ABSTRACT
Indole moiety is widely present in several natural bioactive products and medicinally important compounds. It is an essential scaffold for the development of novel leads. Nowadays, the synthesis of indoles by conventional methods is replaced with greener ones. The dynamic growth in green organic synthetic approaches for different heterocyclic scaffolds has markedly contributed to the field of medicinal chemistry over the last few decades. This review article compiled the last seven years’ results in the green synthesis of indole and its derivatives using microwave irradiations from 2018 to 2024. Microwave (MW) assisted reactions are very rapid, environment-friendly, proficient, and convenient methodologies for the synthesis of organic compounds. This review contains synthetic procedures, reaction conditions, and greener features in synthesizing indole and its derivatives. This review article aims to promote greener synthesis methods and guide the researchers to design, develop, and synthesize indole-based derivatives using a microwave irradiation technique. This article also discusses the anticancer potential of indole-containing compounds reported in recent literature.
1. Introduction
The name Indole was first given by Baeyer and Knop in 1866. Indole was first prepared synthetically by Baeyer (Citation1). This ring is the most abundant and significant heterocycles present in nature. Both synthetic and natural indoles are of biological importance (Citation2). Indole (C8H7N) is a weakly basic molecule which consists of a pyrrole ring fused to a benzene nucleus, and ten π electrons move throughout the structure. Delocalization of a lone pair of nitrogen into the free circulation of the π electronic system is responsible for the basicity of the indole nucleus (Citation3). Indole is a privileged scaffold having outstanding pharmacological activities acting through different mechanisms. reports important marketed drugs having indole rings with various biological activities (Citation4).
Different methods for synthesizing Indole have been described in the literature, including Fischer Indole Synthesis, Cyclization of 2-Alkynylanilines, Reductive Cyclizations, Heteroannulations, and other miscellaneous processes. shows some of the common methods for the conventional synthesis of Indole (Citation5).
Nowadays, academic researchers, as well as pharmaceutical industries, are using green approaches for the synthesis of indole-containing compounds to overcome the shortcomings associated with conventional methods. Different greener methods involve the use of ultrasound, multicomponent reaction, microwave irradiations, deep-eutectic liquids, ionic liquids, water, green solvent, nanocatalysts, green catalysts, and solvent-free reactions etc. Green synthesis is an eco-friendly technique which is designed to exclude toxic waste, to use ecological solvents and to reduce energy consumption (Citation6). ‘green’ chemistry aims to develop technologies for more efficient chemical reactions and to obtain the products safely (Citation7). There are 12 principles of green chemistry (Citation8), as depicted in . This review reflects the last seven years’ advances in the green synthesis of indole and its derivatives using microwave irradiations.
2. Microwave irradiations (MWI):
Microwave irradiation uses high-frequency electromagnetic waves and is commonly used in organic synthesis (Citation9). Microwave irradiation is commonly utilized as an effective heating source in synthetic reactions, providing rapid and targeted heating (Citation10). The use of microwave-assisted techniques has brought a revolution in the field of manufacturing and chemical sciences (Citation11). It shortens the reaction time for synthesis from several hours to a few minutes only by microwave irradiation (Citation12). The advantages of microwave synthesis include short reaction times, solventless reactions, higher yields, lower energy consumption, and, consequently, ideal for optimization processes (Citation13). Under the influence of microwave irradiation, chemical reactions accelerate due to the absorption of microwaves by polar organic molecules. This enables reactions that typically don't occur with conventional heating to take place (Citation14). The synthesis of indole derivatives using MWI in the presence or absence of catalysts, solvents, and solvent-free conditions in recent years is discussed below:
3. Synthesis of indole nucleus using microwave irradiation
Yao et al. synthesized different indole derivatives 1 using Fischler indole synthesis by stirring a mixture of α-amino aryl ethanone (0.6 mmol) using 1,1,1,3,3,3-Hexafluoropropan-2-ol (HFIP 3 mL) as solvent at 120°C under MWI for 40 min. When the reaction was complete, the crude reaction mixture was diluted with Ethanol (5 mL) and filtered using a short pad of celite to obtain the product. The synthetic scheme and green features are shown in , and the product is obtained from moderate to high yield (Citation15).
Rode et al. used microwave irradiation for the conversion of β-(2-aminophenyl)-α,β-ynones derivatives (0.22 mmol) into 2-acyl indoles 2 using AgOTf or CuOTf (20 mol %) as a catalyst. The reaction mixture was exposed to MWI for 15 min at 140°C, and TLC checked reaction completion. A selective anti-Michael intramolecular hydro amination was used, and the first example of 5-exo-dig cyclization of 2-alkynyl anilines having an acyl group on the triple bond was reported, as shown in (Citation16).
Zhao et al. demonstrated that 2-alkynyldimethylamines (0.5 mmol) on stirring in ethanol (3 ml) at 120°C under MWI easily cyclize following 5-endo-dig annulation to give 2-substituted indoles 3. This reaction activates the triple bond even in the absence of metal catalysts or any additives (Citation17). The synthetic scheme and green features of the reaction are shown in .
Fujita et al. synthesized 3-(Trifluoromethyl) indoles using o-sulfonamido-α-(trifluoromethyl) styrenes (0.20 mmol), t-BuOH (2.0 mL) and cerium (IV) ammonium nitrate (CAN) (0.600 mmol). The mixture was heated under microwave irradiation at 100°C and 60 W for 3 min to obtain the derivatives 4 in high yield (Citation18). The scheme is shown in .
Figure 7. Schematic representation for synthesizing 3-(Trifluoromethyl) indole derivatives by Fujita et al.
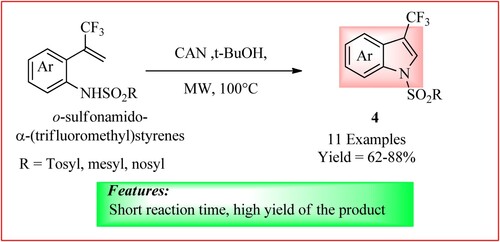
Cirillo et al. used a heated sealed-vessel reactor similar to a microwave reactor to synthesize Indole by Fischer. Phenyl hydrazine hydrochloride salt (1.00 mmol), Dehydroepiandrosterone (DHEA) acetate (1.00 mmol) is taken, and Glacial acetic acid (2 mL) is then added, which forms a suspension. After this tube is sealed, swirled, and heated to 160°C for 20 min. The product 5 was obtained in high yield (Citation19). The reaction is shown in .
Figure 8. Schematic representation for synthesizing Indole derivative by Cirillo et al. 1,2,3-triazole derivatives by Mokariya et al.
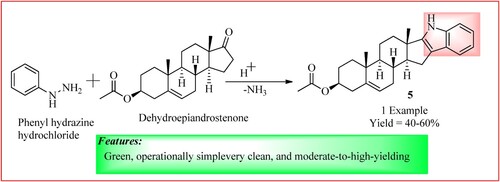
Bellavita et al. synthesized 2-Methyl-1H-indole-3-carboxylate derivatives by reacting enamine intermediate (0.16 mmol), Cu(OAc)2 (0.016 mmol), Pd(OAc)2 (10% mol), K2CO3 (0.4 mmol) dissolved in DMF (2 Ml) in a microwave reaction vessel, under argon atmosphere. Stirring was done at 60°C under MWI to get the final product 6, as shown in (Citation20).
Figure 9. Schematic representation for synthesizing 2-Methyl-1H-indole-3-carboxylate derivatives by Bellavita et al.
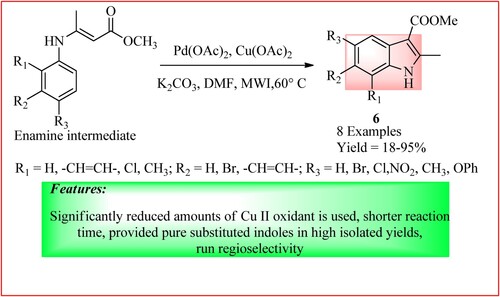
2-Aryl indoles 7 and some fused indole derivatives 8 were synthesized by Mishra et al. by the condensation reaction between 2-(4- Hydrazino-phenyl)-ethanesulfonic acid methylamide hydrochloride (0.11 mmol) and aryl ketones (0.13 mmol) in the presence of ethanol and acetic acid was used as a catalyst at room temperature. The reaction mixture was then MW irradiated for 10 min at 80°C, 300 W, as shown in (Citation21).
4. Synthesis of indole derivatives
Rathod and Biradar developed a green procedure for synthesizing pyran-annulated indole analogs (9, 10, and 11) via a one-pot, three-component reaction. In their synthetic method, they reacted a mixture of malononitrile (0.01 mmol), 2,5-disubstituted indole-3-carboxaldehyde (0.01 mmol) with and different phenols (0.001 mmol) like α-naphthol, 8-hydroxyquinoline, 4-hydroxycoumarin which were then combined with powdered catalyst KNaC4H4O6·4H2O (10 mol %) and powdered 5 Å molecular sieves (0.5–1.0 g). The mixture was irradiated using MW (350–450 W) for 5–6 min loaded into an open borosil glass vessel, and was subjected to MW irradiation at 125–150°C for 10 min to obtain compounds 1, 2, and 3 in high yield. The synthetic scheme and green features of the reaction are shown in (Citation22).
Figure 11. Schematic representation for the synthesis of pyran-annulated indole analogs by Rathod and Biradar.
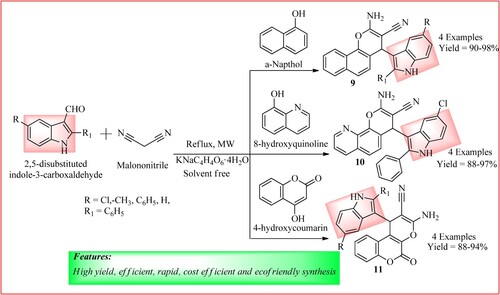
Baharfar et al. synthesize Indole–dihydrofuran biheterocycles by microwave irradiation of a mixture containing substituted 3-cyanoacetyl Indole (1.0 mmol), substituted N-phenacylpyridinium bromide (1.0 mmol), potassium carbonate (1.0 mmol) and aromatic aldehyde (1.0 mmol) in water (3.0 mL) at 180 watts. This one-pot, three-component microwave-assisted synthesis of derivatives 12 is shown in . The products were obtained with high yields ranging from 85 to 98% (Citation23).
Figure 12. Schematic representation for synthesizing Indole–dihydrofuran biheterocycles by Baharfar et al.
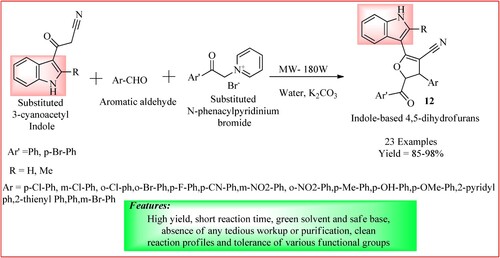
A series of novel spiro [indole-thiazolidines] compounds were synthesized from Compound 13, obtained through different reaction protocols. As shown in , Compound 14 was obtained using the green synthesis method in which a solution of thioglycolic acid (0.1 mmol/1 eq.) and compounds 5 (0.1 mmol/1 eq.) was taken in DMF (5 ml) and catalyst ZrSiO2 (10 mol%) were added. After this, the mixture was exposed to MWI for 9–12 min at 120°C to obtain the product in high yield (Citation24).
Figure 13. Schematic representation for synthesizing novel spiro[indole-thiazolidines] derivatives by Borad et al.
![Figure 13. Schematic representation for synthesizing novel spiro[indole-thiazolidines] derivatives by Borad et al.](/cms/asset/b2c2c582-1fba-4ad4-8c8b-8f9b59cfb29f/tgcl_a_2362925_f0013_oc.jpg)
Malkania et al. synthesized bis (indolyl) methane analogs 15 by reacting indole (0.0463 moles) and desired aldehyde (0.0132 moles), which was charged with water (10 ml) and lactic acid in a catalytic amount. The reaction mixture was irradiated under MWI at 240 W for the specified time, and the procedure is shown in . The reaction mixture was cooled every 1 min of the MW exposure, and the TLC method was used to check the progress of the reaction. It was concluded that an electron-withdrawing group fastened the reaction, and an electron-donating group slowed down the reaction (Citation25).
Bankar synthesized a functionalized nanocat-ferrite-L-cysteine catalyst used in bis(indolyl) methanes 16 syntheses. A mixture of indole (2 mmol), carbonyl compound (1 mmol), and nanocat-Fe-cys (100 mg) and exposed under MWI at 350 W with stirring to get the desired product in high yield. The synthetic scheme and green features of the reaction are shown in (Citation26).
Denya et al. synthesized a series of indole derivatives 18. Compound 17 was synthesized using different reaction protocols, and its position N1 was conjugated with propargyl bromide. For this compound 10 (1 mmol), propargyl bromide (1.2 mmol) in dimethylformamide (10 ml) was stirred in the presence of NaH (1 mmol) at room temperature and was exposed to MWI (95°C, 150 W) for 2–4 h to obtain the desired product (Citation27). The synthetic scheme and green features of the reaction are shown in .
Minkovska et al. developed squaraine dye using a novel greener approach. As shown in , 1, 3, 3-trimethyl-2-methyleneindoline (10 mmol) in ethyl l-lactate (10 mL) and squaric acid (5 mmol) were charged and exposed to MWI at 140°C and 450 W. It was observed that the reagents dissolved and turned blue after 1 min of MWI. The reaction was carried out for 4 min at the same condition to get the required dye 19. The synthetic scheme and green features of the reaction are shown in (Citation28).
Shaikh et al. reported the synthesis of novel fluorinated indole derivatives 20 using MWI. A mixture of 5-fluoroindoline-2, 3-dione (0.01 mol) and various anilines (0.01 mol) was taken in ethanol (5 mL), and copper dipyridine dichloride CuPy2Cl2 (5 mol %) catalyst was added in it. This mixture was charged into a microwave pressure vial (10 mL) and irradiated at 60°C with 200 W power. The products were obtained with good yield (Citation29). The synthetic scheme and green features of the reaction are shown in .
The ‘Click chemistry’ approach was used by Singh et al. for the synthesis of novel indole acetylene Schiff base appended 1, 2, 3- triazole sensor in a two-step procedure. Compounds 21 and 22 were prepared by irradiating a mixture of acteylenic indole appended Schiff base (AISB) (1 equiv) with ϒ-azidopropyltriethoxysilane (AzPTES) (1 equiv) and catalyst [CuBr (PPh3)3] (0.01 mmol) with microwave using one-pot synthesis. 86–98% yield of the products was obtained in 15 min reaction time (Citation30). The synthetic scheme and green features of the reaction are shown in .
Figure 19. Schematic representation for synthesizing novel indole acetylene Schiff base appended 1, 2, 3- triazole by Singh et al.
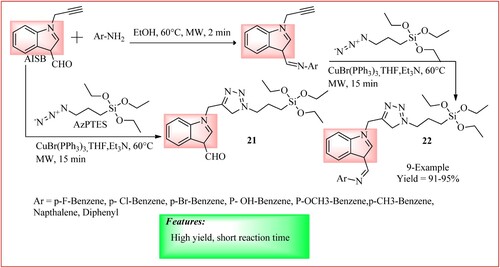
Lee et al. explained an MW-assisted Pictet–Spengler type condensation reaction for synthesizing benzimidazole-linked indoline hybrids 23. The reaction was carried out between C-2-linked o-aminobenzylbenzimidazole (1.48 mmol), aldehydes or ketones (4.44 mmol) and 10 mol% of acetic acid in 4 mL acetonitrile (MeCN) under microwave radiation at 140°C for 30 min. Oxidation of the obtained benzimidazole-linked indoline hybrids with 2,3-dichloro-5,6-dicyano-1,4-benzoquinone (DDQ) generated the corresponding benzimidazole-linked indole hybrids (Citation31) as shown in .
Patel et al. synthesize 3-(3-oxoaryl) indole derivatives 24 using microwave-assisted Michael addition. The reaction was carried out by irradiating a mixture of 2-phenylindole (0.518 mmol) and different chalcone (0.518 mmol) in the presence of 15 mol% of Lewis acid catalyst ZrCl4 (0.078 mmol) under the solvent-free condition at 280 W. The product was obtained at a high yield (Citation32). The synthetic scheme and green features of the reaction are shown in .
Figure 21. Schematic representation for synthesizing b 3-(3-oxoaryl) Indole Derivatives by Patel et al.
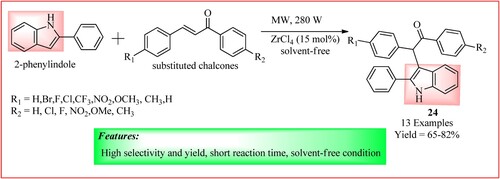
Bakkolla et al. synthesized novel indole derivatives containing cyclic imide and pyrimidine scaffolds by reacting a mixture of compound 25 (0.1 mmol) and phthalic anhydride/ maleic anhydride (0.1 mmol) irradiated under microwave at reaction condition as shown in to get derivatives 26 and 27 with a high yield of 90–92% (Citation33).
Figure 22. Schematic representation for synthesizing novel indole-pyrimidine-cyclic imide derivatives by Bakkolla et al.
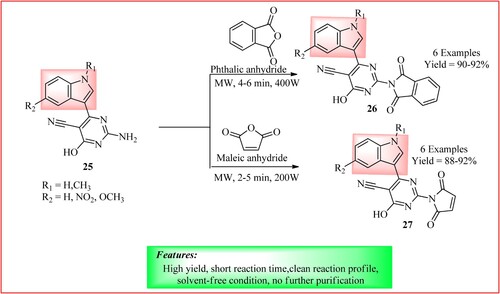
Wu et al. developed a Lewis acid–surfactant–SiO2-combined (LASSC) nanocatalyst, which combines AlCl3·6H2O, SDS, and SiO2. In their reaction, Indole (2.0 mmol), aromatic benzaldehyde (1.0 mmol), and LASSC catalyst (0.5 g) were used with grinding under microwave irradiation for 20 min to yield bis(indolyl)methanes 28 and 29 as the product and were obtained in high yield. The scheme is shown in (Citation34).
Modi and Jain synthesized 3-methyl-1-phenyl-4- ((2-phenyl-1H-indol-3-yl) methylene) -1H-pyrazole -5 (4H) -ones 30 by mixing thoroughly 3-methyl-1 -phenyl-2- pyrazoline-5-one (3 mmol), 2-phenyl-1H-indole-3-carbaldehyde (3 mmol), and montmorillonite KSF (0.4 g) in a mortar and pestle and irradiated with microwaves in a conical flask for 5–11 min in a domestic microwave (800 W, 2450 MHz) (Citation35). The scheme is shown in .
Figure 24. Schematic representation for 3-methyl-1-phenyl-4- ((2-phenyl-1H-indol-3-yl) methylene) -1H-pyrazole -5 (4H) -ones by Modi and Jain.
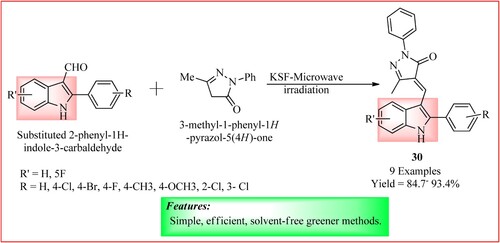
Hote et al. synthesized bis (indolyl)-methanes 31 from the condensation of indole (2 mmol) with various substituted aromatic aldehydes (1 mmol) and was irradiated with visible light at 150W using a tungsten lamp. The product is obtained in a high yield of 81–91% (Citation36). The reaction is shown in .
Rathod et al. synthesized isoniazid derivatives from a reaction of various 2, 5-disubstituted indole-3-carbaldehyde (1 mmol), different phenols (1 mmol), and isoniazid (1 mmol) in hot ethanol (5 ml) using Betti’s reaction. This reaction was carried out under solvent-free and one-pot green reaction conditions using an MW oven heated at 125°C for 5–6 min to get the derivatives 32, 33, and 34 in high yield (Citation37). The synthetic scheme and green features of the reaction are shown in .
Figure 26. Schematic representation for synthesizing indole-containing isoniazid derivatives by Rathod et al.
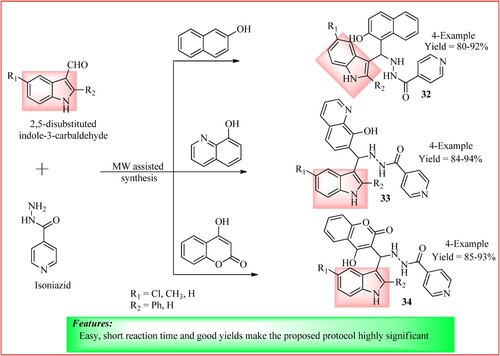
Elkamhawy et al. synthesized N-substituted indole-based analogs using microwave irradiations. Firstly compound 35 was synthesized in which a solution of 5-nitroindole (1, 162.2 mg, 1.0 mmol) in DMF (11 mL) at 0°C was taken, and NaH (60% in oil, 48.0 mg, 1.2 mmol) was added in it and stirring was continued for 10 min at 0°C. The suitable reagent (1.0 mmol; 4-fluorobenzyl bromide/ 2-(chloromethyl) pyridine hydrochloride /and 3-fluorobenzoyl chloride) was added to the mixture and stirred for 24 h at 100°C and cooled back to room temperature. It was then partitioned between water and Ethyl acetate using aqueous sodium bicarbonate. The organic layer was collected and dried using anhydrous sodium sulfate and then concentrated under reduced pressure. This compound 35 (1.0 mmol) was then dissolved in EtOH/H2O (5/2), and then iron powder (558.5 mg, 10.0 mmol) and ammonium chloride (267.5 mg, 5.0 mmol) were added and refluxed for 1 h. Filtration on celite and washing with EtOH give compound 36. Compound 32 (0.1 mmol), DIPEA (0.05 mL, 0.27 mmol), HATU (41.8 mg, 0.11 mmol), the appropriate heteroaryl carboxylic acid derivative (0.11 mmol) and DMF (12 mL) were taken in a sealed MW vial at room temperature and heated under MW at 116°C for 45 min. EA, water, and brine were used to extract the mixture to get compound 37. Compound 38 was synthesized by taking a solution of 5-nitroindole (1, 162.2 mg, 1.0 mmol) in DMF (5 mL), in which potassium carbonate (55.3 mg, 0.24 mmol) was added. Dimethyl carbonate (DMC) (0.17 mL, 2.0 mmol) was then added and refluxed for 3 h. After this, the mixture was cooled to 4–5°C and added to ice-cold water. Compounds 39 and 40 were synthesized using the same reaction protocol as compounds 36 and 37. The whole procedure of synthesis is shown in (Citation38).
Figure 27. Schematic representation for synthesizing N-substituted indole-based analogs by Elkamhawy et al.
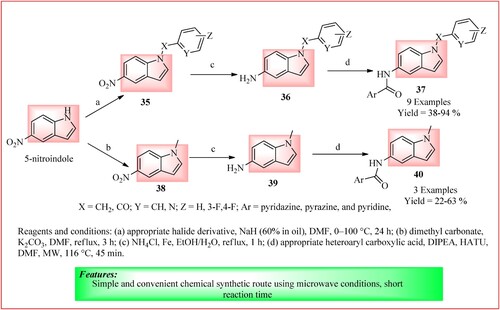
Duguta et al. performed acetylation and formylation of indole using green Vilsmeier –Haack (VH) reagents. Freshly prepared Trichlorotriazine adducts with DMF and DMA (TCTA/DMF, TCTA/DMA, TCCA/DMF and TCCA/DMA) were taken (10 mmol) and loaded in Microwave reaction vials with indoles (10 mmol), and placed in a lab microwave oven on a silica gel solid support (500 mg) to obtain the derivative 41 (Citation39). The synthetic scheme and green features of the reaction are shown in .
Gohain and Thakur developed Au nanoparticles (NPs) by using the aqueous fruit extract of Garcinia cowa at room temperature without any external stabilizing agent. These Au NPs were used as catalysts in synthesizing bis(indolyl)methanes 42 via microwave-aided coupling of substituted indole (2 mmol) and aromatic aldehydes (1 mmol). This coupling reaction was more sustainable and occurred only in 40 s (Citation40). The synthetic scheme and green features of the reaction are shown in .
Taheri et al. used Fe3O4@rGO@ZnO-HPA magnetic core–shell nanoparticles (MCNPs) for the novel and efficient synthesis of benzo[α]furo[2, 3-c]phenazine derivatives. An intermediate was synthesized due to tautomerism of 1, 4-dione, which then condensed with a diamine to produce benzo[a]phenazine-5-ol. The indole and aryl-glyoxal condensed to produce the open shaft at the same time. The C–C and C–N bonds are formed due to intermolecular [3 + 2] reactions, and finally, the loss of water yields the derivative 43. The synthetic scheme and green features of the reaction are shown in (Citation41).
Figure 30. Schematic representation for synthesizing Indole containing benzo[α]furo[2, 3-c]phenazine derivatives by Taheri et al.
![Figure 30. Schematic representation for synthesizing Indole containing benzo[α]furo[2, 3-c]phenazine derivatives by Taheri et al.](/cms/asset/7e114c0e-cd59-4967-90bf-0d48f36c19b8/tgcl_a_2362925_f0030_oc.jpg)
Gampa et al. synthesized indolyl-4H-chromene derivatives 44 using a new one-pot three-component reaction. They reacted equal moles of (E)-N-methyl-1-(methylsulfanyl)-2-nitroethenamine, salicylaldehydes, and indoles using triethylamine as a catalyst without any solvent. This leads to the formation of an indole-substituted chromene ring as two C–C bonds and one C–O bond were synthesized in a single operation. The synthetic scheme and green features of the reaction are shown in (Citation42).
Figure 31. Schematic representation for synthesizing indolyl-4H-chromene derivatives by Gampa et al.
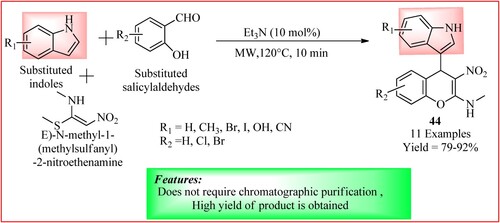
Luna-Mora et al. synthesized 4-[bis(1H-indol-3- yl)methyl]phenyl}-1H-benzimidazole or 1-{3- or 4-[bis(1H-indol-3-yl)methyl]benzyl}-2-{3- or derivatives. Firstly, compound 45 was synthesized using multicomponent reactions by reacting 1H-indole (2 mmol) with either isophthalaldehyde or terephthalaldehyde (1 mmol) under microwave irradiation. In another step, a mixture of o-phenylenediamine (1.00 mmol) and 3/or (4- formylphenyl)diindol-3-ylmethane 45 (2.00 mmol) were blended thoroughly in a microwave vial (20 ml) and irradiated in a monowave 300 microwave reactor at for 3 min at 195°C to obtain the final compound 46. The reaction is shown in (Citation43).
Figure 32. Schematic representation for synthesizing 1-{3- or 4-[bis(1H-indol-3-yl)methyl]benzyl}-2-{3- or 4-[bis(1H-indol-3- yl)methyl]phenyl}-1H-benzimidazole derivatives by Luna-Mora et al.
![Figure 32. Schematic representation for synthesizing 1-{3- or 4-[bis(1H-indol-3-yl)methyl]benzyl}-2-{3- or 4-[bis(1H-indol-3- yl)methyl]phenyl}-1H-benzimidazole derivatives by Luna-Mora et al.](/cms/asset/c0b9fead-6eb7-4e94-bdd1-b329c1c83fcd/tgcl_a_2362925_f0032_oc.jpg)
Kaur et al. reported a series of 1, 4-dihydropyrazolo [4, 3-b] indoles 47 following modified Cadogan condition in which deoxygenation of o-nitrophenyl-substituted N-aryl pyrazoles occurs, leading to the formation of subsequent intramolecular (sp2)-N bond under MWI. This method leads to the formation of N-substituted as well as NH-free fused indoles. The synthesis proceeded in a microwave vial in which PPh3/P(OEt)3 (3 equiv), pyrazole-based nitro compound (1 equiv), and toluene (5 mL) were mixed and stirred at 180/210°C in the CEM (sealed tube) to yield the final product 43 as shown in (Citation44).
Figure 33. Schematic representation for synthesizing 1,4-dihydropyrazolo[4,3-b]indoles by Kaur et al.
![Figure 33. Schematic representation for synthesizing 1,4-dihydropyrazolo[4,3-b]indoles by Kaur et al.](/cms/asset/1bc9cf81-94ab-45ea-85b1-6073ebdf8351/tgcl_a_2362925_f0033_oc.jpg)
Nirwan and Pareek synthesized substituted 3-(4,5-diphenyl-1H-imidazol-2-yl)-1H-indole derivatives 48. A greener one-pot four components approach was in which indole-3-carbaldehyde (1 mmol), ammonium acetate (1 mmol), benzil (1 mmol), and different amines (1 mmol) were condensed under microwave irradiation for 8–15 min at 80°C using Amberlyst A-15 (0.12 g) as a recyclable catalyst as shown in (Citation45).
Figure 34. Schematic representation for synthesizing substituted 3-(4, 5-diphenyl-1H-imidazol-2-yl)-1H-indole and substituted 5-bromo-3-(4,5-diphenyl-1H imidazol-2-yl)-1H-indole by Nirwan and Pareek.
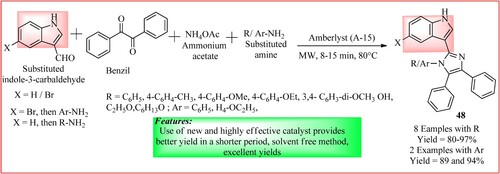
Hassan et al. synthesized 1, 3, 3-tri (1H-indol-3-yl) propan-1-one 49 using pure citrus lemon juice as catalyst. This compound was further used as a precursor for the synthesis of oxoketene gem-dithiol and 1,2-dithiole-3-thione. For synthesizing compound 45, Indole (0.005 mol) and chalcone (0.005 mol) were alkylated directly by dissolving in pure lemon juice (7 mL) followed by 3 min MWI. The product is obtained in a high yield of 99% (Citation46). The synthetic scheme and green features of the reaction are shown in .
Figure 35. Schematic representation for synthesizing 1,3,3-tri(1H-indol-3-yl)propan-1-one by Hassan et al.
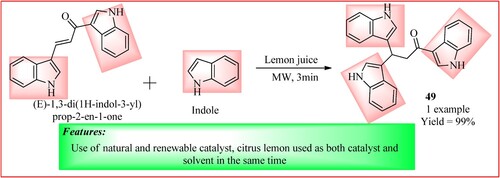
Singh et al. used 1,1,1,3,3,3-Hexafluoroisopropanol (HFIP) as both catalyst and solvent for synthesizing diversified pyrazinoindoles, which was synthesized using a modified Pictet–Spengler reaction. Compound 50 was synthesized, as shown in . For the synthesis of compound 51, they took compound 50 (1.038 mmol) in a microwave vial and charged it with HFIP (1 mL). After this, aryl aldehydes (1.038 mmol) were added to it, and the reaction was carried out for 40 min at 60°C to obtain the final pyrazinoindoles (Citation47).
Mokariya et al. took a mixture of 2-substituted-1-(prop-2-yn-1-yl)-1H-indole-3-carbaldehyde, 2–chloroethylacetate/2–Chloroacetic acid, and sodium azide in equimolar quantity and dissolved them in DMF containing powdered copper iodide (5 mol%). The mixture was mixed thoroughly and subjected to ultrasonication (28 kHz, 70 W) and microwave irradiation (100 w for 15 min) to get the final product 52 (Citation48). The synthetic scheme and green features of the reaction are shown in .
Figure 37. Schematic representation for synthesizing 3-formyl-indole clubbed 1,4-disubstituted-1,2,3-triazole derivatives by Mokariya et al.
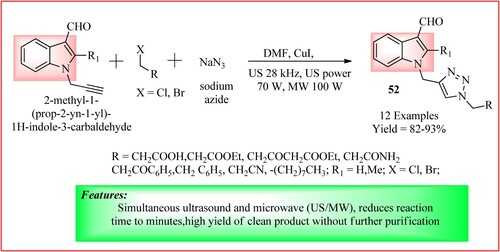
Rajesab et al. synthesized novel indole analogs 53 using MWI. They took a sample crucible and added a solution substituted indole aldehyde (0.05 mol), 2-(5-bromo-3-phenyl-1H-indole-2-carbonyl) hydrazinecarbothioamide (0.01 mol), ethyl acetoacetate (0.01 mol) along with a catalytic amount of MK10 Clay. The mixture was microwave irradiated at 70% microwave intensity for 8–10 min (Citation49). The synthetic scheme and green features of the reaction are shown in .
Wang et al. used a one-pot three-component reaction to synthesize a series of indole-substituted 54 and spiroxindole-consisted dihydropyrido[2,3-d]pyrimidine derivatives 55. They carried the reaction between 2,6-diaminopyrimidin-4-one and 3-cyanoacetyl indoles. For the synthesis of 51 various aryl aldehydes and the synthesis of 52, isatins were used under microwave irradiation, as shown in (Citation50).
Figure 39. Schematic representation for synthesizing indole substituted or spiroxindole-consisted dihydropyrido[2,3-d]pyrimidine derivatives by Wang et al.
![Figure 39. Schematic representation for synthesizing indole substituted or spiroxindole-consisted dihydropyrido[2,3-d]pyrimidine derivatives by Wang et al.](/cms/asset/80b12f19-56d1-4e8e-93e7-e6ffb68cf8f6/tgcl_a_2362925_f0039_oc.jpg)
An efficient one-pot three-component reaction for the synthesis of 2,4-diamino-5-(1H-indol-3-yl)-5H- chromeno[2,3-b]pyridine-3-carbonitrile derivatives has been developed by Avula et al. A mixture of substituted indoles, salicyaldehydes, and 2-aminopropene-1,1,3-tricarbonitrile was taken and trimethylamine was added as catalyst. This mixture was then MWI at 100°C to synthesize the target compound 56 (Citation51). The synthetic scheme and green features of the reaction are shown in .
Figure 40. Schematic representation for synthesizing Indolyl-5H- chromeno[2,3-b] pyridine derivatives by Avula et al.
![Figure 40. Schematic representation for synthesizing Indolyl-5H- chromeno[2,3-b] pyridine derivatives by Avula et al.](/cms/asset/98f2c411-d758-4c6e-b5d1-fa78b27f96df/tgcl_a_2362925_f0040_oc.jpg)
Rivas-Loaiza et al. synthesized Bis(indolyl)methane derivatives using two methods. In the first method, they used indole (2 equivalents), substituted imine (1 equivalent) and organocatalysts (20 mol%), which were stirred for 24 h at 70°C to yield the product 57. In the second method, they used indole (2 equivalents) and corresponding aldehydes (1 equivalent) and added organocatalyst (10 mol%). The mixture was exposed to microwave irradiation with 100 W power at 150°C for 5–30 min, yielding bisindolyl derivatives. The scheme is shown in (Citation52).
Figure 41. Schematic representation for synthesizing Bis(indolyl)methane derivatives b Rivas-Loaiza y et al.
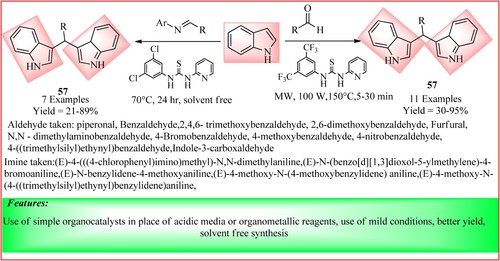
Al-Ajely synthesized a novel set of hippuric hydrazones bearing indole moieties in three steps following the protocol of microwave irradiation and solvent-free conditions. Hippuric hydrazide (1 mmol) and 1H-indole-6-carbaldehyde (1 mmol) were taken in a microwave vial which was irradiated and heated to 45°C (17 bar) for 5 min and then cooled at ambient temperature to obtain the product 58 which is N-(2-(2-((1H-indol-6-yl)methylene)hydrazineyl)-2-oxoethyl)benzamide as shown in (Citation53).
Figure 42. Schematic representation for synthesizing N-(2-(2-((1H-indol-6-yl)methylene)hydrazineyl)-2-oxoethyl)benzamide by Al-Ajely.
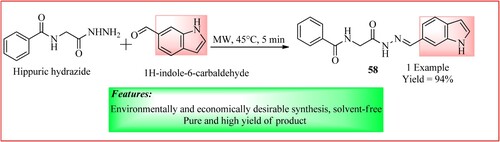
Katowah et al. synthesized novel spiro pyrrolizidine-oxinidole moieties using ultrasound and microwave irradiations. A mixture of isatin (1 mmol), amino acids such as sarcosine or L-proline (1 mmol) and 3,5-bis[arylmethylidene]-4-oxipiperidine-N-ethyl (0.5 mmol) were taken and exposed to ultrasound irradiation for 30 min at 25–30°C or to microwave irradiation at ambient temperature for 10 min to obtain the final product 59 and 60 as shown in (Citation54).
Figure 43. Schematic representation for synthesizing spiro pyrrolizidine-oxinidole derivatives by Katowah et al.
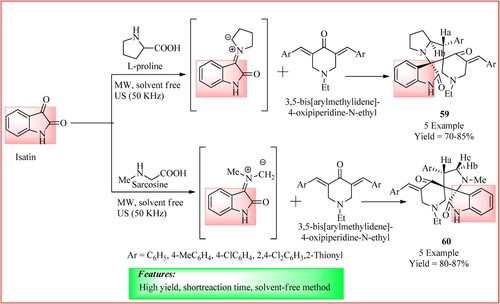
Vijayasaradhi et al. synthesized Indole-3-sulfonamides 61 using Sc(OTf)3 (50 mol%) as a preferred Lewis acid. This Lewis acid was used in a two-step, one-pot synthesis of primary sulfonamide derivatives of indole and N-methyl indole in which Burgess Reagent (tert-butoxy derivative) was used. The reagent and reactions are shown in (Citation55).
Figure 44. Schematic representation for synthesizing Indole-3-sulfonamides derivatives by Vijayasaradhi et al.
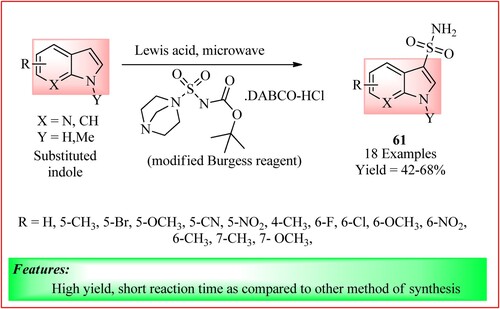
Sahu et al. developed a novel series of Cephalandole A analogs 62 using a p-Toluenesulfonic acid (PTSA) catalyst under microwave irradiation conditions. The mixture of 2-(1H-indol-3-yl)-2-oxoacetic acid and aniline were taken in equal moles and was dissolved in acetonitrile at 90°C. The reaction was irradiated for 15 min and the products were obtained in high yield (Citation56) as shown in .
Chudasama et al. described the microwave-assisted one-pot synthesis of 3-imidazolyl indole combined with 1,2,3-triazole hybrids 63, which exhibit potential as antiproliferative agents. In a 50 mL round bottom flask equipped for microwave heating (set at 100°C and 200 W), 1.0 mmol of N-propargyl-indole-3-carbaldehyde derivatives, along with 1.0 mmol of 2,3-diaminomaleonitrile, 1.2 mmol of azide derivatives, and 5 mol% of CuI in PEG-4000 (2.0 mL) were combined. TLC was used to monitor the reaction. Upon completion of the reaction (15–18 min), the mixture was poured into 50 mL of ice-cold water with continuous stirring. Yellow precipitates were then collected via gravity filtration and washed with ethanol to isolate the desired product. The products were obtained in high yield (Citation57) and the synthetic scheme is shown in .
Figure 46. Schematic representation for synthesizing 3-imidazolyl indole combined with 1,2,3-triazole hybrids by Chudasama et al.
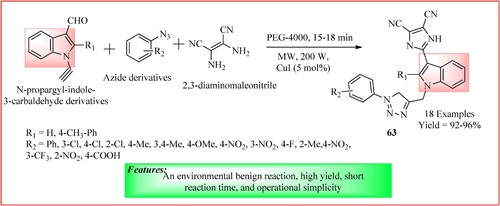
Lateef et al. documented the synthesis of Indol-3-yl-4H-pyran derivatives 64 utilizing a zinc-linked amino acid complex [Zn(L-proline)2] as a green catalyst. In a beaker, a mixture comprising a solution of indole-3-carbaldehyde (1 mmol), malononitrile (1 mmol), a nucleophile (1 mmol), and Zn(L-proline)2 catalyst (0.10 mmol) was combined with 10 mL of H2O and 5 mL of EtOH and subjected to microwave irradiation at 900 W for 5 min. Upon completion of the reaction, the dry product was filtered and purified by multiple washes with aqueous ethanol. The products were obtained in high yield (Citation58) and the synthetic scheme is shown in .
5. Indole-containing compounds as a potent anti-cancer agent
Cancer is one of the most common causes of death globally (Citation59). WHO explains cancer as the uncontrolled proliferative growth and spreading of cells which can affect any part of the body (Citation60). Much research is being carried out to identify and develop potent anticancer agents (Citation61). Derivatives of different chemical moieties have been synthesized and their pharmacological activity has been explored by many researchers. One such chemical entity is Indole which has a wide range of pharmacological action. In this review, we compile the recent literature on indole-containing derivatives with potent activity as an anti-cancer agent.
Álvarez et al. reported compound 65 () which is cyano-substituted indolecombretastatin as a highly potent derivative against HT-29, A549, HL-60, and Hela cell lines with IC50 values of 0.00177, 0.0027, 0.000347, 0.00030 µM. The IC50 values of standard combretastatin A-4 were found 0.013 µM (Citation62). Cong et al. reported compound 66 which inhibited the A549 cell line with 2.5 nM (Citation63). In another study, compound 67 was found to be a potent inhibitor of HepG2 cells with IC50 values of 20 nM and was superior to paclitaxel PTX (IC50 = 2660 nM). This compound was also a potent inhibitor of the glioblastoma (U87MG) cell line with an IC50 value of 16 nM (Citation64). See et al. reported compound 68 (Citation65) and compound 69 (Citation66) as a potent inhibitor of paclitaxel-resistant colon cancer in a mouse model. Compounds 66 and 67 cause 74% and 76% tumor growth inhibition when given at a dose of 20 and 80 mg/kg twice a day. Ammar et al. reported compound 70 which has better activity on EGFR-Tks with IC50 = 23 nM than the standard drug lapatinib (27 nM). This compound showed maximum inhibition on the HepG2 cell line with an IC50 value of 7.53 µM (Citation67). Eldehna et al. reported compound 71 as the most potent of the series which exhibited potent activity on the MCF-7 cell line with IC50 = 1.04 µM (Citation68). Baldisserotto et al. reported compound 72 as the most potent of the series with IC50 values of 0.44 and 0.52 µM on the Colo38 and K562 cell lines (Citation69). Mphahlele et al. reported compound 73 which induced apoptosis in C3A and Caco-2 cells with LC50 values of 12.20 and 6.45 µM and exhibited significant inhibitory activity toward epidermal growth factor receptor (EGFR) with IC50 = 40.7 nM (standard gefitinib has IC50 = 38.9 nM) (Citation70). Li et al. synthesized indole-vinyl sulfone derivatives and reported compound 74 as the most potent inhibitor of cancer cell lines. This compound inhibited HePG2 with an IC50 value of 0.075, A549 with an IC50 value of 0.305 and K562 with an IC50 value of 0.055 µM (Citation71). Badria et al. synthesized chalcones derived from acetyl indole and among all the derivatives compound 75 showed maximum activity against triple-negative breast cancer cells (MDA-MB-231) with IC50 values of 13 µM (Citation72). Wang et al. reported compound 76 which significantly inhibits primary tumor growth, causes decreased tumor metastasis in melanoma xenograft models and also displayed significant capability to overcome paclitaxel resistance in a taxane-resistant PC-3/TxR model with an IC50 value of 66.9 nM (Citation73). Li et al. synthesized quinoline-indole derivatives and reported two compounds 77 and 78 as the most potent among the series with maximum inhibition on K562 Lymphoblast cells with IC50 value of 0.002 µM (Citation74). Naret et al. reported compound 79 which inhibited the growth of HCT 116 cancer cell lines with an IC50 = 2 nM. It showed maximum inhibition of human umbilical vein endothelial cells (HUVEC) with IC50 value of 0.81 nM as compared to standard drug combretastatin A-4 (IC50 = 1.7nM) (Citation75). Khelifi et al. reported compound 80 which was very much potent against HCT 116 cancer cell with IC50 = 0.070 nM than the standard drug isoCA-4 (IC50 = 1.80 nM) (Citation76). Cury et al. synthesized 2-carboxy indole derivatives and reported compound 81 as most potent among the series which inhibited leukemia cells CEM and RS4: 11 with EC50 value of 0.20 and 0.30 µM (Citation77). Diao et al. synthesized substituted 3-amino-1H-indole and 3- amino-1H-7-azaindole derivatives and reported compound 82 as most potent among the series which cause maximum inhibition of HeLa cancer cell line with IC50 value of 3.7 µM. Sigalapalli et al. synthesized thiazolidinone-indole derivatives and reported compound 83 as most potent among the series which inhibited HCT-15 cancer cell line with IC50 value of 0.92 ± 0.12 µM (Citation78). Bakherad et al. synthesized indole-thiosemicarbazone derivatives and reported compound 84 as the most potent among the series with IC50 values of 11.91 and 11.77 µM against Hep-G2 and A-549 as compared to standard etoposide and colchicine (Citation79). They also reported compound 85 as the most potent among the series which inhibited the A-549 cell line with IC50 = 12.5 μm (Citation80). El-Sharief et al. synthesized compound 86 as the most potent among the series which inhibited cancer cell line HEPG2 with an IC50 value of 3.63 µM (Citation81). Feng et al. synthesized indole derivatives and reported compound 87 as the most potent among the series which inhibited Hela, HepG2, A549, and MCF-7 cell lines with IC50 values of 13.71, 15.06, 9.42, and 14.77 μM (Citation82). Hu et al. synthesized Artemisinin-indole derivatives and reported compound 88 which showed the best antiproliferative activity with an IC50 value of 5.25 µM and showed significant MDR reversal which reversed adriamycin with IC50 = 0.76 μM against MCF-7/ADR cells (Citation83). Demurtas et al. synthesized indole hydrazone derivatives and reported compound 89 with best anticancer potential which inhibited the growth of Colo 38 and K562 with IC50 values of 0.59 and 0.067 µM (Citation84). Ozcan-Sezer et al. synthesized 2-methyl indole hydrazones and reported compound 90 as the most potent among the series which inhibited ER + human breast cancer (MCF-7 BUS) cells with an IC50 value of 8.67 µM (Citation85). Cheng et al. synthesized an indole derivative and reported compound 91 which showed highest anti-proliferative activities with GI50 value of 7.82 µM against THP-1 cell (Citation86). Petri et al. reported compound 92 which inhibited all the NCI-60 cell line pannel with GI50 values between 1.67 and 10.3 μM. This compound also inhibited human pancreatic cancer cell line (SUIT-2) with IC50 values of 8.40 µM (Citation87). Romagnoli et al. synthesized 2-alkoxycarbonyl-3- anilinoindoles and reported compound 93 as new antiproliferative agents that inhibited Hela, HT29, MCF-7, and HL-60 with IC50 value of 0.54, 0.32, 0.11 and 1.1 µM (Citation88). Li et al. reported compound 94 as most potent compound of the series which showed activity against HepG2 cells and SMMC-7721 cell line with IC50 values of 0.91 and 0.56 µM (Citation89). Zidar et al reported compound 95 as the most potent among the series which inhibited HeLa, A2780, and MSTO211H with GI50 values of 4.4, 2.2, and 2.4 µM (Citation90). Song et al. synthesized pyrazoline derivatives containing indole skeleton and reported compound 96 with the best anticancer activity having an IC50 value is 15.43 µM toward the MGC-803 cell line (Citation91). Catanzaro et al. reported compound 97 as the most potent anticancer compound among the series which inhibited CEM/C2 and Dld-1 with IC50 value of 9.16 and 7.13 µM (Citation92). Al-Warhi et al. developed a series of oxindole–indole derivatives and reported compound 98 as promising antitumor drugs toward breast cancer which inhibited MCF-7 cell line with IC50 value of 0.39 µM (Citation93). Bahuguna et al. reported compound 99 which showed good IC50 value of 3.423 µg mL−1 and 2.612 µg mL−1 against glioma cell lines (LN-18 and U87 cell) (Citation94). Swamy et al. synthesized azaindole-oxazole derivatives and reported compounds 100 as most potent among the series which has IC50 value of 0.034, 0.59, and 0.28 µM for MCF7, A549 and A375 cell line (Citation95). Al-Hussain et al synthesized indolyl-1,2,4-triazole hybrids and reported compound 101 which strongly inhibited the A498 cell line with double potency as compared to sunitinib. (IC50 of compound 9d = 0.64, sunitinib = 1.25 µM) (Citation96). Cascioferro et al. synthesized imidazo [2,1-b] [1,3,4]thiadiazole derivatives and reported compound 102 with cytotoxic activity against all NCI-60 cancer cell lines with IC50 ranging from 0.23 to 11.4 µM (Citation97). Al-Wabli et al synthesized Isatin–Indole Conjugates and reported compound 103 with seven times more anticancer potency as compared to sunitinib (IC50 of compound = 1.17 μM; sunitinib IC50 = 8.11 μM). Karadayi et al. reported indole-benzimidazoles and synthesized compound 104 with an IC50 value of 5.71 µM against the MCF-7 cell line. Compound 105 showed IC50 values of 32.2, 22.3 and 9.95 µM against MCF-7, MDA-MB-231, HEPG2 respectively (Citation98). Ren et al. reported derivative 106 which has average IC50 value of 50 nmol/L. This compound overcome paclitaxel resistance and showed nearly equal potency against both, a paclitaxel-resistant cancer cell line (A2780/T, IC50 = 9.7 nM) and the corresponding parental cell line (A2780S, IC50 = 6.2 nM) (Citation99). Wang et al reported compound 107 having most potent anticancer activity against A549 and MCF-7 with IC50 values of 3.75 and 1.77 µM (Citation100). Saruengkhanphasit et al. sunthesized 3-phenyl-1H-indole-2-carbohydrazide derivatives and reported compound 108 with the strongest activity against HepG2 cell line with IC50 value of 0.34 µM and compound 109 which has stronger activity against A549 cell line with IC50 value of 0.43 µM compared to doxorubicin (Citation101). Kode et al. synthesized phenstatin-based indole linked chalcone and reported compound 110 as most potent inhibitor of Oral squamous cell carcinoma cell line (SCC-29B) with GI50 value of 0.96 µM (Citation102). Yang et al. synthesized indole-based 1,2,4-triazole derivatives and reported compound 111 having superb antiproliferative efficacies against HeLa cell in the nanomolar range and no cytotoxic activity toward a normal human embryonic kidney cell line (HEK-293) (Citation103). Kaur et al. reported compound 112 as most potent Topo II inhibitor which inhibited cancer cell line MDA-MB-231, MCF7, A549 and HCT116 with IC50 values of 0.84, 0.58, 1.63 and 2.41 µM (Citation44). Konus et al. reported compound 113 as most potent anticancer agent with EC50 value of 86.98 µM for Caco-2, 38.725 µM for LnCap, 70.02 µM for HepG2, and 90.97 µM for Hek293 cell line (Citation104). Elkaeed et al. reported compound 114 which strongly inhibit MCF-7 and HCT 116 cell lines, with IC50 values of 12.93 and 11.52 µM (Citation105). Khalilullah et al. synthesized indole containing analogs and reported compound 115 as most potent among the series which inhibited all NCI-60 cell line. It showed 78.76% growth inhibition against leukemia cell line at 10 µM concentration. Hawash et al. synthesized Indole-acrylamide derivatives and reported compound 116 as most active against the Huh7 cancer cells with IC50 = 5.0 µM which was more potent than standard drug Sorafenib IC50 = 6.7 µM (Citation106). Veeranna et al. reported compound 117 as most potent 1,2,3-triazole tethered indole which inhibited MCF-7 and HepG-2 cell lines with IC50 values of 72.8 and 53.17 µM (Citation107). Puri et al. reported compound 118 as most potent indole derivative synthesized which inhibited MCT1 transport with IC50 value of 81 Nm. This compound inhibited cancer cell line A-549 and MCF-7 with GI50 values of 20.0 and 15.1 µM (Citation108). Yan et al. synthesized indole-chalcone derivatives and reported compound 119 as most potent derivatives the IC50 value of 6–35 nM toward six cancer cells (Citation109). Song et al reported compound 120 which was coumarin-indole derivatives which significantly inhibited gastric cancer cells MGC-803 and HGC-27 withIC50 value of 11 and 16 nM (Citation110). Perike et al.synthesized Indole-Thiazolidinedione-Triazole containing compounds and reported compound 121 which was effective against HePG-2, PC-3, HCT-116, and MCF7 cell lines and has IC50 value of 4.43, 8.03, 4.46, and 3.18 µM (Citation111). Gaur et al. synthesized Indole Based Arylsulfonylhydrazides and reported compound 122 as nontoxic against HEK 293 noncancerous cells. This compound inhibited MDA-MB-468 and MCF-7 cancer cells with the respective IC50 values of 8.2 and 13.2 μM (Citation112). Li et al. synthesized indole-guanidine derivatives and reported compound 123 as most potent in the series which inhibited cancer cell line (SMMC-7721) with IC50 value of 0.042 µM (Citation113). Trivedi et al. synthesized Indole–Schiff Base and reported compound 124 with highest anticancer activity against A549 cell line with IC50 values of 0.2 µM (Citation114). Yu et al. reported compound 125 with potent antiproliferative potential against MGC-803, MCF-7 and HCT-116 cells, with IC50 values of 9.47, 13.1 and 9.58 µM (Citation115). Han et al. synthesized Indole Derivatives of Panaxadiol and reported compound 126 with potent activity against A549 cells line with IC50 = 5.01 μM (Citation116). Zhao et al. synthesized indole-aryl amide derivatives and reported compound 127 which selectively inhibit HT29 cells with IC50 value of 2.61 µM without having any effect on healthy human intestinal cells (Citation117). Yao et al. reported compound 128 as most potent indole derivative among all synthesized compound which inhibited leukemia cell line especially K562 cells with IC50 value of 0.09 µM (Citation118). Budovska et al. synthesized bis-indole analogs and reported compound 129 as most potent derivative which significantly inhibited A549 cancer cell line with IC50 value of 8.7 µM without affecting normal cells (Citation119). Elganzory et al. reported compound 130 as most active against MCF-7 cell line with IC50 value of 0.5 µM (Citation120). Jia et al. reported compound 131 and 132 which inhibited tumor cell proliferation of SW480 human colon cancer cells with IC50 = 15.34 and 15.31 μM, respectively (Citation121). Zhang et al. reported compound 133 as the most potent derivative which inhibited A549 and K562 cells with IC50 values of 12.0 and 10 nM (Citation122). Almehdi et al. reported compound compound 134 as a Bcl-2 inhibitor which inhibited MDA-MB-231, MCF-7, and A549 with IC50 value of 5.22, 0.83 and 0.73 µM (Citation123). Ibrahim et al. reported compound 135 HePG-2 and HCT-116 and MCF-7 cell lines with IC50 value of 4.09, 4.42 and 6.19 µM (Citation124). Abdelazeem et al. synthesized 2- (thiophen-2-yl)-1H-indole derivatives and reported compound 136 as most potent inhibitor of HCT-116 and HT-29 cell lines with IC50 value of 3.566 and 24.39 µg/ml (Citation125).
Figure 48. Indole containing potent derivatives as anticancer agent reported in different literature.
6. Conclusion
Indole is an important heterocyclic ring system, a pharmacophore and a privileged scaffold in medicinal chemistry. Therefore, there is an unceasing search for an environmentally friendly method for its synthesis. The surge in interest for eco-friendly protocols aimed at decreasing chemical waste has propelled microwave irradiation (MW) as a preferred heating method for synthesizing organic compounds with promising pharmacological properties. MW accelerates chemical reactions by polar organic molecules absorbing microwaves, enabling reactions unfeasible through conventional heating. Its advantages include lower energy consumption, faster reaction rates, avoidance of toxic solvents, and easier regulation of reaction parameters. Despite the effectiveness of traditional acidic catalyst-based methods, the growing environmental awareness underscores the importance of exploring greener methodologies. These efforts prioritize eco-friendly methods to reduce toxic waste and energy usage. Conventional methods involve more chemicals and harsh conditions, while green chemistry-compliant synthesis employs milder conditions, less harmful chemicals, efficient catalysts, and solvent-free or water-mediated protocols for higher yields in shorter times. Therefore, based on this information, the use of MW for synthesizing compounds, both novel and existing, proves superior to conventional methods.
Consequently, this review highlights the development of greener protocols using MW over the years while showcasing the pharmacological allure of indoles. The review compiled and organized the Microwave irradiation technique as a green method recording their limitations, advantages, and substrate scope for synthesizing Indole derivatives. This review also focuses on the anticancer potential of indole moiety which will benefit the peer group to plan further exploration in this subject and improve current methodologies.
Author contributions
Alaa O. Baryyan contributed in the funding resources, validation, and manuscript improvement. Faisal A. Almalki: manuscript editing, conceptualization, article writing, funding, and supervision. All authors have read and agreed to the published version of the manuscript.
Supplemental Material
Download MS Word (23.1 KB)Disclosure statement
No potential conflict of interest was reported by the author(s).
References
- Van Order, R.B.; Lindwall, H.G. Chem. Rev. 1942, 30, 69–96.
- Gribble, G.W. J. Chem. Soc. Perkin Trans.. 2000, 1, 1045–1075. doi:10.1039/a909834h.
- Omar, F.; Tareq, A.M.; Alqahtani, A.M.; Dhama, K.; Sayeed, M.A.; Bin Emran, T.; Simal-Gandara, J. Molecules 2021, 26, 2–26.
- Kaushik, N.K.; Kaushik, N.; Attri, P.; Kumar, N.; Kim, C.H.; Verma, A.K.; Choi, E.H. Molecules 2013, 18, 6620–6662.
- Humphrey, G.R.; Kuethe, J.T. Chem. Rev. 2006, 106, 2875–2911.
- Bedlovičová, Z. Green Synthesis of Silver Nanomaterials; Elsevier, 2022; pp 547–569. doi:10.1016/B978-0-12-824508-8.00001-0.
- Soltys, L.; Olkhovyy, O.; Tatarchuk, T.; Naushad, M. Magnetochemistry 2021, 7, 2–34. doi:10.3390/magnetochemistry7110145.
- Anastas, P.; Eghbali, N. Chem. Soc. Rev. 2010, 39, 301–312.
- Zhang, P.; Wang, Q.; Fang, Y.; Chen, W.; Kirchon, A.A.; Baci, M.; Feng, M.; Sharma, V.K.; Zhou, H.-C. Metal-Organic Frameworks (MOFs) for Environmental Applications; Elsevier, 2019; pp 203–229. doi:10.1016/j.xcrp.2021.100348.
- Nasri, S.; Bayat, M.; Miankooshki, F.R.; Samet, N.H. Mol. Divers. 2022, 26, 3411–3445. doi:10.1007/s11030-021-10376-3.
- Tiwari, S.; Talreja, S. J. Pharm. Res. Int. 2022, 34, 74–79.
- Pawliszyn, J. Comprehensive Sampling and Sample Preparation: Analytical Techniques for Scientists; Academic Press: Amsterdam, 2012.
- Albuquerque, H.M.T.; Pinto, D.C.G.A.; Silva, A.M.S. Molecules 2021, 26, 1–15. doi:10.3390/molecules26206293.
- Kolagkis, P.X.; Galathri, E.M.; Kokotos, C.G. Beilstein J. Org. Chem. 2024, 20, 379–426.
- Yao, G.; Zhang, Z.-X.; Zhang, C.-B.; Xu, H.-H.; Tang, R.-Y. Molecules 2018, 23, 1–10. doi:10.3390/molecules23123317.
- Rode, N.D.; Abdalghani, I.; Arcadi, A.; Aschi, M.; Chiarini, M.; Marinelli, F. J. Org. Chem. 2018, 83, 6354–6362.
- Zhao, G.; Roudaut, C.; Gandon, V.; Alami, M.; Provot, O. Green Chem. 2019, 21, 4204–4210.
- Fujita, T.; Ide, K.; Jankins, T.C.; Nojima, T.; Ichikawa, J. Asian J. Org. Chem. 2019, 8, 637–640.
- Cirillo, P.F.; Caccavale, A.; DeLuna, A. J. Chem. Educ. 2020, 98, 567–571.
- Bellavita, R.; Casertano, M.; Grasso, N.; Gillick-Healy, M.; Kelly, B.G.; Adamo, M.F.A.; Menna, M.; Merlino, F.; Grieco, P. Symmetry (Basel) 2022, 14, 1–11. doi:10.3390/sym14030435.
- Mishra, S.K.; Mohanta, P.P.; Behera, A.K. Research Square. 2022. 1–13. doi:10.21203/rs.3.rs-1319618/v1.
- Rathod, A.S.; Biradar, J.S. Russ. J. Gen. Chem. 2018, 88, 2190–2196.
- Baharfar, R.; Azimi, R.; Asdollahpour, Z. Environ. Chem. Lett. 2018, 16, 677–682.
- Borad, M.A.; Bhoi, M.N.; Rathwa, S.K.; Vasava, M.S.; Patel, H.D.; Patel, C.N.; Pandya, H.A.; Pithawala, E.A.; Georrge, J.J. Interdiscip. Sci. Comput. Life Sci. 2018, 10, 411–418.
- Malkania, L.; Bedi, P.; Pramanik, T. Drug Invent. Today 2018, 10, 1740–1744.
- Bankar, S.R. Curr. Organocatalysis 2018, 5, 42–50.
- Denya, I.; Malan, S.F.; Enogieru, A.B.; Omoruyi, S.I.; Ekpo, O.E.; Kapp, E.; Zindo, F.T.; Joubert, J. Medchemcomm 2018, 9, 357–370.
- Minkovska, S.; Burdzhiev, N.; Alexiev, A.; Deligeorgiev, T. Chem. Pap. 2018, 72, 1549–1552.
- Shaikh, I.N.; Bagwan, U.F.; Hunagund, S.M.; Shaikh, I.N.; Afzal, M. Chem. Africa 2018, 1, 3–9.
- Singh, G.; Kalra, P.; Arora, A.; Sharma, G.; Singh, A.; Verma, V. Inorganica Chim. Acta 2018, 473, 186–193.
- Lee, Y.; Chiu, F.; Barve, I.J.; Sun, C. Adv. Synth. Catal. 2018, 360, 502–512.
- Patel, T.; Gaikwad, R.; Jain, K.; Ganesh, R.; Bobde, Y.; Ghosh, B.; Das, K.; Gayen, S. ChemistrySelect 2019, 4, 4478–4482.
- Bakkolla, M.G.; Taduri, A.K.; Bhoomireddy, R.D. Chem. Africa 2019, 2, 587–595.
- Wu, Z.; Wang, G.; Yuan, S.; Wu, D.; Liu, W.; Ma, B.; Bi, S.; Zhan, H.; Chen, X. Green Chem. 2019, 21, 3542–3546.
- Modi, M.; Jain, M. J. Heterocycl. Chem. 2019, 56, 3303–3312.
- Hote, B.S.; Siddiqui, T.A.J.; Pisal, P.M.; Mandawad, G.G. Polycycl. Aromat. Compd. 2022, 42, 1761–1769.
- Rathod, A.S.; Reddy, P.V.; Biradar, J.S. Russ. J. Org. Chem. 2020, 56, 662–670.
- Elkamhawy, A.; Paik, S.; Kim, H.J.; Park, J.-H.; Londhe, A.M.; Lee, K.; Pae, A.N.; Park, K.D.; Roh, E.J. J. Enzyme Inhib. Med. Chem. 2020, 35, 1568–1580.
- Duguta, G.; Muddam, B.; Kamatala, C.R.; Chityala, Y. Chem. Data Collect. 2020, 28, 1–11. doi:10.1016/j.cdc.2020.100382.
- Gohain, S.B.; Thakur, A.J. ChemistrySelect 2021, 6, 6773–6780.
- Taheri, M.; Mohebat, R.; Moslemin, M.H. Polycycl. Aromat. Compd. 2023, 43, 586–596.
- Gampa, M.; Padmaja, P.; Aravind, S.; Reddy, P.N. Chem. Heterocycl. Compd. 2021, 57, 1176–1180.
- R. A. Luna-Mora; C. Álvarez-Toledano; H. Rios-Guerra; F. Ortega-Jiménez; J. G. López-Cortés; J. Pérez-Flores; Á. Torres-Reyes; L. Moreno-González; A. M. Zaldívar; F. Barrera-Téllez, ARKIVOC, 2021, 242–252.
- Kaur, M.; Mehta, V.; Wani, A.A.; Arora, S.; Bharatam, P.V.; Sharon, A.; Singh, S.; Kumar, R. Bioorg. Chem. 2021, 114, 1–14. doi:10.1016/j.bioorg.2021.105114.
- Nirwan, N.; Pareek, C. Curr. Chem. Lett. 2021, 10, 261–270.
- Hassan, E.A.; Mostafa, H.M.; Zayed, S.E. Phosphorus. Sulfur. Silicon Relat. Elem. 2020, 196, 476–485.
- Singh, A.; Singh, S.; Sewariya, S.; Singh, N.; Singh, P.; Kumar, A.; Bandichhor, R.; Chandra, R. Tetrahedron 2021, 84, 1–9. doi:10.1016/j.tet.2021.132017.
- Mokariya, J.A.; Kalola, A.G.; Prasad, P.; Patel, M.P. Mol. Divers. 2022, 26, 936–979. doi:10.1007/s11030-021-10212-8.
- Rajesab, P.; Chavan, P.W.; Badiger, J.G.; Hanamshetty, P.C. Asian J Pharm Clin Res 2022, 15, 62–66.
- Wang, J.; Zhu, S.; Liu, Y.; Zhu, X.; Shi, K.; Li, X.; Zhu, S. Synth. Commun. 2022, 52, 85–95.
- Avula, R.; Babu, H.S.; Venkatanarayana, M. Chem. Data Collect. 2022, 38, 100841. doi:10.1016/j.cdc.2022.100841.
- Rivas-Loaiza, J.A.; García-Merinos, J.P.; Ramírez-Díaz, M.I.; López-Ruiz, H.; López, Y. J. Mol. Struct. 2022, 1264, 133209. doi:10.1016/j.molstruc.2022.133209.
- Al-Ajely, H.M. MINAR International Journal of Applied Sciences and Technology. 2022, 4, 514–519.
- Katowah, D.F.; Hassaneen, H.M.E.; Farghaly, T.A. Arab. J. Chem. 2022, 15, 1–13. doi:10.1016/j.arabjc.2022.103930.
- Vijayasaradhi, S.; Muralikrishna, V.; Srinivas, R.; Rao, C.P.K.; Prasad, K.Y.; Prasad, B.; Khanna, B. Results Chem. 2023, 5, 1–6. doi:10.1016/j.rechem.2022.100754.
- Sahu Priyanka, N.K.; Mahajan, A.T.; Sharma, V.; Suhas, K.P.; Tripathi, P.; Mathur, M.; Jain, M.; Chaudhary, S. J. Mol. Struct. 2024, 1303, 137445. doi:10.1016/j.molstruc.2023.137445.
- Chudasama, D.D.; Rajput, C.V.; Patel, M.S.; Parekh, J.N.; Patel, H.C.; Chikhaliya, N.P.; Puerta, A.; Padrón, J.M.; Ram, K.R. Arch. Pharm. (Weinheim) 2024, 357, 2300632–2300649.
- Abd El-Lateef, H.M.; Kamel, M.S.; Alzahrani, A.Y.A.; Khalaf, M.M.; Gouda, M.; Ali, M.A.E.A.A. Bull. Chem. Soc. Ethiop. 2024, 38, 1077–1090.
- Tasneem, S.; Alam, M.M.; Parvez, S.; Khan, P.F.; Garg, M.; Amir, M.; Akhter, M.; Amin, S.; Khan, M.A. Future Med. Chem. 2023, 15, 1669–1685.
- Shaquiquzzaman, M.; Verma, G.; Marella, A.; Akhter, M.; Akhtar, W.; Khan, M.F.; Tasneem, S.; Alam, M.M. Eur. J. Med. Chem. 2015, 102, 487–529.
- Azad, I.; Nasibullah, M.; Khan, T.; Hassan, F.; Akhter, Y. J. Mol. Graph. Model. 2018, 81, 211–228.
- Álvarez, R.; Gajate, C.; Puebla, P.; Mollinedo, F.; Medarde, M.; Peláez, R. Eur. J. Med. Chem. 2018, 158, 167–183.
- Cong, H.; Zhao, X.; Castle, B.T.; Pomeroy, E.J.; Zhou, B.; Lee, J.; Wang, Y.; Bian, T.; Miao, Z.; Zhang, W. Mol. Pharm. 2018, 15, 3892–3900.
- La Regina, G.; Bai, R.; Coluccia, A.; Naccarato, V.; Famiglini, V.; Nalli, M.; Masci, D.; Verrico, A.; Rovella, P.; Mazzoccoli, C. Eur. J. Med. Chem. 2018, 152, 283–297.
- See, C.S.; Kitagawa, M.; Liao, P.-J.; Lee, K.H.; Wong, J.; Lee, S.H.; Dymock, B.W. Eur. J. Med. Chem. 2018, 156, 344–367.
- See, C.S.; Kitagawa, M.; Liao, P.-J.; Lee, K.H.; Wong, J.; Lee, S.H.; Dymock, B.W. Bioorg. Med. Chem. Lett. 2019, 29, 216–219.
- Ammar, Y.A.; El-Sharief, A.M.S.; Belal, A.; Abbas, S.Y.; Mohamed, Y.A.; Mehany, A.B.M.; Ragab, A. Eur. J. Med. Chem. 2018, 156, 918–932.
- Eldehna, W.M.; Abo-Ashour, M.F.; Ibrahim, H.S.; Al-Ansary, G.H.; Ghabbour, H.A.; Elaasser, M.M.; Ahmed, H.Y.A.; Safwat, N.A. J. Enzyme Inhib. Med. Chem. 2018, 33, 686–700.
- Baldisserotto, A.; Demurtas, M.; Lampronti, I.; Moi, D.; Balboni, G.; Vertuani, S.; Manfredini, S.; Onnis, V. Eur. J. Med. Chem. 2018, 156, 118–125.
- Mphahlele, M.J.; Mmonwa, M.M.; Aro, A.; McGaw, L.J.; Choong, Y.S. Int. J. Mol. Sci. 2018, 19, 1–17. doi:10.3390/ijms19082232.
- Li, W.; Sun, H.; Xu, F.; Shuai, W.; Liu, J.; Xu, S.; Yao, H.; Ma, C.; Zhu, Z.; Xu, J. Bioorg. Chem. 2019, 85, 49–59.
- Badria, F.A.; Soliman, S.M.; Atef, S.; Islam, M.S.; Al-Majid, A.M.; Dege, N.; Ghabbour, H.A.; Ali, M.; El-Senduny, F.F.; Barakat, A. Molecules 2019, 24, 1–12. doi:10.3390/molecules24203728.
- Wang, Q.; Arnst, K.E.; Wang, Y.; Kumar, G.; Ma, D.; White, S.W.; Miller, D.D.; Li, W.; Li, W. J. Med. Chem. 2019, 62, 6734–6750.
- Li, W.; Shuai, W.; Sun, H.; Xu, F.; Bi, Y.; Xu, J.; Ma, C.; Yao, H.; Zhu, Z.; Xu, S. Eur. J. Med. Chem. 2019, 163, 428–442.
- Naret, T.; Khelifi, I.; Provot, O.; Bignon, J.; Levaique, H.; Dubois, J.; Souce, M.; Kasselouri, A.; Deroussent, A.; Paci, A. J. Med. Chem. 2018, 62, 1902–1916.
- Khelifi, I.; Naret, T.; Hamze, A.; Bignon, J.; Levaique, H.; Alvarez, M.C.G.; Dubois, J.; Provot, O.; Alami, M. Eur. J. Med. Chem. 2019, 168, 176–188.
- Cury, N.M.; Capitao, R.M.; do C, R.; de Almeida, B.; Artico, L.L.; Correa, J.R.; Dos Santos, E.F.S.; Yunes, J.A.; Correia, C.R.D. Eur. J. Med. Chem. 2019, 181, 111570. doi:10.1016/j.ejmech.2019.111570.
- Sigalapalli, D.K.; Pooladanda, V.; Singh, P.; Kadagathur, M.; Guggilapu, S.D.; Uppu, J.L.; Tangellamudi, N.D.; Gangireddy, P.K.; Godugu, C.; Bathini, N.B. Bioorg. Chem. 2019, 92, 103188. doi:10.1016/j.bioorg.2019.103188.
- Bakherad, Z.; Safavi, M.; Fassihi, A.; Sadeghi-Aliabadi, H.; Bakherad, M.; Rastegar, H.; Saeedi, M.; Ghasemi, J.B.; Saghaie, L.; Mahdavi, M. Chem. Biodivers. 2019, 16, 5261–5290. doi:10.1002/cbdv.201800470.
- Bakherad, Z.; Safavi, M.; Fassihi, A.; Sadeghi-Aliabadi, H.; Bakherad, M.; Rastegar, H.; Ghasemi, J.B.; Sepehri, S.; Saghaie, L.; Mahdavi, M. Res. Chem. Intermed. 2019, 45, 2827–2854.
- El-Sharief, A.M.S.; Ammar, Y.A.; Belal, A.; El-Sharief, M.A.M.S.; Mohamed, Y.A.; Mehany, A.B.M.; Ali, G.A.M.E.; Ragab, A. Bioorg. Chem. 2019, 85, 399–412.
- Feng, Y.; Teng, X.; Gu, J.; Yu, B.; Luo, Y.; Ye, L. Med. Chem. Res. 2019, 28, 133–142.
- Hu, Y.; Li, N.; Zhang, J.; Wang, Y.; Chen, L.; Sun, J. Bioorg. Med. Chem. Lett. 2019, 29, 1138–1142.
- Demurtas, M.; Baldisserotto, A.; Lampronti, I.; Moi, D.; Balboni, G.; Pacifico, S.; Vertuani, S.; Manfredini, S.; Onnis, V. Bioorg. Chem. 2019, 85, 568–576.
- Ozcan-Sezer, S.; Ince, E.; Akdemir, A.; Ceylan, ÖÖ; Suzen, S.; Gurer-Orhan, H. Xenobiotica 2019, 49, 549–556.
- Cheng, G.; Wang, Z.; Yang, J.; Bao, Y.; Xu, Q.; Zhao, L.; Liu, D. Bioorg. Chem. 2019, 84, 410–417.
- Petri, G.L.; Cascioferro, S.; El Hassouni, B.; Carbone, D.; Parrino, B.; Cirrincione, G.; Peters, G.J.; Diana, P.; Giovannetti, E. Anticancer Res. 2019, 39, 3615–3620.
- Romagnoli, R.; Prencipe, F.; Oliva, P.; Salvador, M.K.; Brancale, A.; Ferla, S.; Hamel, E.; Viola, G.; Bortolozzi, R.; Persoons, L. Bioorg. Chem. 2020, 97, 103665. doi:10.1016/j.bioorg.2020.103665.
- Li, A.-L.; Hao, Y.; Wang, W.-Y.; Liu, Q.-S.; Sun, Y.; Gu, W. Int. J. Mol. Sci. 2020, 21, 1–20. doi:10.3390/ijms21082876.
- Zidar, N.; Secci, D.; Tomašič, T.; Mašič, L.P.; Kikelj, D.; Passarella, D.; Argaez, A.N.G.; Hyeraci, M.; Dalla Via, L. ACS Med. Chem. Lett. 2020, 11, 691–697.
- Song, Y.; Feng, S.; Feng, J.; Dong, J.; Yang, K.; Liu, Z.; Qiao, X. Eur. J. Med. Chem. 2020, 200, 112459. doi:10.1016/j.ejmech.2020.112459.
- Catanzaro, E.; Betari, N.; Arencibia, J.M.; Montanari, S.; Sissi, C.; De Simone, A.; Vassura, I.; Santini, A.; Andrisano, V.; Tumiatti, V. Eur. J. Med. Chem. 2020, 202, 112504. doi:10.1016/j.ejmech.2020.112504.
- Al-Warhi, T.; El Kerdawy, A.M.; Aljaeed, N.; Ismael, O.E.; Ayyad, R.R.; Eldehna, W.M.; Abdel-Aziz, H.A.; Al-Ansary, G.H. Molecules 2020, 25, 1–19. doi:10.3390/molecules25092031.
- Bahuguna, A.; Singh, A.; Kumar, P.; Dhasmana, D.; Krishnan, V.; Garg, N. Comput. Biol. Med. 2020, 116, 103574. doi:10.1016/j.compbiomed.2019.103574.
- Venkat Swamy, P.; Kiran Kumar, V.; Radhakrishnam Raju, R.; Venkata Reddy, R.; Chatterjee, A.; Kiran, G.; Sridhar, G. Synth. Commun. 2020, 50, 71–84.
- Al-Hussain, S.A.; Farghaly, T.A.; Zaki, M.E.A.; Abdulwahab, H.G.; Al-Qurashi, N.T.; Muhammad, Z.A. Bioorg. Chem. 2020, 105, 104330. doi:10.1016/j.bioorg.2020.104330.
- Cascioferro, S.; Petri, G.L.; Parrino, B.; Carbone, D.; Funel, N.; Bergonzini, C.; Mantini, G.; Dekker, H.; Geerke, D.; Peters, G.J. Eur. J. Med. Chem. 2020, 189, 112088. doi:10.1016/j.ejmech.2020.112088.
- Karadayi, F.Z.; Yaman, M.; Kisla, M.M.; Keskus, A.G.; Konu, O.; Ates-Alagoz, Z. Bioorg. Chem. 2020, 100, 103929. doi:10.1016/j.bioorg.2020.103929.
- Ren, Y.; Wang, Y.; Li, G.; Zhang, Z.; Ma, L.; Cheng, B.; Chen, J. J. Med. Chem. 2021, 64, 4498–4515.
- Wang, G.; He, M.; Liu, W.; Fan, M.; Li, Y.; Peng, Z. Arab. J. Chem. 2022, 15, 1–11. doi:10.1016/j.arabjc.2021.103504.
- Saruengkhanphasit, R.; Butkinaree, C.; Ornnork, N.; Lirdprapamongkol, K.; Niwetmarin, W.; Svasti, J.; Ruchirawat, S.; Eurtivong, C. Bioorg. Chem. 2021, 110, 104795. doi:10.1016/j.bioorg.2021.104795.
- Kode, J.; Kovvuri, J.; Nagaraju, B.; Jadhav, S.; Barkume, M.; Sen, S.; Kasinathan, N.K.; Chaudhari, P.; Mohanty, B.S.; Gour, J. Bioorg. Chem. 2020, 105, 104447. doi:10.1016/j.bioorg.2020.104447.
- Yang, F.; Jian, X.-E.; Chen, L.; Ma, Y.-F.; Liu, Y.-X.; You, W.-W.; Zhao, P.-L. New J. Chem. 2021, 45, 21869–21880.
- Konus, M.; Çetin, D.; Kızılkan, N.D.; Yılmaz, C.; Fidan, C.; Algso, M.; Kavak, E.; Kivrak, A.; Kurt-Kızıldoğan, A.; Otur, Ç J. Mol. Struct. 2022, 1263, 133168. doi:10.1016/j.molstruc.2022.133168.
- Elkaeed, E.B.; Yousef, R.G.; Elkady, H.; Gobaara, I.M.M.; Alsfouk, A.A.; Husein, D.Z.; Ibrahim, I.M.; Metwaly, A.M.; Eissa, I.H. Processes 2022, 10, 1–25. doi:10.3390/pr10071391.
- Hawash, M.; Kahraman, D.C.; Olgac, A.; Ergun, S.G.; Hamel, E.; Cetin-Atalay, R.; Baytas, S.N. J. Mol. Struct. 2022, 1254, 132345. doi:10.1038/s41598-022-23050-x.
- Veeranna, D.; Ramdas, L.; Ravi, G.; Bujji, S.; Thumma, V.; Ramchander, J. ChemistrySelect 2022, 7, e202201758. doi:10.1002/slct.202201758.
- Puri, S.; Stefan, K.; Khan, S.L.; Pahnke, J.; Stefan, S.M.; Juvale, K. J. Med. Chem. 2022, 66, 657–676.
- Yan, J.; Xu, Y.; Jin, X.; Zhang, Q.; Ouyang, F.; Han, L.; Zhan, M.; Li, X.; Liang, B.; Huang, X. Eur. J. Med. Chem. 2022, 227, 113897. doi:10.1016/j.ejmech.2021.113897.
- Song, J.; Guan, Y.-F.; Liu, W.-B.; Song, C.-H.; Tian, X.-Y.; Zhu, T.; Fu, X.-J.; Qi, Y.-Q.; Zhang, S.-Y. Eur. J. Med. Chem. 2022, 238, 114467. doi:10.1016/j.ejmech.2022.114467.
- Perike, N.; Edigi, P.K.; Nirmala, G.; Thumma, V.; Bujji, S.; Naikal, P.S. ChemistrySelect 2022, 7, e202203778. doi:10.1002/slct.202203778.
- Gaur, A.; Peerzada, M.N.; Khan, N.S.; Ali, I.; Azam, A. ACS Omega 2022, 7, 42036–42043.
- Li, J.; Si, R.; Zhang, Q.; Li, Y.; Zhang, J.; Shan, Y. Chem. Biol. Interact. 2022, 368, 110242. doi:10.1016/j.cbi.2022.110248.
- Trivedi, K.M.; Patel, U.P.; Dabhi, R.C.; Maru, J.J. Russ. J. Bioorganic Chem. 2022, 48, 601–608.
- Yu, G.-X.; Hu, Y.; Zhang, W.-X.; Tian, X.-Y.; Zhang, S.-Y.; Zhang, Y.; Yuan, S.; Song, J. Molecules 2022, 27, 1–15. doi:10.3390/molecules27154996.
- Han, L.; Li, T.; Miao, D.; Lee, J.; Xiao, S.; Piao, H.; Zhao, Y. Chem. Biodivers. 2022, 19, e202200372. doi:10.1002/cbdv.202200372.
- Zhao, J.; Carbone, J.; Farruggia, G.; Janecka, A.; Gentilucci, L.; Calonghi, N. Molecules 2022, 28, 1–15. doi:10.3390/molecules28010265.
- Yao, Y.; Huang, T.; Wang, Y.; Wang, L.; Feng, S.; Cheng, W.; Yang, L.; Duan, Y. J. Enzyme Inhib. Med. Chem. 2022, 37, 652–665.
- Budovska, M.; Michalkova, R.; Kello, M.; Vaskova, J.; Mojzis, J. Molecules 2023, 28, 1–19. doi:10.3390/molecules28010251.
- Elganzory, H.H.; Alminderej, F.M.; El-Bayaa, M.N.; Awad, H.M.; Nossier, E.S.; El-Sayed, W.A. Molecules 2022, 27, 1–23. doi:10.3390/molecules27206960.
- Jia, H.-W.; Yang, H.-L.; Xiong, Z.-L.; Deng, M.-H.; Wang, T.; Liu, Y.; Cheng, M. Bioorg. Chem. 2022, 129, 106213. doi:10.1016/j.bioorg.2022.106213.
- Zhang, G.; Tang, Z.; Fan, S.; Li, C.; Li, Y.; Liu, W.; Long, X.; Zhang, W.; Zhang, Y.; Li, Z. J. Enzyme Inhib. Med. Chem. 2023, 38, 1–15. doi:10.1080/14756366.2022.2163393.
- Almehdi, A.M.; Soliman, S.S.M.; El-Shorbagi, A.-N.A.; Westwell, A.D.; Hamdy, R. Int. J. Mol. Sci. 2023, 24, 1–19. doi:10.3390/ijms241914656.
- Ibrahim, M.S.; Farag, B.; Al-Humaidi, J.Y.; Zaki, M.E.A.; Fathalla, M.; Gomha, S.M. Molecules 2023, 28, 1–15. doi:10.3390/molecules28093869.
- N. Abdelazeem; S. A Gouharb; C. A Fahmy; Z. A Elshahid; M. El-Hussieny. Cell Cycle Arrest Downregulation MiR-25, C-Myc Il-6.