyne Cycloaddition Reaction and a Preliminary Assay of Cytotoxicity of the Obtained Compounds. Molecules 2020, 25 (24). doi:10.3390/molecules25246019.
- Luan, T.; Quan, Z.; Fang, Y.; Yang, H. Design, Synthesis and Antiproliferative Activity of Chrysin Derivatives Bearing Triazole Moieties. Chin. J. Org. Chem. 2020, 40 (2), 440–46. doi:10.6023/cjoc201907012.
- Gholampour, M.; Ranjbar, S.; Edraki, N.; Mohabbati, M.; Firuzi, O.; Khoshneviszadeh, M. Click Chemistry-Assisted Synthesis of Novel Aminonaphthoquinone-1,2,3-Triazole Hybrids and Investigation of Their Cytotoxicity and Cancer Cell Cycle Alterations. Bioorg. Chem. 2019, 88 (19), 102967. doi:10.1016/j.bioorg.2019.102967.
- Ruddarraju, R.R.; Murugulla, A.C.; Kotla, R.; Chandra Babu Tirumalasetty, M.; Wudayagiri, R.; Donthabakthuni, S.; Maroju, R.; Baburao, K.; Parasa, L.S. Design, Synthesis, Anticancer, Antimicrobial Activities and Molecular Docking Studies of Theophylline Containing Acetylenes and Theophylline Containing 1,2,3-Triazoles with Variant Nucleoside Derivatives. Eur. J. Med. Chem. 2016, 123, 379–396. doi:10.1016/j.ejmech.2016.07.024.
- Bistrović, A.; Krstulović, L.; Stolić, I.; Drenjančević, D.; Talapko, J.; Taylor, M.C.; Kelly, J.M.; Bajić, M.; Raić-Malić, S. Synthesis, Anti-Bacterial and Anti-Protozoal Activities of Amidinobenzimidazole Derivatives and Their Interactions with DNA and RNA. J. Enzyme Inhib. Med. Chem. 2018, 33 (1), 1323–1334. doi:10.1080/14756366.2018.1484733.
- Kathleen, L.; Ferreira, M.; Rêgo, V.; Pereira, A. Derivatives and Their Evaluation as Therapeutic Strategy for Malaria Control. Eur. J. Med. Chem. 2023, 255, 2–6. doi:10.1016/j.ejmech.2023.115400.
- Oramas-Royo, S.; López-Rojas, P.; Amesty, A.; Gutiérrez, D.; Flores, N.; Martín- Rodríguez, P.; Fernández-Pérez, L.; Estévez-Braun, A. Synthesis and Antiplasmodial Activity of 1,2,3-Triazole-Naphthoquinone Conjugates. Molecules 2019, 24 (21), 3917. doi:10.3390/molecules24213917.
- Rahme, E.; Bernatsky, S. NSAIDS and Risk of Lower Gastrointestinal Bleeding. Lancet 2010, 376 (9736), 146–148. doi:10.1016/S0140-6736(10)60839-2.
- Angajala, K.K.; Vianala, S.; Macha, R.; Raghavender, M.; Thupurani, M.K.; Pathi, P.J. Synthesis, Anti-Inflammatory, Bactericidal Activities and Docking Studies of Novel 1,2,3-Triazoles Derived from Ibuprofen Using Click Chemistry. Springerplus. 2016, 5 (1). doi:10.1186/s40064-016-2052-5.
- Naaz, F.; Preeti Pallavi, M.C.; Shafi, S.; Mulakayala, N.; Shahar Yar, M.; Sampath Kumar, H.M. 1,2,3-Triazole Tethered Indole-3-Glyoxamide Derivatives as Multiple Inhibitors of 5-LOX, COX-2 & Tubulin: Their Anti-Proliferative & Anti-Inflammatory Activity. Bioorg. Chem. 2018, 81. doi:10.1016/j.bioorg.2018.07.029.
- Assis, S.P.D.O.; da Silva, M.T.; da Silva, F.T.; Sant’Anna, M.P.; de Albuquerque Tenório, C.M.; dos Santos, C.F.B.; da Fonseca, C.S.; Seabra, G.; Lima, V.L.; de Oliveira, R.N. Design and Synthesis of Triazole-Phthalimide Hybrids with Anti-Inflammatory Activity. Chem. Pharm. Bull. 2019, 67 (2), 96–105. doi:10.1248/cpb.c18-00607.
- Das, T.; Jayasudha, R.; Chakravarthy, S.K.; Prashanthi, G.S.; Bhargava, A.; Tyagi, M.; Rani, P.K.; Pappuru, R.R.; Sharma, S.; Shivaji, S. Alterations in the gut Bacterial Microbiome in People with Type 2 Diabetes Mellitus and Diabetic Retinopathy. Sci. Rep. 2021, 11 (1), 1–15. doi:10.1038/s41598-020-79139-8.
- Zhang, W.; Li, J.; Lu, S.; Han, N.; Miao, J.; Zhang, T.; Qiang, Y.; Kong, Y.; Wang, H.; Gao, T.; Liu, Y.; Li, X.; Peng, X.; Chen, X.; Zhao, X.; Che, J.; Zhang, L.; Chen, X.; Zhang, Q.; Kan, B. Gut Microbiota Community Characteristics and Disease-Related Microorganism Pattern in a Population of Healthy Chinese People. Sci. Rep. 2019, 9 (1), 1–10. doi:10.1038/s41598-018-37186-2.
- Iraji, A.; Shareghi-Brojeni, D.; Mojtabavi, S.; Faramarzi, M.A.; Akbarzadeh, T.; Saeedi, M. Cyanoacetohydrazide Linked to 1,2,3-Triazole Derivatives: A new Class of α-Glucosidase Inhibitors. Sci. Rep. 2022, 12 (1), 1–15. doi:10.1038/s41598-022-11771-y.
- Dastjerdi, H.F.; Naderi, N.; Nematpour, M.; Rezaee, E.; Mahboubi-Rabbani, M.; Ebrahimi, M.; Hosseinipoor, S.; Hosseini, O.; Tabatabai, S.A. Design, Synthesis and Anti-Diabetic Activity of Novel 1,2,3-Triazole-5-Carboximidamide Derivatives as Dipeptidyl Peptidase-4 Inhibitors. J. Mol. Struct. 2020, 1221, 128745. doi:10.1016/j.molstruc.2020.128745.
- Saeedi, M.; Mohammadi-Khanaposhtani, M.; Pourrabia, P.; Razzaghi, N.; Ghadimi, R.; Imanparast, S.; Faramarzi, M.A.; Bandarian, F.; Esfahani, E.N.; Safavi, M.; Rastegar, H.; Larijani, B.; Mahdavi, M.; Akbarzadeh, T. Design and Synthesis of Novel Quinazolinone-1,2,3-Triazole Hybrids as new Anti-Diabetic Agents: In Vitro α-Glucosidase Inhibition, Kinetic, and Docking Study. Bioorg. Chem. 2019, 83, 161–169. doi:10.1016/j.bioorg.2018.10.023.
- Zhou, B.; He, Y.; Zhang, X.; Xu, J.; Luo, Y.; Wang, Y.; Franzblau, S.G.; Yang, Z.; Chan, R.J.; Liu, Y.; Zheng, J.; Zhang, Z.Y. Targeting Mycobacterium Protein Tyrosine Phosphatase B for Antituberculosis Agents. Proc. Natl. Acad. Sci. U. S. A. 2010, 107 (10), 4573–4578. doi:10.1073/pnas.0909133107.
- Patpi, S.R.; Pulipati, L.; Yogeeswari, P.; Sriram, D.; Jain, N.; Sridhar, B.; Murthy, R.; Anjana Devi, T.; Kalivendi, S.V.; Kantevari, S. Design, Synthesis, and Structure-Activity Correlations of Novel Dibenzo[b,d] Furan, Dibenzo[b,d]Thiophene, and N-Methylcarbazole Clubbed 1,2,3-Triazoles as Potent Inhibitors of Mycobacterium Tuberculosis. J. Med. Chem. 2012, 55 (8), 3911–3922. doi:10.1021/jm300125e.
- de Oliveira, V.N.M.; do Amaral Moura, C.F.; dos Santos Peixoto, A.; Ferreira, V.P.G.; Araújo, H.M.; Pimentel, L.M.L.M.; do Ó Pessoa, C.; Nicolete, R.; Dos Anjos, J.V.; Sharma, P.P.; Rathi, B.; Pena, L.J.; Rollin, P.; Tatibouët, A.; Nascimento de Oliveira, R. Synthesis of Alkynylated 1,2,4-Oxadiazole/1,2,3-1H-Triazole Glycoconjugates: Discovering New Compounds for Use in Chemotherapy Against Lung Carcinoma and Mycobacterium Tuberculosis. Eur. J. Med. Chem. 2021, 220, 113472. doi:10.1016/j.ejmech.2021.113472.
- Erasmus, C.; Aucamp, J.; Smit, F.J.; Seldon, R.; Jordaan, A.; Warner, D.F.; N’Da, D.D. Synthesis and Comparison of in Vitro Dual Anti-Infective Activities of Novel Naphthoquinone Hybrids and Atovaquone. Bioorg. Chem. 2021, 114. doi:10.1016/j.bioorg.2021.105118.
- Seck, L.; Nguemo, F. Triazole, Imidazole, and Thiazole-Based Compounds as Potential Agents Against Coronavirus. Results Chem. 2021, 1. doi:10.1016/j.rechem.2021.100132.
- Al-Humaidi, J.Y.; Shaaban, M.M.; Rezki, N.; Aouad, M.R.; Zakaria, M.; Jaremko, M.; Hagar, M.; Elwakil, B.H. 1,2,3-Triazole-Benzofused Molecular Conjugates as Potential Antiviral Agents Against SARS-CoV-2 Virus Variants. Life 2022, 12 (9), 1–14. doi:10.3390/life12091341.
- Andreeva, O.V.; Garifullin, B.F.; Zarubaev, V.V.; Slita, A.V.; Yesaulkova, I.L.; Saifina, L.F.; Shulaeva, M.M.; Belenok, M.G.; Semenov, V.E.; Kataev, V.E. Synthesis of 1,2,3-Triazolyl Nucleoside Analogues and Their Antiviral Activity. Mol. Diversity 2021, 25 (1), 473–490. doi:10.1007/s11030-020-10141-y.
- Dantas, W.M.; de Oliveira, V.N.M.; Santos, D.A.L.; Seabra, G.; Sharma, P.P.; Rathi, B.; Pena, L.J.; de Oliveira, R.N. Searching Anti-Zika Virus Activity in 1 H-1,2,3-Triazole Based Compounds. Molecules 2021, 26 (19). doi:10.3390/molecules26195869.
- Marzi, M.; Farjam, M.; Kazeminejad, Z.; Shiroudi, A.; Kouhpayeh, A.; Zarenezhad, E. A Recent Overview of 1,2,3-Triazole-Containing Hybrids as Novel Antifungal Agents: Focusing on Synthesis, Mechanism of Action, and Structure-Activity Relationship (SAR). J. Chem. 2022, 2022. doi:10.1155/2022/7884316.
- Akolkar, S.V.; Nagargoje, A.A.; Shaikh, M.H.; Warshagha, M.Z.A.; Sangshetti, J.N.; Damale, M.G.; Shingate, B.B. New N-Phenylacetamide-Linked 1,2,3-Triazole-Tethered Coumarin Conjugates: Synthesis, Bioevaluation, and Molecular Docking Study. Archiv Der Pharmazie (Weinheim) 2020, 353 (11). doi:10.1002/ardp.202000164.
- Yan, W.; Wang, X.; Li, K.; Li, T.X.; Wang, J.J.; Yao, K.C.; Cao, L.L.; Zhao, S.S.; Ye, Y.H. Design, Synthesis, and Antifungal Activity of Carboxamide Derivatives Possessing 1,2,3-Triazole as Potential Succinate Dehydrogenase Inhibitors. Pestic. Biochem. Physiol. 2019, 156, 160–169. doi:10.1016/j.pestbp.2019.02.017.
- Mantoani, S.P.; de Andrade, P.; Chierrito, T.P.C.; Figueredo, A.S.; Carvalho, I. Potential Triazole-Based Molecules for the Treatment of Neglected Diseases. Curr. Med. Chem. 2019, 26 (23), 4403–4434. doi:10.2174/0929867324666170727103901.
- Porta, E.O.J.; Ballari, M.S.; Carlucci, R.; Wilkinson, S.; Ma, G.; Tekwani, B.L.; Labadie, G.R. Systematic Study of 1,2,3-Triazolyl Sterols for the Development of new Drugs Against Parasitic Neglected Tropical Diseases. Eur. J. Med. Chem. 2023, 254, 15378. doi:10.1016/j.ejmech.2023.115378.
- Kalinin, D.V.; Jana, S.K.; Pfafenrot, M.; Chakrabarti, A.; Melesina, J.; Shaik, T.B.; Lancelot, J.; Pierce, R.J.; Sippl, W.; Romier, C.; Jung, M.; Holl, R. Structure-Based Design, Synthesis, and Biological Evaluation of Triazole-Based SmHDAC8 Inhibitors. ChemMedChem 2020, 15 (7), 571–584. doi:10.1002/cmdc.201900583.
- Jiang, X.; Hao, X.; Jing, L.; Wu, G.; Kang, D.; Liu, X.; Zhan, P. Recent Applications of Click Chemistry in Drug Discovery. Expert Opin. Drug Discovery 2019, 14 (8), 779–789. doi:10.1080/17460441.2019.1614910.
- Vaishnani, M.J.; Jain, V.D. Green Synthesis of 1,4-Disubstituted 1,2,3-Triazole Acetamide Derivatives by Utilizing the Click Chemistry Approach. Rasayan J. Chem. 2023, 16 (2), 811–19. doi:10.31788/RJC.2023.1628271.
- Rani, A.; Singh, G.; Singh, A.; Maqbool, U.; Kaur, G.; Singh, J. CuAAC-ensembled 1,2,3-Triazole-Linked Isosteres as Pharmacophores in Drug Discovery: Review. RSC Adv. 2020, 10 (10), 5610–5635. doi:10.1039/C9RA09510A.
- Kumar, S.; Sharma, B.; Mehra, V.; Kumar, V. Recent Accomplishments on the Synthetic/Biological Facets of Pharmacologically Active 1H-1,2,3-Triazoles. Eur. J. Med. Chem. 2021, 212, 113069. doi:10.1016/j.ejmech.2020.113069.
- Rodrigues, L.D.; Sunil, D.; Chaithra, D.; Bhagavath, P. 1,2,3/1,2,4-Triazole Containing Liquid Crystalline Materials: An up-to-Date Review of Their Synthetic Design and Mesomorphic Behavior. J. Mol. Liq. 2020, 297, 111909. doi:10.1016/j.molliq.2019.111909.
- Nemallapudi, B.R.; Guda, D.R.; Ummadi, N.; Avula, B.; Zyryanov, G.V.; Reddy, C.S.; Gundala, S. New Methods for Synthesis of 1,2,3-Triazoles: A Review. Polycyclic Aromat. Compd. 2020, 1–19. doi:10.1080/10406638.2020.1866038.
- De Nino, A.; Maiuolo, L.; Costanzo, P.; Algieri, V.; Jiritano, A.; Olivito, F.; Tallarida, M.A. Recent Progress in Catalytic Synthesis of 1,2,3-Triazoles. Catalysts 2021, 11 (9), 1–48. doi:10.3390/catal11091120.
- Dai, J.; Tian, S.; Yang, X.; Liu, Z. Synthesis Methods of 1,2,3-/1,2,4-Triazoles: A Review. Front. Chem. 2022, 10 (9), 1–24. doi:10.3389/fchem.2022.891484.
- Opsomer, T.; Dehaen, W. Metal-free Syntheses of N-Functionalized and NH-1,2,3-Triazoles: An Update on Recent Developments. Chem. Commun. 2021, 57 (13), 1568–1590. doi:10.1039/D0CC06654K.
- Rengasamy, R.; Vijayalakshmi, K.; Punitha, N.; Paul Raj, J.; Karthikeyan, K.; Elangovan, J. A Novel Route to 1,4-Disubstituted-1,2,3-Triazoles Through Metal-Free Decarboxylative Azide-Alkene Cycloaddition. Tetrahedron Lett. 2021, 84, 153440. doi:10.1016/j.tetlet.2021.153440.
- Camberlein, V.; Kraupner, N.; Bou Karroum, N.; Lipka, E.; Deprez-Poulain, R.; Deprez, B.; Bosc, D. Multi-component Reaction for the Preparation of 1,5-Disubstituted 1,2,3-Triazoles by in-Situ Generation of Azides and Nickel-Catalyzed Azide-Alkyne Cycloaddition. Tetrahedron Lett. 2021, 73, 153131. doi:10.1016/j.tetlet.2021.153131.
- Patterson, S.J.M.; Clark, P.R.; Williams, G.D.; Tomkinson, N.C.O. An Azide and Acetylene Free Synthesis of 1-Substituted 1,2,3-Triazoles. Tetrahedron Lett. 2020, 61 (45), 152483. doi:10.1016/j.tetlet.2020.152483.
- Asgari, M.S.; Bahadorikhalili, S.; Ghaempanah, A.; Rashidi Ranjbar, P.; Rahimi, R.; Abbasi, A.; Larijani, B.; Mahdavi, M. Copper-catalyzed one-pot Synthesis of Amide Linked 1,2,3-Triazoles Bearing Aryloxy Skeletons. Tetrahedron Lett. 2021, 65, 152765. doi:10.1016/j.tetlet.2020.152765.
- Saikia, A.A.; Nishanth Rao, R.; Das, S.; Jena, S.; Rej, S.; Maiti, B.; Chanda, K. Sequencing [3+2]-Cycloaddition and Multicomponent Reactions: A Regioselective Microwave-Assisted Synthesis of 1,4-Disubstituted 1,2,3-Triazoles Using Ionic Liquid Supported Cu(II) Precatalysts in Methanol. Tetrahedron Lett. 2020, 61 (36), 152273. doi:10.1016/j.tetlet.2020.152273.
- Li, L.; Xing, X.; Zhang, C.; Zhu, A.; Fan, X.; Chen, C.; Zhang, G. Novel Synthesis of 5-Iodo-1,2,3-Triazoles Using an Aqueous Iodination System Under air. Tetrahedron Lett. 2018, 59 (39), 3563–3566. doi:10.1016/j.tetlet.2018.08.039.
- Garg, A.; Ali, A.A.; Damarla, K.; Kumar, A.; Sarma, D. Aqueous Bile Salt Accelerated Cascade Synthesis of 1,2,3-Triazoles from Arylboronic Acids. Tetrahedron Lett. 2018, 59 (45), 4031–4035. doi:10.1016/j.tetlet.2018.09.064.
- Maiuolo, L.; Russo, B.; Algieri, V.; Nardi, M.; Di Gioia, M.L.; Tallarida, M.A.; De Nino, A. Regioselective Synthesis of 1,5-Disubstituted 1,2,3-Triazoles by 1,3-Dipolar Cycloaddition: Role of Er(OTf)3, Ionic Liquid and Water. Tetrahedron Lett. 2019, 60 (9), 672–674. doi:10.1016/j.tetlet.2019.01.053.
- Kumar, B.S.; Gadakh, S.; Sudalai, A. Metal-free’ Synthesis of 1,2,3-Triazoles via Tandem Azidation, Intramolecular [3+2]-Cycloaddition and Aromatization of Ethyl Acrylate Derivatives. Tetrahedron Lett. 2018, 59 (24), 2365–2367. doi:10.1016/j.tetlet.2018.05.020.
- Zheng, Z.; Shi, L. An Efficient Regioselective Copper-Catalyzed Approach to the Synthesis of 1,2,3-Triazoles from N-Tosylhydrazones and Azides. Tetrahedron Lett. 2016, 57 (46), 5132–5134. doi:10.1016/j.tetlet.2016.10.033.
- Ali, A.A.; Konwar, M.; Chetia, M.; Sarma, D. [Bmim]OH Mediated Cu-Catalyzed Azide–Alkyne Cycloaddition Reaction: A Potential Green Route to 1,4-Disubstituted 1,2,3-Triazoles. Tetrahedron Lett. 2016, 57 (50), 5661–5665. doi:10.1016/j.tetlet.2016.11.014.
- Vergara-Arenas, B.I.; Lomas-Romero, L.; Ángeles-Beltrán, D.; Negrón-Silva, G.E.; Gutiérrez Carrillo, A.; Lara, V.H.; Morales-Serna, J.A. Multicomponent Synthesis of 4-Aryl-NH-1,2,3-Triazoles in the Presence of Al-MCM-41 and Sulfated Zirconia. Tetrahedron Lett. 2017, 58 (28), 2690–2694. doi:10.1016/j.tetlet.2017.05.055.
- Reddy, G.S.; Reddy, L.M.; Kumar, A.S.; Ramachary, D.B. Organocatalytic Selective [3+2] Cycloadditions: Synthesis of Functionalized 5-Arylthiomethyl-1,2,3-Triazoles and 4-Arylthio-1,2,3-Triazoles. J. Org. Chem. 2020, 85 (23), 15488–15501. doi:10.1021/acs.joc.0c02247.
- Rajabi-Moghaddam, H.; Naimi-Jamal, M.R.; Tajbakhsh, M. Fabrication of Copper(II)-Coated Magnetic Core-Shell Nanoparticles Fe3O4@SiO2-2-Aminobenzohydrazide and Investigation of its Catalytic Application in the Synthesis of 1,2,3-Triazole Compounds. Sci. Rep. 2021, 11 (1), 1–14. doi:10.1038/s41598-021-81632-7.
- Nandi, D.; Perla, V.K.; Ghosh, S.K.; Arderne, C.; Mallick, K. Copper-azide Nanoparticle: A ‘Catalyst-cum-Reagent’ for the Designing of 5-Alkynyl 1,4-Disubstituted Triazoles. Sci. Rep. 2020, 10 (1), 1–10. doi:10.1038/s41598-020-74018-8.
- Cui, X.; Zhang, X.; Wang, W.; Zhong, X.; Tan, Y.; Wang, Y.; Zhang, J.; Li, Y.; Wang, X. Regitz Diazo Transfer Reaction for the Synthesis of 1,4,5-Trisubstituted 1,2,3-Triazoles and Subsequent Regiospecific Construction of 1,4-Disubstituted 1,2,3-Triazoles via C-C Bond Cleavage. J. Org. Chem. 2021, 86 (5), 4071–4080. doi:10.1021/acs.joc.0c02912.
- Kwok, S.W.; Fotsing, J.R.; Fraser, R.J.; Rodionov, V.O.; Fokin, V.V. Transition-Metal-Free Catalytic Synthesis of 1,5-Diaryl-1,2,3-Triazoles. Org. Lett. 2010, 12 (19), 11–13. doi:10.1021/ol101568d.
- Bahadorikhalili, S.; Ma’mani, L.; Mahdavi, H.; Shafiee, A. Copper Supported Β-Cyclodextrin Functionalized PEGylated Mesoporous Silica Nanoparticle-Graphene Oxide Hybrid: An Efficient and Recyclable Nano-Catalyst for Straightforward Synthesis of 2-Arylbenzimidazoles and 1,2,3-Triazoles. Microporous Mesoporous Mater. 2018, 262, 207–216. doi:10.1016/j.micromeso.2017.11.046.
- Haque, A.; Hsieh, M.F.; Hassan, S.I.; Haque Faizi, M.S.; Saha, A.; Dege, N.; Rather, J.A.; Khan, M.S. Synthesis, Characterization, and Pharmacological Studies of Ferrocene-1H-1,2,3-Triazole Hybrids. J. Mol. Struct. 2017, 1146, 536–545. doi:10.1016/j.molstruc.2017.06.027.
- Aziz Ali, A.; Gogoi, D.; Chaliha, A.K.; Buragohain, A.K.; Trivedi, P.; Saikia, P.J.; Gehlot, P.S.; Kumar, A.; Chaturvedi, V.; Sarma, D. Synthesis and Biological Evaluation of Novel 1,2,3-Triazole Derivatives as Anti-Tubercular Agents. Bioorg. Med. Chem. Lett. 2017, 27 (16), 3698–3703. doi:10.1016/j.bmcl.2017.07.008.
- Jia, F.; Zhang, B. Mechanistic Insight Into the Silver-Catalyzed Cycloaddition Synthesis of 1,4-Disubstituted-1,2,3-Triazoles: The key Role of Silver. New J. Chem. 2019, 43 (22), 8634–8643. doi:10.1039/C9NJ01700C.
- Bao, P.; Yue, H.; Meng, N.; Zhao, X.; Li, J.; Wei, W. Copper-Catalyzed Three-Component Reaction of Alkynes, TMSN3, and Ethers: Regiocontrollable Synthesis of N1- and N2-Oxyalkylated 1,2,3-Triazoles. Org. Lett. 2019, 21 (18), 7218–7222. doi:10.1021/acs.orglett.9b02295.
- Pacifico, R.; Destro, D.; Gillick-Healy, M.W.; Kelly, B.G.; Adamo, M.F.A. Preparation of Acidic 5-Hydroxy-1,2,3-Triazoles via the Cycloaddition of Aryl Azides with β-Ketoesters. J. Org. Chem. 2021, 86 (17), 11354–11360. doi:10.1021/acs.joc.1c00778.
- Chen, J.; Liang, T.; Zhao, H.; Lin, C.; Chen, L.; Zhang, M. Silver-mediated Three-Component Cycloaddition Reaction for Direct Synthesis of 1-N-Vinyl-Substituted 1,2,3-Triazoles. Org. Biomol. Chem. 2019, 17 (19), 4843–4849. doi:10.1039/C9OB00686A.
- Qiu, S.; Chen, Y.; Song, X.; Liu, L.; Liu, X.; Wu, L. Potassium Tert-Butoxide Promoted Synthesis of 4,5-Diaryl-2 H-1,2,3-Triazoles from Tosylhydrazones and Nitriles. Synlett. 2021, 32 (1), 86–90. doi:10.1055/s-0040-1707321.
- Wan, J.P.; Cao, S.; Liu, Y. A Metal- and Azide-Free Multicomponent Assembly Toward Regioselective Construction of 1,5-Disubstituted 1,2,3-Triazoles. J. Org. Chem. 2015, 80 (18), 9028–9033. doi:10.1021/acs.joc.5b01121.
- Engholm, E.; Stuhr-Hansen, N.; Blixt, O. Facile Solid-Phase Ruthenium Assisted Azide-Alkyne Cycloaddition (RuAAC) Utilizing the Cp∗RuCl(COD)-Catalyst. Tetrahedron Lett. 2017, 58 (23), 2272–2275. doi:10.1016/j.tetlet.2017.04.095.
- Xavier, D.M.; Goldani, B.S.; Seus, N.; Jacob, R.G.; Barcellos, T.; Paixão, M.W.; Luque, R.; Alves, D. Sonochemistry in Organocatalytic Enamine-Azide [3+2] Cycloadditions: A Rapid Alternative for the Synthesis of 1,2,3-Triazolyl Carboxamides. Ultrason. Sonochem. 2017, 34, 107–114. doi:10.1016/j.ultsonch.2016.05.007.
- Mondal, P.; Ghosh, S.; Kanti Das, S.; Bhaumik, A.; Das, D.; Islam, S.M. Use of an Efficient Polystyrene-Supported Cerium Catalyst for one-pot Multicomponent Synthesis of Spiro-Piperidine Derivatives and Click Reactions in Green Solvent. Appl. Organomet. Chem. 2018, 32 (4), 1–15. doi:10.1002/aoc.4227.
- Garg, A.; Sarma, D.; Ali, A.A. Microwave Assisted Metal-Free Approach to Access 1,2,3-Triazoles Through Multicomponent Synthesis. Curr. Res. Green Sustainable Chem. 2020, 3, 100013. doi:10.1016/j.crgsc.2020.100013.
- Arafa, W.A.A.; Nayl, A.E.A.A. Water as a Solvent for Ru-Catalyzed Click Reaction: Highly Efficient Recyclable Catalytic System for Triazolocoumarins Synthesis. Appl. Organomet. Chem. 2019, 33 (10), 1–12. doi:10.1002/aoc.5156.
- Sebest, F.; Haselgrove, S.; White, A.J.P.; Díez-González, S. Metal-Free 1,2,3-Triazole Synthesis in Deep Eutectic Solvents. Synlett. 2020, 31 (6), 605–609. doi:10.1055/s-0039-1690736.
- Kumar, N.; Ansari, M.Y.; Kant, R.; Kumar, A. Copper-catalyzed Decarboxylative Regioselective Synthesis of 1,5-Disubstituted 1,2,3-Triazoles. Chem. Commun. 2018, 54 (21), 2627–2630. doi:10.1039/C7CC09934G.
- Chetia, M.; Singh Gehlot, P.; Kumar, A.; Sarma, D. A Recyclable/Reusable Hydrotalcite Supported Copper Nano Catalyst for 1,4-Disubstituted-1,2,3-Triazole Synthesis via Click Chemistry Approach. Tetrahedron Lett. 2018, 59 (4), 397–401. doi:10.1016/j.tetlet.2017.12.051.
- Yamada, M.; Takahashi, T.; Hasegawa, M.; Matsumura, M.; Ono, K.; Fujimoto, R.; Kitamura, Y.; Murata, Y.; Kakusawa, N.; Tanaka, M.; Obata, T.; Fujiwara, Y.; Yasuike, S. Synthesis, Antitumor Activity, and Cytotoxicity of 4-Substituted 1-Benzyl-5-Diphenylstibano-1H-1,2,3-Triazoles. Bioorg. Med. Chem. Lett. 2018, 28 (2), 152–154. doi:10.1016/j.bmcl.2017.11.038.
- Wu, Z.G.; Liao, X.J.; Yuan, L.; Wang, Y.; Zheng, Y.X.; Zuo, J.L.; Pan, Y. Visible-Light-Mediated Click Chemistry for Highly Regioselective Azide–Alkyne Cycloaddition by a Photoredox Electron-Transfer Strategy. Chemistry – A European Journal 2020, 26 (25), 5694–5700. doi:10.1002/chem.202000252.
- Wang, W.; Lin, Y.; Ma, Y.; Tung, C.H.; Xu, Z. Copper(I)-Catalyzed Three-Component Click/Persulfuration Cascade: Regioselective Synthesis of Triazole Disulfides. Org. Lett. 2018, 20 (10), 2956–2959. doi:10.1021/acs.orglett.8b01002.
- Shu, M.; Ran, L.; Liu, K.; Yang, W.; Wang, Q. An Efficient and Regiospecific Synthesis of 1,5-Diaryl-4-Benzothiazolyl-1,2,3-Triazoles by Organocatalytic 1,3-Dipolar Cycloaddition Reactions. Synth. Commun. 2020, 50 (12), 1863–1870. doi:10.1080/00397911.2020.1758144.
- Pokhodylo, N.T.; Tupychak, M.A.; Palchykov, V.A. Dihydro-2H-thiopyran-3(4H)-one-1,1-dioxide–a new Cyclic Ketomethylenic Reagent for the Dimroth-Type 1,2,3-Triazole Synthesis. Synth. Commun. 2020, 50 (12), 1835–1844. doi:10.1080/00397911.2020.1757113.
- Kafle, A.; Bhattarai, S.; Handy, S.T. An Unusual Triazole Synthesis from Aurones. Synthesis (Germany) 2020, 52 (16), 2337–2346. doi:10.1055/s-0040-1708019.
- Vroemans, R.; Horsten, T.; Van Espen, M.; Dehaen, W. 5-Formyltriazoles as Valuable Starting Materials for Unsymmetrically Substituted Bi-1,2,3-Triazoles. Front. Chem. 2020, 8 (April), 5–10. doi:10.3389/fchem.2020.00271.
- Silaichev, P.S.; Beryozkina, T.V.; Novikov, M.S.; Dehaen, W.; Bakulev, V.A. A Base-Controlled Reaction of 2-Cyanoacetamidines (3,3-Diaminoacrylonitriles) with Sulfonyl Azides as a Route to Nonaromatic 4-Methylene-1,2,3-Triazole-5-Imines. Eur. J. Org. Chem. 2020, 2020 (24), 3688–3698. doi:10.1002/ejoc.202000453.
- Yang, H.; Zeng, T.; Xi, S.; Hu, S.; Wu, Y.; Tang, Y. Photoinduced, Strain-Promoted Cycloadditions of: Trans-Cycloheptenones and Azides. Green Chem. 2020, 22 (20), 7023–7030. doi:10.1039/D0GC02347G.
- Abdalkareem Jasim, S.B.; Mohammed, D.; Turki Jalil, A.; Smaisim, G.F.; Shareef Mohsen, K.; Abed Hussein, S.; Shafik, M.-S. An Efficient and Attractive Synthetic Protocol for Three-Component Preparation of NH-1,2,3-Triazoles Using a Novel Magnetically Recoverable Copper Catalyst. Polycyclic Aromat. Compd. 2023, 1–21. doi:10.1080/10406638.2023.2167217.
- Mazur, O.M.; Zhelavskyi, S.; Zviagin, E.M.; Shishkina, V.S.; Musatov, I.V.; Kolosov, A.M.; Shvets, E.H.; Andryushchenko, A.; Chebanov, V.A. Effective Microwave-Assisted Approach to 1,2,3-Triazolobenzo-Diazepinones via Tandem Ugi Reaction/Catalyst-Free Intramolecular Azide–Alkyne Cycloaddition. Beilstein J. Org. Chem. 2021, 17, 678–687. doi:10.3762/bjoc.17.57.