ABSTRACT
The parametric and traditional CAD approaches are fundamental for pattern construction. This paper empirically analyses their geometric data entry, construction space, methods, workflow, and outputs, highlighting differences between the two. The results show that the traditional approach offers faster data entry but lacks editability, while the parametric approach prioritizes data modification efficiency despite requiring more time for initial entry. The traditional approach allows quicker construction but becomes time-consuming for significant pattern changes, whereas the parametric approach, although slower to construct, offers editable blocks for efficient modification. The parametric approach captures both geometric shapes and block logic, in contrast to the traditional approach's focus solely on geometric shapes. The parametric approach supports the creation of custom garments, reducing waste and promoting sustainability, while the traditional approach leads to static blocks necessitating recreation for each new wearer. Accordingly, educational institutions should spearhead the transition to the parametric approach.
1. Introduction
Most consumers in today's garment market would like to customise the fit of their clothing (Jin et al., Citation2023;Yang, Zhang, & Shan, Citation2007). The trend towards customisation, or bespoke fashion, has emerged as the most significant tendency in the apparel market (Yan and Kuzmichev, Citation2020). Customisation is a manufacturing technique aimed at delivering personalised, individually tailored, and top-notch apparel products to consumers (Baran & Galka, Citation2016;Moore, Citation2020) . It helps apparel businesses increase consumer satisfaction and reduce returns of ill-fitting garments, thus becoming more competitive (Gu, Yu, & Behbahani, Citation2018; Yang et al., Citation2007). Nevertheless, the apparel business still faces difficulties in satisfying consumers’ demands for well-fitting clothing (Gu et al., Citation2018). This is because the first step in producing high-quality personalised clothing is creating patterns according to a customer's exact requirements posing difficulties in terms of unreasonable time costs, labour costs, and resource wastage if conducted manually (Jin et al., Citation2023). The utilisation of pattern construction technologies has facilitated the customisation process; however, the traditional use of these technologies in the creation of personalised patterns still requires a significant amount of manual work and effort. Thus, the adoption of automated pattern technology is a requirement to facilitate pattern customisation because it connects consumer demands with a manufacturer's ability to produce clothing in a more efficient and timely manner. Advances in 2-D CAD technology have brought about a degree of automation to resizing patterns and making and applying changes to them, making a fundamental contribution to customisation (Xu, Thomassey, & Zeng, Citation2020). Despite the multitude of advancements in pattern-making technologies, it is noteworthy that the construction of patterns for customised apparel still relies predominantly on a limited number of approaches. These approaches serve as the foundation for various methods used in digital pattern generation and customisation techniques.
Therefore, the primary aim of this paper is to pave the way for more efficient and effective pattern construction processes. This will be achieved by classifying and evaluating current pattern construction approaches and customising techniques, with a particular focus on the traditional CAD and parametric approaches, which serve as the fundamental approaches in pattern construction. Through an empirical comparison that emphasises the principles underlying the construction process rather than focusing solely on the principles imposed by specific technologies, the paper offers valuable insights into the strengths and limitations of the comparable approaches. It also provides insights on how to overcome the identified limitations, ultimately enhancing the ability to create customised apparel that better caters to individual needs and preferences.
2. Research design
This paper utilises an exploratory empirical approach, where the researchers directly observe and investigate a specific area previously underexplored in-depth by others (Daneshgar, Citation2023; Mwenisongole & Mligo, Citation2018).
To achieve the research aim, two research strategies were employed. The first strategy is archival research, which involves analyzing existing scholarly documents to gather information on a topic (Leach, Citation2018). This strategy helped in classifying pattern construction approaches and customisation techniques. The thorough review of literature, scholarly articles, and previous studies provided a comprehensive understanding of the subject matter and helped in identifying gaps in existing knowledge and contextualising the empirical comparison within the broader literature. By citing some of the primary research that utilised objective data collection methods, the credibility and validity of the paper's findings were further supported and enhanced.
The second research strategy was a hands-on experiment, wherein researchers actively engage in hands-on practical activities instead of delegating them to others activities (HC Publishers, Citation2016). This strategy allowed the researchers to personally construct four pattern blocks (sleeve, trousers, skirt, and bodice) using both comparable approaches. These patterns served as the artifacts that were subsequently evaluated and compared. The selection of these specific patterns was purposeful as they form the foundation for covering major body areas. Additionally, these blocks can be resised and manipulated to create a wide range of customised garment styles and exhibit varying levels of geometric complexity. With the inclusion of essential geometric elements such as darts, straight lines, and curved lines, the comparison between traditional CAD and parametric constructions was critically examined.
During this direct involvement, empirical data was collected through observation and self-reported, taking into account significant criteria including accuracy, fit, complexity, effort and time consumption, and ease of modification. To ensure objectivity, the selected criteria were in line with established criteria utilised by scholars, and they were explicitly defined and measurable. Transparent reporting of comparative figures enabled readers to evaluate the credibility and dependability of the findings.
The collected data were then analyzed using two approaches: the thematic approach and the comparative approach. The thematic approach identified key themes within the data, with a specific focus on construction inputs and pattern construction outputs. Three sub-themes were identified under construction inputs: geometric data entries, construction space and process, and construction workflow. The analysis was limited to these themes because the empirical comparison aimed to investigate the underlying principles of the construction process in comparable approaches, rather than focusing solely on principles imposed by specific technologies. These themes are firmly grounded in existing literature as they key factors that differentiate the construction processes of both approaches (Andriasyan, Moyano, Nieto-Julián, & Antón, Citation2020; Gindis & Kaebisch, Citation2019; Stephen Samuel, Citation2006; Yang et al., Citation2007). Understanding these themes contributes to a better understanding of the characteristics of the patterns, and The comparative approach was utilised to systematically analyze and compare these themes, resulting in a comprehensive evaluation of the strengths and limitations associated with each approach. This analysis also offers valuable insights into overcoming these limitations and enhancing the construction process.
3. Literature/archival research review
This research is founded on an extensive literature review that explores various construction approaches and adjustment techniques utilised in pattern customisation, as well as 2D pattern construction technologies. Therefore, this section will be divided into three subsections to evaluate these three dimensions of the literature. The purpose of this evaluation is to classify pattern construction approaches and justify why this empirical comparison focuses specifically on the parametric and traditional digital approaches using 2D CAD technologies. Furthermore, it will help identify the research gap and highlight the contribution of this paper in addressing it.
3.1. Pattern construction approaches and adjustment techniques for customised apparel
There are multiple approaches and techniques for pattern construction and adjustment that cater to customised apparel, allowing for precise alignment with individual measurements, shape, and preferences to ensure an optimal fit (Istook, Citation2002). These approaches include manual, traditional digital, and advanced digital approaches.
Traditional manual approach encompasses the construction of a 2-D basic block pattern by hand on a paper that fits a standard body size, followed by physical customisation to its size, shape, or style based on specific body’s measurements using different techniques (Datta & Seal, Citation2018;Almond & Power, Citation2018). Two manual adjustment techniques utilised by pattern makers are the slashing-and-spreading technique and the pivot technique. In the slashing-and-spreading technique, the paper pattern is strategically cut and spread apart or overlapped to accommodate different measurements (Moniruzzaman & Rita, Citation2022), as demonstrated in . The pivot technique involves anchoring the pattern and adjusting its width by moving it in various directions (Zieman, Citation2009), as depicted in . Both techniques necessitate skill, precision, and a comprehension of pattern construction principles. Ensuring proportionality with the original pattern is essential when applying these adjustments.
With digital pattern-making software, manual construction can now be executed digitally using digital tools, known as the traditional digital approach. Understanding traditional alteration techniques remains valuable for pattern makers, allowing them to adapt concepts effectively to digital tools. The traditional digital approach offers customisation through two techniques. One technique involves using mathematical formulas and anthropometric data to calculate appropriate sizes and make adjustments (Liu, Xie, & Song, Citation2017). This requires a strong understanding of structural theory, practical experience, and CAD proficiency. Another technique involves using graded patterns and alteration tables to adapt patterns to individuals with specific measurements (Yang et al., Citation2007).
Parametrisation has emerged as a key approach in advanced digital construction, enabling the development of various pattern generation methods. It involves defining parameters to establish the relationship between pattern inputs and outputs. Through parametric construction, dynamic and geometrically associative patterns can be generated (Caetano, Santos, & Leitão, Citation2020; Casini, Citation2022; Touloupaki & Theodosiou, Citation2017). This geometric association automates resising and adjustment processes, facilitating the creation of patterns that accurately adapt to individual body measurements and shape. Parametric construction offers a superior alternative to traditional construction in customisation operations for bespoke garments, where patterns are uniquely created and cut for each wearer (Anderson, Citation2009).
Parameterisation has been used by researchers to develop innovative methods for automatically generating customised digital patterns for apparel (Xiu, Wan, & Cao, Citation2011; Zhang, Innami, Kim, & Takatera, Citation2015; Kang & Kim, Citation2019; Jin et al., Citation2023). AI systems are utilised in one method to automatically generate patterns by creating a resizable parametric pattern. Another method involves scanning and flattening a 3D model, then transforming it into a 2D parametric pattern using specialised software (Huang et al., Citation2012). These methods provide alternative approaches for pattern generation and customisation based on individual requirements. It's important to note that these methods deviate from traditional construction processes, as the roles of traditional or parametric construction are programmed into the system, reducing the need for extensive pattern construction knowledge and skills from the user's perspective.
The made-to-measure (MTM) technique is commonly used to adjust existing patterns and create clothing products that are tailored to individual users (Daanen & Hong, Citation2008). This technique takes into account additional body dimensions and individual preferences for textiles and colours during the alteration process. The aim is to prioritise the individual early in the clothing production chain. It's important to note, however, that the construction process in MTM systems still relies on manual, traditional digital, or parametric construction approaches (Xiu et al., Citation2011; Lim & Istook, Citation2012; Jin et al., Citation2023).
Based on this evaluation, it becomes evident that the traditional digital and parametric approaches serve as fundamental construction methods and foundational for other pattern generation and customising techniques. By focusing on these two approaches, a comprehensive examination and comparison of their advantages and limitations can provide valuable insights and guidance for future advancements in pattern construction. These findings not only contribute to the field of pattern-making but also have broader implications, shaping the future development of the pattern construction process.
3.2. Pattern-making 2D CAD systems for customisation
A 2D CAD pattern-making software is a 2D vector-based system that allows pattern makers to create, import, and modify 2D pattern pieces using drafting, digitising, or modifying existing blocks with pattern-making rules (Jhanji, Citation2018; Moore, Citation2020).
Most pattern-making CAD systems used in academia and industry are 2D, and their integration has played a crucial role in enhancing the efficiency and accuracy of pattern construction and manipulation (Jhanji, Citation2018; Moniruzzaman & Rita, Citation2022). These CAD systems offer specialised functions for altering existing patterns, such as manipulating darts and adding fullness, along with generic tools commonly found in vector-based software programs (Moniruzzaman & Rita, Citation2022; Moore, Citation2020). Pattern-making 2D CAD systems can be categorised into three main groups based on their construction approaches: traditional systems, parametric systems, and hybrid systems. The evaluation of these systems was primarily based on personal experience in using them due to limited information available in the literature.
Traditional 2D CAD systems, such as Optitex, are software programs that emulate manual pattern-making techniques in a digital environment (Stephen Samuel, Citation2006; Optitex, n.d.). While lacking feature-driven geometry functions, these systems provide tools and features that simulate the traditional drafting process, allowing pattern makers to construct patterns digitally using digital equivalents of manual pattern-making tools like rulers and pencils.
Parametric CAD systems automate pattern modifications and resising using predefined parameters. Seamly2D and Valentina are 2D parametric CAD systems in garment pattern construction. They employ the point-to-point construction method, where users sequentially define key points from start to end. For instance, in Seamly2D, a rectangle is constructed by developing points based on distance and angle from the datum point. The software prompts users to calculate distances using variables. These points form the rectangle's sides, and intersections complete it. This streamlines pattern construction, reducing complexity and enhancing efficiency.
Hybrid CAD systems combine traditional and parametric approaches, offering versatile tools for pattern making (Stephen Samuel, Citation2006). Lectra Modaris is an example of a hybrid CAD system that utilises a track-based method for parametric construction. In this method, users create a track and move the pattern along it for adjustments and modifications. While similar to Seamly2D and Valentina in concept, the specific steps differ. Constructing a parametric rectangle in Lectra Modaris involves two stages.
In the first stage, called ‘Creating the Frame or Track for the Parametric Rectangle’, the process begins by generating a datum point as the starting point. Then, the linked function is activated to establish connections between elements. A horizontal line is drawn to create one side of the rectangle, followed by a vertical line to complete the frame. Moving to the second stage, known as ‘Constructing the Parametric Rectangle’, the chart manager is set up to manage parameters and variables. Points are developed along the vertical and horizontal lines created earlier. These points are then linked to the appropriate axis for proper alignment. Finally, vertical and horizontal lines are drawn to connect the established points, forming the parametric rectangle.
In this paper, understanding and comparing different CAD systems was crucial to select suitable software for the study's objectives and establish a foundation for analyzing comparable approaches. This comparison highlighted construction principles, distinctions, and functionalities of CAD systems. Analyzing the construction process of parametric rectangles in Seamly2D and Lectra Modaris revealed that Seamly2D offers a simpler and faster approach with the same outcome. Due to its advantages in ease of use, speed, and comparable outcomes, Seamly2D was preferred for constructing parametric patterns in the empirical comparison, reflecting the aim of adopting an efficient and user-friendly approach to pattern making.
3.3. Existing comparative research on construction approaches for customised patterns
Evaluating previous comparative research was crucial in this paper. We analyzed their contributions and limitations, building upon their findings and identifying areas for further investigation. This contributed informative perspectives and unique viewpoints to the field's existing knowledge. Comparing our findings to their results, obtained through objective methods, increased result accuracy and reliability.
In the study conducted by Yang et al. (Citation2007), a theoretical comparison between the parametric approach and the traditional grading approach was presented, focusing on pattern alterations. The parametric pattern was modified by adjusting parameter values while considering topology structures. The researchers identified four categories of structure parameters: measurement parameters, style parameters, graphical variables, and compound parameters. Manipulating these parameters allows the generation of new patterns through dimension-driven processes. The study argued that the parametric approach improves efficiency in pattern construction and alteration by enabling convenient generation of individual patterns through geometric parameter adjustments. On the other hand, the traditional grading approach involves multiple steps such as identifying alteration points, establishing numbering conventions, formulating alteration rules, and ensuring accuracy, making it comparable to manual pattern construction.
In their comparative study, Huang et al. (Citation2012) introduced a novel parametric approach for generating fit-ensured block patterns and compared it to the traditional approach. The proposed method utilised a piecewise B-spline curve model to parameterise a human model, allowing for body recognition and feature alignment. A 3D wireframe was aligned with pattern-critical body features, and a two-step wireframe deformation scheme optimised ease distribution and alignment. The 3D block garments were created using a boundary triangulation algorithm and then flattened to produce 2D block patterns suitable for industrial use. The effectiveness of the approach was validated by generating customised block patterns for different body shapes and comparing the fitting performance with traditional pattern-construction approaches such as the British and Japanese methods. The results demonstrated that the proposed parametric approach offered advantages such as flexible ease distribution, precise fit and comfort control, proper alignment of body features for an appealing appearance and balanced drape, distortion-free 2D patterns suitable for manufacturing, computational efficiency, and suitability for online applications.
Xiu et al. (Citation2011) conducted a comparison that aligns with previous research, confirming the advantages of the parametric approach over the traditional approach in terms of efficiency and flexibility. The parametric approach enables the automatic generation of patterns with consistent topological structures and allows for the optimisation of specific patterns in an interactive graphical environment. These capabilities make the parametric approach beneficial for both mass production and mass customisation, as it can produce standardised patterns as well as individual patterns tailored to different body measurements. The study emphasises the potential of the parametric approach in apparel pattern construction and recommends its adoption in the industry.
The aforementioned studies have identified differences between the parametric and traditional approaches, particularly emphasising the importance of the parametric construction approach for customisation needs. However, it is important to recognise that certain differences discussed in these studies are influenced by the specific technologies utilised rather than solely by the construction principles themselves. For instance, Yang et al. (Citation2007) classified structure parameters into different categories, which may not be applicable to all parametric systems, including the one used in this paper, namely Seamly2D.
Furthermore, these studies often lack empirical analyses that directly compare the inputs and outputs of parametric construction to the conventional approach, independent of specific technologies and their impacts. Such a comparison is crucial to comprehend the differences in inputs and outputs and to identify the advantages, disadvantages, and limitations of each approach. Without such analyses, it is difficult to gain a comprehensive understanding and provide valuable insights for the advancement of pattern construction.
Therefore, this study aims to fill this void by conducting an empirical comparison between parametric construction and the traditional CAD approach. The comparison will be guided by criteria derived from specialised and multidisciplinary literature, which offers relevant insights into garment pattern construction.
4. Methodology
The research commenced by constructing the selected patterns using two comparable construction approaches. The construction of both traditional and parametric blocks involved the utilisation of the Aldrich method (2015). This method was chosen for its accessibility and extensive use in academia and the apparel industry (Alrushaydan et al., Citation2020; Lim & Cassidy, Citation2017). The measurement data utilised for pattern construction was obtained from an Alvanon UK Size 12 female dress form, which is widely recognised as an industry standard (). It is important to note that the measurements and method employed in pattern construction are solely construction requirements and do not have any impact on the research outcomes, as the study does not involve direct fit evaluation.
Table 1. Measurements required to draft the blocks from Alva 12.
The researchers constructed Aldrich parametric and traditional blocks utilising the calculations, data entry methods, geometric principles, and anatomical considerations illustrated in . It should be noted that the construction steps were numbered sequentially and each point, along with the calculation employed to develop it, were presented in the same colour.
5. Results and discussion
The comparative analysis of the inputs and outputs of parametric and traditional CAD approaches reveals several distinctions, which are tabulated and subsequently explicated in the succeeding sections. All terminologies utilised in the will be adequately defined in their respective sections to enhance clarity and comprehension.
Table 2. Summary of the comparison’s outputs.
5.1. Construction inputs
The present section focuses on investigating three essential construction inputs, namely geometric data entry, construction space and process, and construction workflow. These inputs constitute the various stages of a block construction, and their thorough examination is indispensable for identifying potential issues that may arise during the construction process and devise effective strategies to mitigate them. Additionally, an in-depth understanding of these inputs can lead to the development of more efficient and effective construction methods and workflows. Lastly, this investigation can provide valuable insights into the similarities and differences between traditional and parametric CAD construction methods, facilitating the selection of the most appropriate approach for a given project.
5.1.1. Geometric data entry
Geometric data entry refers to the procedures employed to input the desired pattern into the IT program (Gindis & Kaebisch, Citation2019). This data includes the length, type, and angle of each geometric element in the block. In the traditional CAD approach, entering geometric data is easier and faster than the parametric one. This is because it is only necessary to enter the data of the required elements directly and manually via the keyboard, so it appears on the command line as editable data. Nevertheless, after pressing enter, this data is not editable anymore. This means that the entered data is not reusable. Thus, to change or modify the entered geometric data, the user needs to delete what has been constructed using this data and reenter the data again.
In the parametric approach using Seamly2D, the geometric data is also entered manually via the keyboard, however, it may take longer to edit than the traditional approach. The reason is that each element (length, angle, base point, and label) should be pre-specified, and the data appears on the screen as a ‘properties editor’ which can be permanently editable as shown in .
The reusability and editability of geometric data make the parametric approach more efficient than the traditional approach in the customisation process. This is attributed to the parametric approach's ability to facilitate faster and more streamlined resising and adjustments to patterns which are crucial requirements for customisation. Previous research studies, such as Bao et al. (Citation2021);Huang et al. (Citation2012) Yang et al. (Citation2007), have provided evidence supporting this finding.
5.1.2. Construction space and process
In the context of pattern construction CAD systems, construction space can be defined as the workspace where a pattern is created using drafting tools, in some software this is referred to as a sheet. Both construction methods build elements in a 2D vector space, allowing the development of patterns using simple elements like lines and Bézier curves in the Cartesian (x, y) coordinate system. In contrast to manual methods, this 2D space is resizable to the requirements of the pattern.
In conventional CAD construction, however, elements are created by the user directly interacting with the pattern geometry. The user drafts the desired geometric elements using mouse movements and clicks. Elements are constructed based on manual calculations undertaken by the user to apply the measurements to the pattern using the rules and instructions of the construction method. For example, in the Aldrich method, the skirt block width from the back centre to the front centre is constructed based on this manual calculation ‘half of the hip circumference + 1.5 as fixed ease’. These manual calculations are time-consuming, and the traditional approach does not allow their embedding as associated elements. Parameter specifications are not used in this construction, and no complex geometric or spatial relationships between the pattern elements are developed because elements are connected by the constructor. This means that each element in the pattern is practically independent from the others even if there are actual dependencies between them. As a result, if elements are changed or removed, the others around them are not affected. This functionality makes it possible to create patterns quickly but has the disadvantage that significant changes to the pattern will mean much time being spent in carrying out numerous other resulting modifications.
Unlike the conventional approach, parametric construction relies on specifying points needed to build up the block, and the software automatically connects them to each other. These points are not specified directly. They are, instead, specified indirectly through their coordinates (locations) using tools in the software. In this paper, three methods were used to specify points in the construction space which were: an absolute Cartesian method, an absolute Polar method and a relative Polar method.
It is important to note that the investigation of these methods is based on existing multidisciplinary literature (McFarland, Citation2008;Onstott, Citation2017). Previous scholarly works in pattern construction have not specifically focused on examining these methods within this domain. Exploring this aspect is significant as it provides a unique perspective on pattern development and introduces a different approach to thinking about patterns, representing a crucial transitional step. Notably, Seamly2D offers tools such as a point at distance and angle, and intersection point XY from 2 points, which demonstrate the practical application of these coordinate systems. Consequently, this investigation contributes to bridging a research gap in the pattern construction literature by providing fresh insights and understanding into the concept, role, and impacts of coordinate systems in the development of parametric patterns.
The absolute coordinate system of the Cartesian method defines coordinates in a grid of perpendicular lines (Volkwyn, Airey, Gregorcic, & Linder, Citation2020; Snow et al., Citation2022). In the horizontal x-axis, positive values denote movement to the right and negative values movement to the left, whilst positive values on the vertical y-axis denote movement up and negative values movement down. The point where these two axes intersect is the origin, start or datum point. The coordinates of this point are X 0, Y 0 and it is used as the absolute reference for identifying points on the XY system with a pair of equations for each point. The X coordinate is the first equation, and the Y coordinate is the second equation ().
This method was used to specify most coordinates. For example, to locate the centre front point at the hip level (A8) in the trouser block, a vertical line intersecting the X axis was constructed using this pair of equations (X = Hips/4+ Fr hip ease, Y = -waist to hip). The horizontal distance between the origin point and the intersection point is called the X coordinate of A8, specifying how far A8 is to the right from the origin along the X axis. The vertical distance between the origin and the intersection point is called the Y coordinate of A8, specifying how far the point is below the origin along the -Y axis. The intersection of these horizontal and vertical lines is the coordinate of A8, whose X coordinate is positive because it is measured from the right of the origin, while its Y coordinate is negative because it is measured down from the origin point. Points on patterns can have origin points that are individual to them and each point can become an origin for another point. This network of points is what creates the pattern outline.
The second method of specifying coordinates is the absolute Polar method. In this method, a coordinate is identified by distance and angle (direction) from the origin point (Snow et al., Citation2022, p. 150); (Hahn, Citation2020, p. 128). The distance equals the length of line to be constructed, and the angle is formed by the height of the vertical line intersecting the identified point with the x-axis. See .
Seamly2D defines right (toward three o’clock) as the default direction of the 0° angle. All other directions are determined from a counterclockwise rotation. In the construction space, up is 90°, left is 180°, and down is 270°. See .
The absolute Polar coordinates method allows creating oblique lines from the origin point. For example, it was used in the skirt block to connect the back centre at waist level (A1) to the back side seam uplift (A10).
Regarding the relative Polar coordinates method, this relies on a distance and angle relative to the last point (Frey & McFarland, Citation2007, p. 32). This method allows creating oblique lines from a coordinate developed in reference to the origin point as shown in .
An example of using this method is identifying the coordinate of back shoulder point (A11) in the bodice back block based on the back neck point (A9) identified in reference to the origin point (A0).
In all these methods, coordinates of points were specified using a point-numbering method based on the calculations of Aldrich method like the traditional CAD construction, but these calculations were undertaken automatically by the software. This requires creating a dynamic table containing all variables (measurements, ease, and additions) needed to construct the parametric block. shows the variables needed to construct an Aldrich parametric skirt as an example.
Table 3. The variables table of an Aldrich parametric skirt.
Creating this dynamic table requires breaking down the calculation of construction, which is time consuming, but it highlights all variables impacting the garment style and fit and makes them controllable. Thus, they can be investigated, complicated more or fed by other variables. After creating this table, its variables are used to formulate the calculations needed to build the block, known in parametric construction as parametric equations, and the software calculates them automatically.
What is special in specifying coordinates in the parametric construction is that each point is linked technically to its inputs – properties such as its length equation, angle, and dependency. These inputs stay technically connected to the block outputs via this properties editor. Setting these properties could take time, but they are dynamic, editable, and reusable. Thus, once they are established, any changes in the properties will be easy and time-saving compared to the conventional method. Also, specifying points in the construction space and connecting them with parameters ensures more precision and accuracy. Parametric construction reduces the requirements of experience (Yang et al., Citation2007) compared to conventional direct construction, which requires a significant level of knowledge and practical experience to get precise and accurate outputs.
5.1.3. Construction workflow
The construction workflow refers to the various steps, choices, and rules required to carry out a determined task (Allen, Citation2006). In traditional construction, the workflow is not dynamic. All the pattern elements are static and independent. This makes the traditional workflow equivalent to the manual one that captures only the geometric shape of the pattern but not its logic. Neither does it provide reusability of the pattern geometry as parameterisation does. illustrates the traditional CAD construction workflow.
Such undynamic workflow means that in traditional construction restricts the capacity to experiment with the construction process and limits adjustments that may be made later.
In contrast, the parametric construction workflow captures the pattern’s geometric shape as well as its logic and provides reusability of the pattern's geometry to modify it, resize it or reuse it to generate unlimited styles. illustrates the parametric construction workflow.
This dynamic workflow facilitates the decomposition of patterns into rules and provides the flexibility to easily make changes and apply them to the pattern. It allows the pattern constructor to think through the body-to-pattern relationship and explore the entire pattern within the identified parameters. This, in turn, enables testing of novel alternatives and solutions for fit issues in a CAD environment without time-consuming reworkings and adjustments, ultimately promoting creative outcomes better than what is possible in the traditional approach. Previous studies have also highlighted the positive relationship between design creativity and the parametric approach, as argued by Hesselgren, Charitou and Dritsas (Citation2007), Jabi et al. (Citation2017), Iordanova et al. (Citation2023) and Yu, Gu, and Ostwald (Citation2018)
5.2. Construction outputs
The pattern outputs of parametric and the traditional CAD approaches are similar because blocks are constructed using the same measurements and rules of the Aldrich method. All blocks are 2D composed of a set of points, straight lines and curves. However, in the traditional construction, the pattern outputs are static similar to the manual ones as shown in .
Although the traditional approach and its rendering tools allow block construction more quickly than the parametric approach, once they are created, these static blocks cannot be resised entirely. They can only be graded at fixed points using the traditional grading system. The traditional blocks require recreation from scratch for each new wearer or to test a solution. Also, making any significant changes to any one of them requires adjusting all impacted elements manually. This is a time-consuming process.
On the contrary, the pattern outputs of parameterisation are dynamic, geometrically associative, and responsive to input-properties. shows the pattern outputs of the parametric approach.
Figure 15. Blocks outputs of parametric approach, numbering identifies points controllable by the points’ editor.
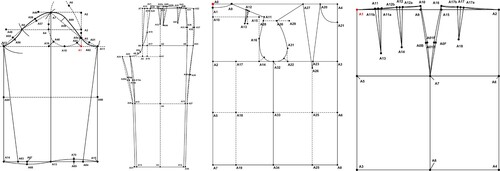
As shown in the figure, these parametric blocks have numbers where each element of the pattern is coded as a discrete point linked to input data. The body-to-pattern relationship exists and is coded and stored into these numbers called parameters. In the context of pattern construction, parameters are spatial and geometric variables that serve to characterise the various properties of the pattern including its shape, size, orientation, and location of its individual components (Kondolf & Piégay, Citation2016). These parameters do not implement changes, as these are executed by the pattern constructor, but rather they modify the pattern as a result of the constructor's changes.
By establishing this connectivity between the block inputs and outputs, the parametric approach effectively replaces the direct interaction between the constructor and the block geometry with the development of a series of relationships by which the block's geometric elements automatically connect with each other to build up the block. This allows engineering of the block by specifying where each point is located on the block surface and describing how it relates spatially to other elements. This approach is essential to understanding how a block is built from the start point to the endpoint and the relationships between the block’s constituent parts. This helps the constructor identify where the body-to-pattern relationships exist. Therefore, they can customise the block according to individual inputs, and when there is a fit issue, the constructor would be able to determine its potential causes, the block elements that are affected by this issue, and the areas where a solution might be developed. This is done in a deeper and more dynamic way than possible with the traditional approach.
Thus, once the initial block has been rigged and coded, it no longer needs to be recreated for each new wearer or for testing a fit proposal. All that is required is to change the values in the variables table or change the construction rules in the properties editor, and the changes will be reflected instantly in the block, either visually through its dimensions or in the related databases such as the variables table and the properties editor of the altered parameters. As a result, the time saved on the recreation of a block is considerable, and a foundation for improving its fit, resising, customising, or manipulating it to different styles is provided.
Facilitating the development of customised patterns for individual customers has the potential to promote sustainability by curbing the wastage and overproduction inherent in mass production models. By producing clothing items that are tailored to fit the precise measurements of each customer, the amount of fabric used in the production process can be reduced, resulting in greater material efficiency and less fabric waste.
However, it should be borne in mind that in comparison to traditional CAD outputs, parametric outputs demand a greater initial investment of time and effort to identify, create, and encode the relationships between the blocks’ constituents and their input data. However, as blocks are often used for long periods of time, having this flexibility brings greater opportunities for adjusting for individual size, shape or changed style requirements.
While coding a block, parameterisation allows visualisation. The lines and curves used to construct the block can be formatted in different styles, such as broken or continuous, weight, thick or thin, and colours, in accordance with their intended uses. For example, structural lines which are constructed as a base to other lines but do not form a part of the block output are dotted in the pattern construction. Visualising pattern construction explains the block theory and allows pattern constructors to visualise their ideas. Visualising a block could also include visualising the body dimensions and ease allowance, in other words the variance between the dimension of the body and the measurements of the block, added for comfort and mobility purposes (Zhang et al., 2015; Jay 1969 cited in Gill & Chadwick, Citation2009); it could also include the removal of dependencies in the draft process so that the front and the back can be drafted separately as seen in .
Visualising the block and body dimensions assists the constructor in making decisions about the body-to-pattern relationship, and the removal of dependencies between the front and back blocks in the construction process is the first step to break the traditional restraints in pattern construction.
However, the outputs of parametric and traditional approaches are the same geometrically. This is a limitation of parameterisation caused by adhering to the rules of the Aldrich method when parameterising the blocks. Adhering to the rules of existing pattern construction methods results in blocks having fit issues because they are unsuitable for bespoke garments in which a block should be constructed for an individual body’s specifications in terms of shape and measurements. Lim and Cassidy (Citation2017) have confirmed this unsuitability after testing the suitability of four popularly used pattern construction methods, including Aldrich used in this comparison, for bespoke trousers. Lim and Cassidy (Citation2017) constructed trouser blocks using the four selected methods using the same subject’s anthropometric measurements. Then, the block’s outputs were used to fabricate the experimental trousers which then were evaluated by experts while being worn by the subject in different positions such as standing straight, stepping at walking pace, sitting 90˚, stooping 90˚, climbing the stairs and squatting on hams. The outcomes of this evaluation showed that existing methods generate unsatisfactory appearance and fit. This is because they do not consider the ethnic differences in the body characteristics, and their proportional rules are fixed while subjects vary proportionally.
Alrushaydan et al. (Citation2020) confirmed these outcomes after constructing a trouser pattern using 18 different methods commonly used in pattern constructions for industrial and academic practices including those used in Lim and Cassidy (Citation2017). The block outcomes were then compared digitally to one another in relation to the body by superimposing them upon each other. The outcomes comparison showed that existing methods produce different blocks in terms of shape and measurement although they were constructed for the same sized body-form. The differences found in Alrushaydan et al. (Citation2020) included the amount of side seam uplift shaping a part of the trouser and skirt blocks. This poor fitting suggests that none of existing methods are underpinned by pattern cutting theory or developed based on a suitable depth of understanding of the body-to-pattern relationship.
Lim and Cassidy (Citation2017) and Alrushaydan et al. (Citation2020) focused mainly on evaluating the fit of garments constructed using existing pattern construction methods, which often rely on fixed proportional rules and do not account for individual variations in body shape and proportions. However, one of the limitations of this research is that it does not offer much insight into why the garments did not fit well or how the pattern construction methods can be improved.
Visualising the pattern, as discussed above, can help address this limitation by providing a more comprehensive understanding of the body-to-pattern relationship and how it affects the final garment fit. The parametric approach allows pattern constructors to manipulate the lines and curves of the block in different styles and colours, which can help them better visualise how changes in the pattern affect the final garment. This visualisation can help pattern constructors identify areas of the pattern that need adjustment, such as adding or removing ease, adjusting the angle of darts, or altering seam placement. By doing so, pattern constructors can create more customised patterns that result in better-fitting garments.
Furthermore, visualising the pattern can also help pattern constructors identify issues related to the dynamic properties of the body, such as how the garment moves and stretches with the body during different activities. By imagining garment movement and identifying areas of strain or discomfort, pattern constructors can create patterns that not only fit well but also enhance comfort, mobility, and performance.
6. Conclusions
The process of producing a custom-fit garment typically involves constructing a customised block and making numerous iterative modifications to customise the block. In the traditional approach, any modifications in the geometric inputs or outputs are applied manually to all modified elements of the pattern. This makes the traditional construction method time-consuming, costly, relies heavily on the skill and experience of the pattern constructor and is ultimately unsuitable for individually customised blocks generation. The parametric approach overcomes these limitations by providing connectivity between the block inputs and outputs. This connectivity provides a speedy, time-saving and accurate mechanism to arrive at the body’s key dimensions needed to customise a pattern. This connectivity makes the block inputs editable and its outputs adjustable. This provides the pattern constructor with control over the block geometric elements and opportunity of exploration and testing in CAD and makes any modification a less-time consuming process and reducing the experience requirements needed in the traditional construction. The parametric approach also makes more explicit the body to pattern relationship and helps to bridge the gap between pattern construction and engineering required to harness CAD developments and support better pattern outcomes.
7. Limitations
To provide accurate representation and guidance, it's important to acknowledge the study's limitations. These include a limited sample size (four basic blocks), potentially limiting applicability to more complex patterns. The study did not evaluate the fit of traditional and parametric approaches on real bodies, focusing solely on comparing construction processes. Additionally, the comparison used specific software (Seamly2D and Lectra) with their own unique limitations, potentially yielding different results with other software.
8. Recommendations
This paper suggests using open-source parametric pattern construction technologies in educational institutions to teach efficient and accurate construction methods. Researchers can leverage the parametric approach to develop novel construction methods for the bespoke garment industry, benefiting from speed, accuracy, and time-saving advantages. Parameterisation enables the development of dynamic pattern systems for fit experiments and facilitates improving the relationship between the body and the pattern. Furthermore, improving the efficiency of existing open-source software with a 2D-to-3D algorithm and parametric human form can enhance the customisation and fit of blocks. Future research should include comprehensive evaluations of fit on real bodies to further enhance understanding and applicability of these construction approaches.
Disclosure statement
No potential conflict of interest was reported by the author(s).
References
- Aldrich, W. (2015). Metric pattern cutting for women’s wear (6th ed). Chichester, UK: Wiley.
- Allen, K. S. (2006). Programming windows workflow foundation: Practical WF techniques and examples using XAML and C#. Birmingham, UK: Packt Publishing.
- Almond, K., & Power, J. (2018). Breaking the rules in pattern cutting: An interdisciplinary approach to promote creativity in pedagogy. Art, Design and Communication in Higher Education, 17(1), 33–50. doi:10.1386/adch.17.1.33_1.
- Alrushaydan, T., et al. (2020). Enhancing pattern construction by body scanning: the importance of curves. 11th Int. Conference and Exhibition on 3D Body Scanning and Processing Technologies, [Preprint].
- Anderson, R. (2009). Bespoke: Savile row ripped and smoothed. London, UK: Simon & Schuster.
- Andriasyan, M., Moyano, J., Nieto-Julián, J. E., & Antón, D. (2020). From point cloud data to building information modelling: An automatic parametric workflow for heritage. Remote Sensing, 12(7), 1094. doi:10.3390/rs12071094.
- Bao, C., et al. (2021). 3D interactive garment parametric pattern-making and linkage editing based on constrained contour lines. International Journal of Clothing Science and Technology, 33(5), 696–723.
- Baran, R. J., & Galka, R. J. (2016). Customer relationship management: The foundation of contemporary marketing strategy. New York: Taylor & Francis.
- Caetano, I., Santos, L., & Leitão, A. (2020). Computational design in architecture: Defining parametric, generative, and algorithmic design. Frontiers of Architectural Research, 9(2), 287–300.
- Casini, M. (2022). Construction 4.0 advanced technology, tools and materials for the digital transformation of the construction industry. Woodhead Publishing.
- Daanen, H., & Hong, S. (2008). Made-to-measure pattern development based on 3D whole body scans. International Journal of Clothing Science and Technology, 20(1), 15–25. doi:10.1108/09556220810843502.
- Daneshgar, F. (2023). A practical guide to qualitative research: A guide for research students, supervisors, and academic authors. Bloomington, Indiana, United States: Xlibris AU.
- Datta, D., & Seal, P. (2018). Various approaches in pattern making for garment sector. Journal of Textile Engineering & Fashion Technology, 4, 36899621.
- Frey, D., & McFarland, J. (2007). AutoCAD 2008 and AutoCAD LT 2008. New Jersey: John Wiley & Sons.
- Gill, S., & Chadwick, N. (2009). Determination of ease allowances included in pattern construction methods. International Journal of Fashion Design, Technology and Education, 2, 23–31. doi:10.1080/17543260903018990.
- Gindis, E., & Kaebisch, R. C. (2019). Up and running with AutoCAD 2020. Amsterdam: Academic Press.
- Gu, N., Yu, R., & Behbahani, P. A. (2018). Parametric design: Theoretical development and algorithmic foundation for design generation in architecture. In B. Sriraman (Ed.), Handbook of the mathematics of the arts and sciences (pp. 1–22). Cham: Springer International Publishing. doi:10.1007/978-3-319-70658-0_8-1.
- Hahn, A. J. (2020). Basic calculus of planetary orbits and interplanetary flight, basic calculus of planetary orbits and interplanetary flight. New York: Springer.
- Hesselgren, L., Charitou, R., & Dritsas, S. (2007). The bishopsgate tower case study. International Journal of Architectural Computing, 5(1), 61–81. doi:10.1260/147807707780912912.
- Huang, H. Q., et al. (2012). Block pattern generation: From parameterizing human bodies to fit feature-aligned and Flattenable 3D garments. Computers in Industry, 63(7), 680–691.
- Iordanova, I., et al. (2023). Parametric methods of exploration and creativity during architectural design: A case study in the design studio. T. Tidafi and T. Dorta (Eds.), Joining languages, cultures and visions: CAADFutures 2009, PUM, 2009, pp. 423–439. [Preprint].
- Istook, C. L. (2002). Enabling mass customization: Computer-driven alteration methods. International Journal of Clothing Science and Technology, 14(1), 61–76.
- Jabi, W., et al. (2017). Enhancing parametric design through non-manifold topology. Design Studies, 52, 96–114.
- Jhanji, Y. (2018). 11 – Computer-aided design – Garment designing and patternmaking. In R. Nayak, & R. B. T.-A. Padhye (Eds.), The textile institute book series (pp. 253–290). Sawston, Cambridge: Woodhead Publishing.
- Jin, P., Fan, J., Zheng, R., Chen, Q., Liu, L., Jiang, R.-T., & Zhang, H. (2023). Design and research of automatic garment-pattern-generation system based on parameterized design. Sustainability, 15(2), 1268–1268. doi:10.3390/su15021268.
- Kang, Y., & Kim, S. (2019). Three-dimensional garment pattern design using progressive mesh cutting algorithm. International Journal of Clothing Science and Technology, 31(3), 339–349.
- Kondolf, G. M., & Piégay, H. (2016). Tools in fluvial geomorphology. John Wiley & Sons, Ltd.
- Leach, G. (2018). Forensic psychology. London: EDTECH.
- Lim, H., & Istook, C. (2012). Automatic pattern generation process for made-to-measure. Journal of Textile and Apparel, Technology and Management, 7.
- Lim, H. W., & Cassidy, T. (2017). A comparative study of trouser pattern making methods. Journal of Textile Engineering & Fashion Technology, 1(5), 00031.
- Liu, W., Xie, H., & Song, Y. (2017). Study on the method of generating paper pattern by clothing fit customization. Journal of Business Administration Research, 6(2), 22.
- McFarland, J. (2008). AutoCAD 2009 and AutoCAD LT 2009 : No experience required. Hoboken: John Wiley & Sons.
- Moniruzzaman, M., & Rita, A. (2022). An approach to design solutions for garments using a CAD system. Journal of Textile Engineering & Fashion Technology, 8, 145–148.
- Moore, J. (2020). Patternmaking history and theory. New York: Bloomsbury Visual Arts PP - London.
- Mwenisongole, T. A., & Mligo, E. S. (2018). Pastoral counseling for orphans and vulnerable children: A narrative approach. Eugene: Wipf and Stock Publishers.
- Onstott, S. (2017). AutoCAD 2018 and AutoCAD LT 2018 essentials. Indianapolis: John Wiley & Sons.
- Publishers, H. C. (2016). Collins cobuild advanced dictionary of English. Jakarta: Gramedia Pustaka Utama.
- Snow, R. E., et al. (2022). Aptitude, learning and instruction. Oxfordshire: Taylor & Francis.
- Stephen Samuel, A. P. G. B. (2006). Basic and intermediate NX4 modeling, drafting and assemblies. San Jose: Design Visionaries.
- Touloupaki, E., & Theodosiou, T. (2017). Performance simulation integrated in parametric 3D modeling as a method for early stage design optimization-A review. Energies, 10(5), 637.
- Volkwyn, T. S., Airey, J., Gregorcic, B., & Linder, C. (2020). Developing representational competence: Linking real-world motion to physics concepts through graphs. Learning: Research and Practice, 6(1), 88–107. doi:10.1080/23735082.2020.1750670.
- Xiu, Y., Wan, Z.-K., & Cao, W. (2011). A constructive approach toward a parametric pattern-making model. Textile Research Journal, 81(10), 979–991.
- Xu, Y., Thomassey, S., & Zeng, X. (2020). Garment mass customization methods for the cutting-related processes. Textile Research Journal, 91(7–8).
- Yan, J., & Kuzmichev, V. E. (2020). A virtual e-bespoke men’s shirt based on new body measurements and method of pattern drafting. Textile Research Journal, 90(19-20), 2223–2244.
- Yang, Y., Zhang, W., & Shan, C. (2007). Investigating the development of digital patterns for customized apparel. International Journal of Clothing Science and Technology, 19(3), 167–177.
- Yu, R., Gu, N., & Ostwald, M. (2018). Evaluating creativity in parametric design environments and geometric modelling environments. Architectural Science Review, 61(6), 443–453. doi:10.1080/00038628.2018.1512043.
- Zhang, J., Innami, N., Kim, K., & Takatera, M. (2015). Upper garment 3D modeling for pattern making. International Journal of Clothing Science and Technology, 27(6), 852–869.
- Zieman, N. L. (2009). Pattern fitting with confidence. Cincinnati: Kp Craft Books.