ABSTRACT
As tourism increases globally, studies have documented impacts on wildlife from anthropogenic disturbances. In this observational experiment we aimed to investigate if snowmobile traffic affected the diurnal activity of Arctic fox in High Arctic Svalbard. We conducted the study in two areas in Svalbard, one control area with low snowmobile traffic and one experimental area with high snowmobile traffic. In each area 10 camera-traps, baited with reindeer carcasses, were positioned and programmed to take photographs every five minutes. The proportion of photographs with foxes was higher during the night than during the day, and the difference between night and day was larger in the area with more snowmobile traffic. By using data obtained according to a similar study design in two Arctic Russian sites, Yamal and Nenetsky, with little human activity and low snowmobile traffic, we were able to compare Arctic fox activity patterns in Svalbard on a larger scale. Our results indicate that snowmobile traffic had an impact on the diurnal activity of the Arctic fox in Svalbard, while there were no obvious diurnal activity patterns among Russian foxes. Even the area with low snowmobile traffic in Svalbard showed increased use of the reindeer carcasses during the night compared to one of the Russian sites, where foxes used carcasses equally during day and night. Such knowledge is of importance in designing cautious management practices.
ABBREVIATIONS:
Travel and the tourism industry are steadily increasing on a global scale (Reid et al. Citation2005), bringing human traffic into areas where wildlife was previously undisturbed. A large body of literature examining the effects of anthropogenic disturbances on wildlife includes reports of behavioural and physiological studies of ungulates (Eckstein et al. Citation1979; MacArthur et al. Citation1979; Freddy et al. Citation1986; Creel et al. Citation2002), marine mammals (Born et al. Citation1999), seabirds (Gabrielsen & Smith 1995) and carnivores (Amstrup Citation1993; Creel et al. Citation2002; Andersen & Aars Citation2008; Gese et al. Citation2013), among other animals. Such disturbances may lead to an array of impacts, with different effects on individuals, populations or species interactions, e.g., disturbances may displace predators but not prey (Muhly et al. Citation2011).
Easier year-round access to many parts of the Arctic has facilitated increased tourism and recreation in the region (CAFF Citation2013). One activity that has increased in the Arctic is snowmobiling. Effects of snowmobile disturbances on wildlife can be species-specific, vary across ecosystems (e.g., predator abundances and hunting history are different across the Arctic) or be related to snow properties (e.g., how easily animals can walk without breaking through the snow) (Neumann & Merriam Citation1972; Creel et al. Citation2002; Andersen & Aars Citation2008; Gese et al. Citation2013; Salek et al. Citation2014).
The Norwegian High-Arctic archipelago of Svalbard has experienced a fivefold increase in visitors from the early 1990s (23 000 visitors) to 2014 (120 000 visitors; Governor of Svalbard Citation2014). Guided snowmobile tours are the most popular wintertime activity for tourists (Governor of Svalbard Citation2014). Since Svalbard has high standards for environmental management, the need for research on the effect of motorized vehicles on wildlife has been highlighted in a White Paper to the Norwegian parliament (Ministry of Justice and the Police Citation2010). Svalbard’s simple terrestrial ecosystem comprises few resident species, including one ungulate (Svalbard reindeer [Rangifer tarandus platyrhynchus]), one ptarmigan species (Svalbard rock ptarmigan [Lagopus hyperborea]) and two mammalian predator species: the Arctic fox (Vulpes lagopus) and the polar bear (Ursus maritimus) (Ims et al. Citation2014). Negative responses to snowmobile traffic have been documented for both Svalbard reindeer and polar bears (Tyler Citation1991; Andersen & Aars Citation2008). However, for reindeer the evidence is equivocal. Based on the level of snowmobile traffic in 1987, no major negative effect at the population level was detected in reindeer (Tyler Citation1991). However, in a recent study, individual reindeer showed clear avoidance of snowmobile trails (Tangberg Citation2016). A study of polar bears from 2003 to 2005 found that females with small cubs, which reacted at the longest distances from a snowmobile, were especially disturbed, but it was not possible based on individual behaviour to draw conclusions about long-term responses at the population level (Andersen & Aars Citation2008). No published study has yet focused on effects of snowmobile traffic on the Arctic fox. However, one study in Svalbard found differences in reaction patterns to snowmobile traffic (Eid et al. unpubl. data). The differences in reaction patterns differed depending on whether the fox was located in flat or in steep terrain. Foxes in flat terrain had a shorter flight distance to the snowmobile (200 m) compared to foxes in steep terrain (500 m; Eid et al. unpubl. data).
During the International Polar Year Arctic Predators project (2007–2010) camera-traps were used to study the scavenger guilds at several sites from the Russian Arctic (Yamalo-Nenetsky Autonomous Okrug and Nenetsky Autonomous Okrug) to the Norwegian Arctic (Varanger and Svalbard). A surprising result emerged that led to the present study (Rodnikova et al. Citation2010; Ehrich et al. Citation2011; Gauthier & Berteaux Citation2011). Although Arctic foxes at the Norwegian sites visited baited camera-traps in March–April for the same amount of time during a 24-hour period as did Arctic foxes at the Russian sites, their visits were at different times of the day: in Varanger and Svalbard, Arctic foxes were recorded at camera-traps mainly during the night whereas at the Russian sites they did not appear more often at night than during the day (Rodnikova et al. Citation2010). Neither the light regime nor the composition of the predator guild could explain the differences in fox activity pattern between Svalbard and Yamal/Nenetsky (Rodnikova et al. Citation2010; Ehrich et al. Citation2011). The fact that Arctic foxes in Varanger used the camera-traps mainly during the night was explained by the presence of scavengers, such as golden eagle (Aquila chrysaetos), competing and excluding the Arctic fox from visiting the camera-traps during the light part of the day (Killengreen et al. Citation2012). Since Arctic foxes in Svalbard and at the Russian sites lack competitors and predators (Fuglei et al. Citation2003; Eide et al. Citation2012; Pokrosvky et al. Citation2014; Sokolov et al. Citation2016), and live under similar light conditions in late winter, we expected no differences in diurnal activity documented by camera-traps in Svalbard and at the Russian sites.
Earlier studies have suggested that human activity can alter carnivore behaviour and spatial distribution (Amstrup Citation1993; Creel et al. Citation2002; George & Crooks Citation2006; Andersen & Aars Citation2008; Gese et al. Citation2013). One plausible reason for the differences in time-use between foxes in Svalbard and Russia that we intended to test in our follow-up study may relate to the different levels of snowmobile traffic between Svalbard (high traffic) and Russia (low traffic). Because all the camera-traps in the Arctic Predators study were located along the main snowmobile trail from Longyearbyen, the largest settlement in Svalbard (Governor of Svalbard Citation2014), we hypothesized that in our follow-up study Arctic foxes in Svalbard would visit the camera-traps mainly during the night to avoid motorized traffic. In this study we used baited camera-traps to address three specific aims: (1) to investigate if snowmobile traffic changed the diurnal activity of the Arctic fox by comparing one control area with low snowmobile traffic to an experimental area with high snowmobile traffic in Svalbard in 2013; (2) to investigate if there are year-specific differences in Arctic fox activity in Svalbard by comparing the data from the experimental area (high snowmobile traffic) in 2013 with similar data from the same area in 2008 and 2009 (Rodnikova et al. Citation2010); (3) to investigate if there are differences in diurnal activity at a larger scale between different Arctic sites by comparing Arctic fox activity in the control area in Svalbard (low snowmobile traffic) with Arctic fox populations in the Russian Arctic (low snowmobile traffic).
Methods
Study site and design
During 2008 and 2009, we studied the diurnal activity of Arctic fox at three Arctic sites that had been part of the Arctic Predators project (Ehrich et al. Citation2011). One site was in Nordenskiöld Land, Spitsbergen, Svalbard (78°N, 16°E; ). The two other sites were in Russia, in Erkuta on the southern Yamal Peninsula (hereafter Yamal), at approximately 68°N, 69°E, and Nenetsky Rige in the Pechora lowlands (hereafter Nenetsky), at approximately 68°N, 53°E (). For descriptions of the two Russian study areas, see, among others, Sokolov et al. (Citation2016) and Pokrosvky et al. (Citation2014), respectively. The observations spanned a large latitudinal range, from the High-Arctic Nordenskiöld Land to the Low-Arctic Russian sites (CAFF Citation2013).
Figure 1. (a) Map showing the study area in NordenskiöldLand, Svalbard, with camera-traps in the experimental area (black squares) and control area (black dots). The most used snowmobile trails are indicated by blue lines. (b) Map showing the study sites in Svalbard (Nordenskiöld Land) and in Russia (Nenetsky and Yamal).
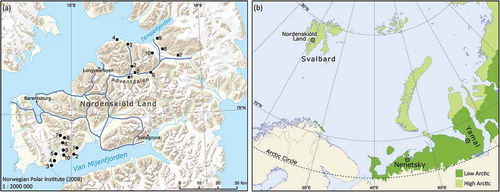
In 2013, we further investigated the diurnal activity of Arctic fox with an observational experiment in Svalbard. To test for the hypothesized effect of disturbance from snowmobile traffic, we established a control area (77°N, 14°E; 130 km2) in addition to the experimental area (480 km2) already established in Nordenskiöld Land in 2008. The new control site, close to Van Mijenfjorden on Nordenskiöld Land and approximately 65 km from the experimental area, was located in an area that is infrequently visited by people and is characterized by low snowmobile traffic (). The experimental site was located in an area with more frequent human visits and is characterized by high snowmobile traffic (). The experimental area includes the large Adventdalen valley, the main route for most snowmobiles leaving Longyearbyen. After leaving Adventdalen there are several snowmobile trails to choose from, but the majority of organized snowmobile tours for tourists go to Tempelfjorden, Barentsburg, Sveagruva and the east coast of Spitsbergen. The camera-trap located closest to Longyearbyen captured part of the snowmobile traffic out of town, showing both a weekly pattern with an increase in traffic each weekend as well as increasing traffic during the study period ().
Figure 2. Number of snowmobiles observed every 5 minutes at one camera-trap positioned right outside the main settlement, Longyearbyen, Svalbard, in the experimental area from 1 March to 29 April 2013.
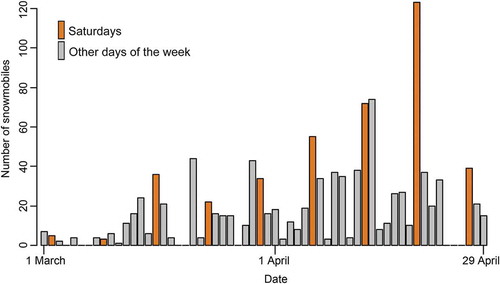
To estimate the diurnal activity of Arctic foxes we established 10 automatic camera-trap stations with bait at each site in Svalbard and in Russia. Automatic camera-trap stations with or without bait are a well-known and increasingly used method for studying effects of human activities on wildlife (Erb et al. Citation2012; Hamel et al. Citation2013; Barrueto et al. Citation2014). At each camera-trap, one automatic digital camera was operational from approximately the beginning of March to the beginning of May. During 2008 and 2009, each automatic camera-trap was equipped with a white flash that was activated at night, while in 2013 each camera-trap was equipped with an infrared flash. In the experimental area, all camera-traps were identical from year to year, except for one camera-trap that was moved further away from a cabin in 2013. Distance between adjacent camera-traps varied from 3 to 6 km. Locally, the exact positions of the camera-traps were chosen based on topography, mainly to avoid snow accumulation on cameras and baits. We programmed the automatic camera-traps to take time-lapse photographs of the immediate area around a carcass at 10-minute intervals in 2008 and 2009 and at 5-minute intervals in 2013. In Svalbard, the bait used to attract Arctic foxes consisted of 10–20 kg pieces of Svalbard reindeer that had died of natural causes, and in Russia the baits were remains of slaughtered semi-domesticated reindeer. We changed digital flash cards approximately every two weeks and renewed the carcasses that had been completely or partly consumed at biweekly inspections.
Data and statistical analyses
To estimate how the diurnal activity pattern of Arctic fox responded to snowmobile traffic we divided each 24-hour period into two 12-hour intervals, defining day as 07:00–19:00 and night as 19:00–07:00, reflecting the general pattern of snowmobile activity. For all statistical analyses, the response variable was the proportion of photographs with Arctic fox taken at each camera-trap per 12-hour period. We removed from the data set photographs that were obscured because of weather conditions or time periods during which the carcass was missing. We used GLMM with a logit link and a binomial error distribution to analyse the proportion of photographs with Arctic foxes. Analyses were carried out using the function lmer of the package lme4 (Bates et al. Citation2015) in R version 3.2.2 software (R Development Core Team Citation2015). We compared models with increasing complexity using likelihood ratio tests, and checked the selected models graphically for trends in the raw residuals, presence of outliers and approximate normality of the random effects.
First, we evaluated the effect of high and low snowmobile traffic at a local scale on Arctic fox diurnal activity pattern. We included as factorial fixed effect explanatory variables the time period (Period: day or night), and the two areas on Nordenskiöld Land (Treatment: experimental [high snowmobile activity] or control [low snowmobile activity]). In addition, since the use of carcasses may change over the course of the winter season, we included ‘day-of-the-year’ as a continuous variable to account for seasonal changes (Date). Both the location of the camera-traps and the specific local daily circumstances such as weather conditions may influence the proportion of photographs with Arctic foxes. We therefore included two nested random factors for the intercept: the identity of each camera-trap and the identity of each day at each camera-trap as factors nested within camera-traps (camera-day-ID). A set of five candidate models was assembled including an increasing number of additive fixed effects (Date, Period, Treatment) and the interactions Treatment × Period and Treatment × Date. In addition to the graphical tests described above, we tested for temporal autocorrelation by plotting temporal autocorrelation plots of the residuals for each camera-trap separately. This test was only done for the analysis of the data from 2013, which was the year with most data.
Second, we evaluated whether the effect of snowmobile traffic at the local scale persisted over several years. We investigated the activity pattern of Arctic foxes in the experimental area (high snowmobile activity) by fitting GLMMs to a data set consisting of three years with camera-trap data. The fixed effect explanatory variables were year (2008, 2009, 2013; Year), the two time periods (Period: day or night) and Date as a continuous variable. All models included camera-traps and camera-day-ID as random intercepts, as described above. We used likelihood ratio tests to compare seven candidate models including an increasing number of additive fixed effects and the interactions Year × Period, and Year × Date (Supplementary Table S1).
Finally, we evaluated the effect of snowmobile traffic on Arctic fox populations at a large scale. We assessed whether Arctic fox diurnal activity patterns differed among three sites in the Arctic (Svalbard, Nenetsky and Yamal), all with low snowmobile traffic. The proportion of photographs with Arctic foxes from the control area in Nordenskiöld Land was compared with data from the two Russian sites, Nenetsky and Yamal. In Nenetsky the camera-traps were located in an area that is very rarely visited by humans on snowmobiles during the winter. In Yamal human presence is also limited and consists of only a small settlement with four to eight resident people in the study area, as well as a railroad and a winter road with low intensity of snowmobiles crossing the area. In Svalbard, observations were available only from one year (2013), but at the two Russian sites, observations were available for two years (2008, 2009). Since we had technical problems with the baits and the cameras in Nenetsky in 2009, we chose to use the year 2008 for the Russian sites. We fitted GLMMs by including the following fixed effect explanatory variables: Site (Svalbard [control area], Yamal and Nenetsky), Period (day or night) and Date (continuous). As above, camera-trap and camera-day-ID were included as nested random intercepts. Seven candidate models of increasing complexity including an increasing number of additive fixed effects and the interactions Site × Period, and Site × Date were compared using likelihood ratio tests (Supplementary Table S2).
Results
Effect of high and low snowmobile traffic at the local scale
We obtained 320 000 photographs in 2013, of which 170 958 (control area) and 133 452 (experimental area) were included in the analysis of Arctic fox diurnal activity pattern at the local scale. Of these, 13 003 (7.6%) and 10 423 (7.8%) yielded observations of Arctic foxes from the control and experimental area, respectively (for a summary see Supplementary Table S3). After dividing the 24-hour cycle into two periods, we calculated the proportion of photographs with Arctic fox for each period (i.e., two periods per day). Overall, Arctic foxes were recorded in 1071 periods of a total of 2214 periods. At the most, Arctic foxes were present on 140 of 144 photographs taken during a 12-hour period. Both areas had a higher number of photographs with Arctic foxes during the night, but the difference between night and day was larger in the experimental area (9.3% versus 6.1%) compared to the control area (8.2% versus 6.7%; ). The GLMM analysis confirmed this result. The likelihood ratio test showed a clear support for the interaction Period × Treatment in addition to Period, Treatment and Date as fixed effects, but not for the interaction Treatment × Date (). The selected model showed that during the day, the proportion of photographs with Arctic foxes was lower in both areas (). Moreover, the difference between night and day was larger in the experimental area, as there was a significant interaction Period × Treatment (−0.314, CI = −0.376; −0.253 on the logit scale). The odds ratio for the difference between day and night was 0.75 (95% CI, CI = 0.720–0.780) in the control area and 0.55 (CI = 0.522–0.573) in the experimental area. The assessment of diagnostic plots revealed that the distribution of the random effects camera-day-ID was somewhat bimodal. In addition, for six out of 20 cameras there was an indication for temporal autocorrelation of the residuals up to a lag of three days. Therefore, to have a robust assessment of CIs, we performed bootstrapping over blocks of five days for each camera-trap station and obtained a CI of −0.533 to −0.094 for the interaction Treatment × Period day (logit scale; a CI excluding 0 means that the interaction is significant). This shows that Arctic foxes concentrate their use of the baited camera-traps during the night (19:00–07:00) in areas with high snowmobile traffic, more so than in areas with less snowmobile traffic. Moreover, the number of photographs with Arctic foxes decreased significantly in both areas throughout the spring ().
Table 1. Model comparison and parameter estimates for selected model. (a) Candidate GLMMs applied to model the proportion of photographs with Arctic foxes during day (07:00–19:00) and night (19:00–07:00). Three fixed effect explanatory variables were used: Period (day or night), Date (a continuous day number starting with 1 January) and area (Treatment: control area with low snow mobile traffic and experimental area with high snow mobile traffic). Additive effects are indicated with + and interactions with ×. Models of increasing complexity were compared with likelihood ratio tests. Camera-trap station and the identity of each day at each camera-trap (camera-day-ID) were included in all models as random effects. The selected model is highlighted in boldface. (b) Parameter estimates for the selected GLMM explaining the proportion of photographs with Arctic fox per time period. Estimates are shown on the logit scale with standard errors and p values. The reference levels are night for the Period and Control for Treatment. The estimates represent contrasts to the reference level. Coefficients for random effects and residual variability are standard deviations.
Figure 3. (a) Average proportion of photographs with Arctic foxes during day (07:00–19:00) and night (19:00–07:00) in the two study areas on Nordenskiöld Land, Svalbard. Dark blue denotes the experimental area with high snowmobile traffic and light blue the control area with low snowmobile traffic. The error bars indicate 95% CIs estimated from binomial distributions. (b) Predicted proportion of photographs of Arctic foxes at camera-traps during the season in the experimental area (dark blue) and control area (light blue), for day and night in relation to camera-day-ID as predicted by the selected GLMM. Predictions were based on the fixed effect variable only.
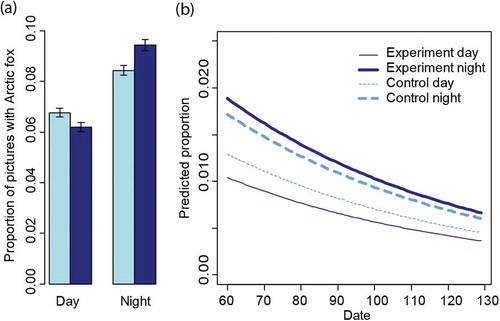
Temporal effects of snowmobile traffic on local scale
We obtained fewer photographs of good quality for analyses of data from the experimental area in 2008 (19 316) and 2009 (16 234). The reasons for this were a change in the time-lapse period in 2013 (5 minutes) compared to 2008 and 2009 (10 minutes), and technical problems with some of the cameras, resulting in bad quality photographs or periods with no pictures taken in 2008 and 2009. In 2008 and 2009, 189 (1%) and 643 (4%) photographs yielded observations of Arctic foxes, respectively. Compared to 2013 with 10 423 (7.8%) photographs of Arctic foxes, this indicates lower Arctic fox activity (or abundance) in 2008 and 2009 (). Despite this, the relative proportion of pictures with Arctic fox during the night and the day was more or less similar over all the three years in the experimental area (). The selected GLMM included Period, Date and Year, and the interaction Date × Year. There was no support for the interaction Year × Period (Supplementary Table S1). We observed a consistent pattern with lower Arctic fox activity during day compared to night in all years (odds ratio = 0.550, CI = 0.525–0.575; Supplementary Table S1). The only difference between the study years was seen in the interaction Year × Date, where 2009 had an increase in the number of photographs with Arctic foxes during the spring, while 2008 and 2013 experienced a decrease (). To check for an effect of the different time lapse used in 2013, we repeated the analysis including only every other picture from 2013. The results were similar and confirmed the lower proportion of pictures with Arctic foxes in 2009 and especially in 2008, the different trend over the spring in 2009 and the absence of an interaction Period × Year.
Figure 4. (a) Average proportion of photographs with Arctic foxes during day (07:00–19:00) and night (19:00–07:00) over three years (2008, 2009 and 2013) in the experimental area in Svalbard. The error bars indicate 95% CIs estimated from binomial distributions. (b) Change in proportion of photographs with Arctic foxes during the late winter and early spring in the years 2008, 2009 and 2013 as predicted based on the GLMM in the experimental area in Svalbard. Predicted proportions are shown for camera-day-ID and predictions were based only on the fixed effects variables.
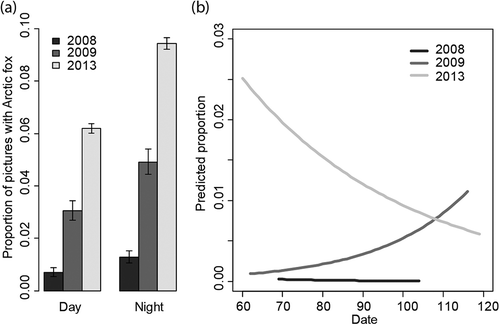
Large-scale comparison of effects of snowmobile traffic on Arctic fox populations
In Nenetsky, we obtained 20 140 photographs in 2008 and 6488 photographs in 2009. In 2008, 228 photographs yielded observations of Arctic foxes (1.1%), while only 15 photographs of Arctic foxes were obtained in 2009 (0.2%) because of problems with the bait and technical problems with the cameras. In Yamal, we obtained 40 035 photographs in 2008 and 40 292 in 2009, with 1403 (3.5%) and 567 (1.4%) photographs that yielded observations of Arctic foxes, respectively (for a summary see Supplementary Table S3). Arctic foxes were the most frequent species observed at the camera-traps in both areas (Rodnikova et al. Citation2010). For the comparison of the diurnal pattern with Svalbard (data from control area in 2013), we used only the data from 2008 from the Russian areas, when most pictures were taken and the presence of Arctic foxes was highest. There was a slight increase in the mean proportion of photographs with Arctic foxes during the night both in Nenetsky and in Yamal, but CIs for day and night overlapped largely (). The best GLMM model included an effect of Site (Nenetsky, Yamal and Svalbard), Date and Period, as well as the interactions Period × Site, and Date × Site (Supplementary Table S2). Arctic foxes used the bait approximately as much during the day as during the night in Yamal, resulting in a significant interaction Period × Site (Supplementary Table S2). For Nenetsky, the interaction was not significant, probably because of the small sample size from this site. The interaction Site × Date revealed that in Yamal and Nenetsky the proportion of photographs with Arctic foxes increased during the spring, while in Svalbard there was a decrease (). We also repeated this analysis using every other picture from Svalbard to account for the differences in time lapse, and obtained similar results as those presented above.
Figure 5. (a) Average proportion of photographs with Arctic foxes during day (07:00–19:00) and night (19:00–07:00) at the three Arctic study sites with low snowmobile traffic in Nordenskiöld Land (Svalbard), and in Nenetsky and Yamal (Russia). The error bars indicate 95% CIs estimated from a binomial distribution. (b) Predicted proportion of photographs with Arctic foxes during spring at the three study sites with low snowmobile traffic in Nordenskiöld Land in Svalbard, and in Nenetsky and Yamal in Russia, as predicted by the selected GLMM. Proportions were predicted for the fixed effect variables only.
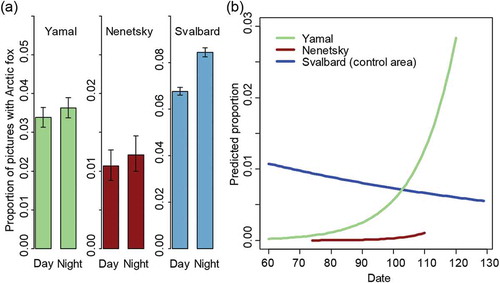
Discussion
Our results showed that Arctic foxes in Svalbard had different diurnal activity patterns at the camera-traps in the control and experimental areas, indicating a small-scale impact of snowmobile traffic. To test the consistency of this result, we compared our data from the experimental area to a similar study in the same area from 2008 and 2009 to account for potential inter-annual variation in environmental conditions (e.g., abundance of Arctic foxes, natural carcass availability, weather conditions). The temporal comparison showed that Arctic foxes had consistently similar diurnal activity patterns, also independent of differences in numbers of photographs each year. Thus, the time spent on a carcass during day and night was not affected by the size of the Arctic fox population, nor competition for carcasses. To test for differences in diurnal activity between Arctic fox populations on a larger scale, we compared our data from the control area in Svalbard (low snowmobile traffic) with two Russian sites, Nenetsky and Yamal, both with low snowmobile traffic. The Svalbard Arctic foxes had a different diurnal activity pattern, being more active during the night, compared to Arctic foxes in Yamal, that were equally active day and night. Although the activity pattern in Nenetsky appeared similar to that in Yamal, the difference between Nenetsky and Svalbard was not significant because of the low amount of data from Nenetsky.
The diverse factors that may influence the activity patterns of carnivores include the presence of competitors, accessibility of prey or food items, species plasticity and human disturbances (George & Crooks Citation2006; Berger Citation2007). However, the few studies of Arctic fox diurnal activity patterns that have been undertaken have yielded divergent results. Eberhardt et al. (Citation1982) report Arctic foxes in Alaska to be more nocturnal than diurnal in summer and Frafjord (Citation1992) reports the same tendency for foxes in Svalbard for the winter, but with large individual variations. A more recent study in Svalbard showed no circadian rhythms in body temperature and heart rate in wild Arctic foxes held in captivity both summer and winter (Fuglei Citation2000), indicating no specific diurnal activity pattern. Our results showing that Arctic foxes concentrate their use of reindeer carcasses to the night (19:00–07:00) in areas with high snowmobile traffic, more so than in areas with less snowmobile traffic, are therefore inconsistent with previous studies.
Similar shifts in diurnal activity patterns due to human disturbances are also found in other mammals. For instance, George & Crooks (Citation2006) demonstrated that the bobcat (Lynx rufus) shifted daily activity patterns to become more nocturnal in high-use recreational areas, while Creel et al. (Citation2002) found higher stress hormone levels in elk (Cervus elaphus) and wolves (Canis lupus) in areas and during times of the day with heavy snowmobile traffic. Despite these responses, Creel et al. (Citation2002) found no evidence that the snowmobile traffic was negatively affecting the population dynamics of the species in the study area.
Wildlife reactions to snowmobiles are mainly related to the sound from the engine of the vehicle (Reimers et al. Citation2003). In Svalbard, a guided tourist group consists of a column of up to 25 snowmobiles and the sound may be a substantial disturbance. Currently, a good estimate of the total snowmobile traffic load in Svalbard is not available because only the organized tourist trips are registered in the official statistics (Governor of Svalbard Citation2014). However, the number of hire-days of snowmobiles is available and can be used as a proxy for snowmobile traffic. In 2008 and 2009 approximately 9000 hire-days were registered per winter season while in 2013 it had increased to 11 000 (Governor of Svalbard Citation2014). In comparison, in the study of elk and wolf mentioned above (Creel et al. Citation2002), the number of snowmobiles varied between 15 000 and 25 000 per winter season—twice as much as in Svalbard.
The consistent temporal Arctic fox activity pattern across study years in the experimental area supports our hypothesis. However, the large differences in the proportion of photographs with Arctic foxes between years need to be discussed. Both the Arctic fox and reindeer populations in the experimental area are monitored annually (Ims et al. Citation2014). In 2008, the natural mortality of reindeer was high, which resulted in large numbers of carcasses (206 were observed) available on the tundra. This year contrasts with the other two years, when there were considerably fewer carcasses available (17 in 2009 and 26 in 2013). Arctic fox densities also played a role: the Arctic fox population density was comparatively low in 2008 but comparatively high in 2013 (Fuglei & Pedersen unpubl. data). In combination, differences in the density of Arctic foxes and the availability of reindeer carcasses likely explain the low number of foxes at the camera-traps in 2008 and the high number in 2013. Despite the low abundance of Arctic foxes and the low numbers of foxes at the camera-traps in 2008, the baited camera-traps were still more frequently visited at night.
The differences in diurnal activity between Arctic fox populations experiencing low snowmobile traffic at a large scale were surprising, since in both Svalbard and northern Russia daylight increases rapidly in spring, and in both areas the predator guilds are dominated by Arctic foxes during winter (Rodnikova et al. Citation2010). However, there may be alternative explanations for the differences between the study sites. Winter temperatures at the Russian sites are much lower than in Svalbard (data from www.worldclim.org). The Russian foxes may experience more energy demands and therefore need to search for food on a 24-hourly basis. Additionally, food resource availability differs between the sites. Arctic foxes in Svalbard live in an ecosystem lacking small rodents and competitors (Fuglei et al. Citation2003; Eide et al. Citation2012), which is not the case in Russia (Pokrosvky et al. Citation2014; Sokolov et al. Citation2016). The scarcity and unpredictability of food resources in Svalbard makes the foxes behave as generalist scavengers, feeding opportunistically (Fuglei et al. Citation2003; Eide et al. Citation2012). One study in Svalbard showed that Arctic foxes had huge variation in fat content during winter (from 5% to 40% of skinned body mass; Prestrud & Nilssen Citation1992), indicating a need for an opportunistic search for food.
The more plausible explanation for the differences found in this study relate to anthropogenic disturbances, in that the Arctic fox population in both areas (control and experimental) in Svalbard are affected by snowmobile traffic, but foxes from the area of lower snowmobile use are affected to a lesser degree. The experimental area covers the main snowmobile trail out of Longyearbyen (the main settlement in Svalbard), while the control area was located 65 km to the south-west. Arctic foxes are able to move over vast areas during winter: a satellite study in the Canadian Arctic showed that they can move up to 4000 km from February to July (Tarroux et al. Citation2010). A satellite study in Svalbard showed that Arctic foxes moved on average 6.6 km per day with individual variation from 0.5 km to 72.6 km (Fuglei et al. Citation2016). It is therefore likely that the foxes in our study were able to move across Nordenskiöld Land in a relatively short time. Hence, Arctic foxes in the entire Nordenskiöld Land region have likely encountered snowmobile traffic and may have changed their activity patterns accordingly.
Whether snowmobile traffic has affected the diurnal behaviour patterns of Arctic foxes in Svalbard to the extent that it has a negative impact on the Arctic fox population dynamics through changed survival or reproduction remains to be studied. Data from an annual monitoring programme that includes inspections of active breeding dens show no indication of a decline in the Arctic fox population in Svalbard (Ims et al. Citation2014). Therefore, we cannot confirm any regional negative effects of snowmobile traffic on the Arctic fox population.
Conclusion
Our results suggest that snowmobile traffic has changed the diurnal activity of the Arctic fox in Nordenskiöld Land, Svalbard. However, the study did not prove negative impacts at the population level since the abundance of foxes did not decline. We lack knowledge about the cumulative effects of snowmobile traffic, harvesting (Fuglei et al. Citation2016), pollutants (e.g., Aas et al. Citation2014; Andersen et al. Citation2015) and climate change (Hansen et al. Citation2013) on the Arctic fox population in Svalbard. The changes in climate are happening faster in the Arctic than any other place on Earth (Nordli et al. Citation2014), and future impacts on species and ecosystems are difficult to predict.
Supplementary material
Download PDF (189.5 KB)Acknowledgements
We thank the following for participating in the fieldwork: in Svalbard, K. Johannsen, T. Sandal, E. Johansen, R. Krapp and H. Kauko; in Yamal, the Laptander family, V.N. Sidorov, V. Shtro, S. Osokin, G. Popov and V.A. Sokolov; in Nenetsky, A. Glotov and the Nenetsky State Nature Reserve.
Disclosure statement
No potential conflict of interest was reported by the authors.
Supplemental material
The supplemental material for this article can be accessed here
Additional information
Funding
References
- Aas C.B., Fuglei E., Herzke D., Yoccoz N.G. & Routti H. 2014. Effect of body condition on tissue distribution of perfluoroalkylated substances (PFASs) in Arctic fox (Vulpes lagopus). Environmental Science & Technology 48, 1–10.
- Amstrup S.C. 1993. Human disturbance of denning polar bears in Alaska. Arctic 46, 246–250.
- Andersen M. & Aars J. 2008. Short-term behavioural response of polar bears (Ursus maritimus) to snowmobile disturbance. Polar Biology 31, 501–507.
- Andersen M., Fuglei E., König M., Lipasti I., Pedersen Å.Ø., Polder A., Yoccoz N.G. & Routti H. 2015. Levels and temporal trends of persistent organic pollutants (POPs) in Arctic foxes (Vulpes lagopus) from Svalbard in relation to dietary habits and food availability. Science of the Total Environment 511, 112–122.
- Barrueto M., Ford A.T. & Clevenger A.P. 2014. Anthropogenic effects on activity patterns of wildlife at crossing structures. Ecosphere 5, doi: 10.1890/ES13-00382.1
- Bates D., Martin M., Bolker B. & Walker S. 2015. Fitting linear mixed-effects models using lme4. Journal of Statistical Software 67, 1–48.
- Berger J. 2007. Fear, human shields and the redistribution of prey and predators in protected areas. Biology Letters 3, 620–623.
- Born E.W., Riget F.F., Dietz R. & Andriashek D. 1999. Escape responses of hauled out ringed seals (Phoca hispida) to aircraft disturbance. Polar Biology 21, 171–178.
- CAFF 2013. Arctic biodiversity assessment. Status and trends in Arctic biodiversity. Akureyri: Conservation of Arctic Flora and Fauna.
- Creel S., Fox J.E., Hardy A., Sands J., Garrott B. & Peterson R.O. 2002. Snowmobile activity and glucocorticoid stress responses in wolves and elk. Conservation Biology 16, 809–814.
- Eberhardt L.E., Hanson W.C., Bengtson J.L., Garrot R.A. & Hanson E.E. 1982. Arctic fox home range characteristics in an oil-developed area. Journal of Wildlife Management 46, 183–190.
- Eckstein R.G., O ̓Brien T.F., Rognstad O.J. & Bollinger J.G. 1979. Snowmobile effects on movements of white-tailed deer: a case study. Environmental Conservation 6, 45–51.
- Ehrich D., Lecomte N., Fuglei E., Ims R.A. & Yoccoz N.G. 2011. Arctic predators as indicators of tundra ecosystem state. In O. Orheim & K. Ulstein (eds.): The international polar year 2007-2008. The Norwegian contribution. Pp. 116–119. Oslo: Norwegian Research Council.
- Eide N.E., Stien A., Prestrud P., Yoccoz N.G. & Fuglei E. 2012. Reproductive responses to spatial and temporal prey availability in a coastal Arctic fox population. Journal of Animal Ecology 81, 640–648.
- Erb P.L., McShea W.J. & Guralnick R.P. 2012. Anthropogenic influences on macro-level mammal occupancy in the Appalachian trail corridor. PLoS One 7, e2574, doi: 10.1371/journal.pone.0042574
- Frafjord K. 1992. Behavioural ecology and behavioural energetics in the Arctic fox Alopex lagopus. PhD thesis, Museum of Zoology, University of Bergen.
- Freddy D.J., Bronaugh W.M. & Fowler M.C. 1986. Responses of mule deer to disturbance by persons afoot and snowmobiles. Wildlife Society Bulletin 14, 63–68.
- Fuglei E. 2000. Physiological adaptations of the Arctic fox to High Arctic conditions. PhD thesis, Division of General Physiology, University of Oslo.
- Fuglei E., Berteaux D., Pedersen Å.Ø. & Tarroux A. 2016. Arctic fox spatial ecology related to harvest management. Final report to Svalbard Environmental Protection Fund. Brief Report 35. Tromsø: Norwegian Polar Institute.
- Fuglei E., Øritsland N.A. & Prestrud P. 2003. Local variation in Arctic fox abundance on Svalbard, Norway. Polar Biology 26, 93–98.
- Gabrielsen G.W. & Smith E.N. 1995. Physiological responses of wildlife to disturbance. In R.L. Knight & K.J. Gutzwiller (eds.): Wildlife and recreationists. Coexistence through management and research. Washington, DC: Island Press.
- Gauthier G. & Berteaux D. (eds.) 2011. ArcticWOLVES: Arctic Wildlife Observatories Linking Vulnerable Ecosystems. Final synthesis report. Quebec City: Centre for Northern Studies, University of Laval.
- George S.L. & Crooks K.R. 2006. Recreation and large mammal activity in an urban nature reserve. Biological Conservation 133, 107–117.
- Gese E.M., Dowd J.L.B. & Aubry L.M. 2013. The influence of snowmobile trails on coyote movements during winter in high-elevation landscapes. PLoS One 8, e82862, doi: 10.1371/journal.pone.0082862
- Governor of Svalbard 2014. Reiselivsstatistikk for Svalbard 2014. (Travel industry statistics for Svalbard 2014.) Longyearbyen: Office of the Governor of Svalbard.
- Hamel S., Killengreen S.T., Henden J.-A., Eide N.E., Roed-Eriksen L., Ims R.A. & Yoccoz N.G. 2013. Towards good practice guidance in using camera-traps in ecology: influence of sampling design on validity of ecological inferences. Methods in Ecology and Evolution 4, 105–113.
- Hansen B.B., Grøtan V., Aanes R., Sæther B.-E., Stien A., Fuglei E., Ims R.A., Yoccoz N.G. & Pedersen Å.Ø. 2013. Climate events synchronize the dynamics of a resident vertebrate community in the High Arctic. Science 339, 313–315.
- Ims R.A., Alsos I.G., Fuglei E., Pedersen Å.Ø. & Yoccoz N.G. 2014. An assessment of MOSJ – the state of the terrestrial environment in Svalbard. Rapportserie 144. Tromsø: Norwegian Polar Institute.
- Killengreen S.T., Strømseng E., Yoccoz N.G. & Ims R.A. 2012. How ecological neighborhoods influence the structure of the scavenger guild in Low Arctic tundra. Diversity and Distributions 18, 563–574.
- MacArthur R.A., Johnston R.H. & Geist V. 1979. Factors influencing heart rate in free-ranging big horn sheep: a physiological approach to the study of wildlife harassment. Canadian Journal of Zoology 57, 2010–2021.
- Ministry of Justice and the Police 2010. Svalbard. Report 22 (2008-2009) to the Storting. Oslo: Norwegian Ministry of Justice and the Police.
- Muhly T.B., Semeniuk C., Massolo A., Hickman L. & Musiani M. 2011. Human activity helps prey win the predator–prey space race. PLoS One 6, e17050, doi: 10.1371/journal.pone.0017050
- Neumann P.W. & Merriam H.G. 1972. Ecological effects of snowmobiles. Canadian Field-Naturalist 86, 207–212.
- Nordli Ø., Przybylak R., Ogilvie A.E.J. & Isaksen K. 2014. Long-term temperature trends and variability on Spitsbergen: the extended Svalbard Airport temperature series, 1898-2012. Polar Research 33, article no. 21349, doi: 10.3402/polar.v33.21349
- Pokrosvky I.G., Ehrich D., Ims R.A., Kulikova O., Lecomte N. & Yoccoz N.G. 2014. Diet, nesting density, and breeding success of rough-legged buzzards (Buteo lagopus) on the Nenetsky Ridge, Arctic Russia. Polar Biology 37, 447–457.
- Prestrud P. & Nilssen K. 1992. Fat deposition and seasonal variation in body composition of Arctic foxes in Svalbard. Journal of Wildlife Management 56, 221–233.
- R Development Core Team 2015. R: a language and environment for statistical computing. Vienna: R Foundation for Statistical Computing.
- Reid W.V., Mooney H.A., Cropper A., Capistrano D., Carpenter S.R., Chopra K., Dasgupta P., Dietz T., Duraiappah A.K., Hassan R., Kasperson R., Leemans R., May R.M., McMichael A.J., Pingali P., Samper C., Scholes R., Watson R.T., Zakri A.H., Shidong Z., Ash N.J., Bennett E., Kumar P., Lee M.J., Raudsepp-Hearne C., Simons H., Thonell J. & Zurek M.B. 2005. Ecosystems and human well-being. Synthesis. Washington, DC: Island Press.
- Reimers E., Eftestol S. & Colman J.E. 2003. Behavior responses of wild reindeer to direct provocation by a snowmobile or skier. The Journal of Wildlife Management 67, 747–754.
- Rodnikova A., Fuglei E., Ims R.A., Killengren S., Sokolov A. & Goltsman M. 2010. Predator guild structure in different tundra ecosystems in winter. Poster presented at the International Polar Year Oslo Science Conference, 8–12 June, Oslo.
- Salek M., Drahnikova L. & Tkadlec E. 2014. Changes in home range sizes and population densities of carnivore species along the natural to urban habitat gradient. Mammal Review 45, 1–14.
- Sokolov A.A., Sokolova N.A., Ims R.A., Brucker L. & Ehrich D. 2016. Emergent rainy winter warm spells may promote boreal predator expansion into the Arctic. Arctic 69, 121–129.
- Tangberg A. 2016. The Svalbard reindeer (Rangifer tarandus platyrhynchus) and snowmobile tracks. Quantifying spatial and temporal patterns in avoidance behaviour. Master’s thesis, Norwegian University of Life Sciences, Trondheim.
- Tarroux A., Berteaux D. & Bety J. 2010. Northern nomads: ability for extensive movements in adult Arctic foxes. Polar Biology 33, 1021–1026.
- Tyler N.J.C. 1991. Short-term behavioural responses of Svalbard reindeer (Rangifer tarandus platyrhyncus) to direct provocation by a snowmobile. Biological Conservation 56, 179–1940