ABSTRACT
To fully analyze the forestry and wood products sector's impact on climate protection through the reduction of CO2 emissions, it is necessary to integrate all aspects of both carbon storage and the substitution effects of forestry and wood use. Substitution effects are assessed based on specific assumptions derived from life-cycle assessment studies, which for the purpose of comparison usually refer to the respective energy mix. This definition is, however, normative. Different assumptions regarding fossil fuel substitution, such as marginal benefit or changing energy mix, are also possible. This paper discusses which of these alternative assumptions are most appropriate for analyzing fossil fuel substitution and then, based on three different approaches (status quo, changing energy mix, marginal fossil), shows the variation in the results these assumptions provide. The calculations include three different forest management strategies in order to show the effects of forest C sinks and different wood volumes available for use (material and energy). Long-term simulations tracking the C effects of forestry and wood use reveal the greatest variations. These findings are useful for deciding between different forestry management scenarios and strategies for wood product and energy use from a climate protection perspective.
1. Introduction
A sustainable forestry and wood products sector provides a positive contribution to climate protection by reducing atmospheric carbon. Forests act as carbon sinks by absorbing CO2 through photosynthesis and tree growth (forest carbon stock). When a tree is harvested, the absorbed C is not immediately released as CO2. While quite a substantial amount of the biomass is left on site to decompose with the time, the larger portion of the biomass is used for energy production or stored in long-lived (wood) products [Citation1,Citation2]. Wood use can also replace fossil fuels such as oil, gas or coal since during processing and disposal wood products typically require less energy than other competing products (material substitution) [Citation3–6], and wood can be used as an alternative to fossil fuels (fuel substitution) [Citation7–9].
Increased forest carbon stock levels are recognized as a measure for reducing CO2 emissions in the context of climate reporting (see Art. 3.4. of the Kyoto Protocol) [Citation10–13]; the effects of harvested wood product (HWP) pools are expected to be addressed in a follow-up agreement to the Kyoto Protocol [Citation14,Citation15]. The IPCC Good Practice Guidance for Land Use, Land Use Change and Forestry provides an accepted basis for assessing carbon storage in forests and HWPs. Today the substitution effects, on the other hand, are not recognized as reducing CO2 emissions in climate reporting directly, but since they reduce the use of fossil fuels [Citation16] the effect is accounted in the energy sector. The substitution effects are not evaluated using universally accepted calculation standards, but are based on reasonable and plausible assumptions in individual studies [Citation5,Citation17]. These studies use so-called substitution factors [Citation6]. For example, the determination of CO2 or C effects of material substitution is based on the regional energy mix [Citation5,Citation17] using life-cycle assessment (LCA) software, such as GaBi (Ganzheitliche Bilanzierung); GEMIS (Globales Emissions-Modell integrierter Systeme) or the Ecoinvent database. In most cases, energy substitution is assessed based on the assumption of a marginal fossil fuel-based technology (e.g., “marginal technologies used for the production of heat;” for details see section “Developing different assessment approaches”). This makes it possible to determine the average fossil energy mix, as in [Citation17] based on the Swedish Energy Agency 2012, or a fossil energy mix which takes into account the use of wood and its substitution in specific combustion technologies (such as household wood-burning stoves) as in [Citation18] based on [Citation19]. Rüter [Citation20] chooses the combustion of light oil as the marginal fuel, which, compared with the approach selected by BMU [Citation19], given today's energy use, leads to the same results. It makes sense to use different approaches for assessing the material and energy substitution (average energy mix versus marginal technology). A more general analysis, including more than 100 countries [Citation16], shows that biomass does not substitute for fossil fuels in a 1:1 ratio, and [Citation21] shows the deficiencies of the carbon neutrality approach. A quite comprehensive study with regard to the influence of various baseline scenarios on the CO2-effects of wood fuel was given by Johnson and Tschudi [Citation22]. They tested for the baseline definitions no baseline, reference point, marginal fossil fuel and biomass opportunity cost. The results show extreme variations in the carbon footprint of wood energy. Focused on the aim of the current study, to compare the substitution of a given fossil fuel mix and for a given region/situation, it is possible to assess material as well as fuel substitution based on a marginal fossil fuel-based technology. The quoted studies show a large variety in their assessment approaches; they refer to the average fossil energy mix or a specific fossil energy mix or a particular fossil fuel such as heating oil. This approach is more specific than general approaches which study fossil fuel substitution with non-fossil fuels, as described by [Citation16].
Since determining the most appropriate method for assessing the current C effects of wood use is already subject to debate, deciding the best approach for determining future effects is less clear. As numerous studies assess the C effects of forestry and wood use in the future and often for long periods of time, from 50 to 100 years [Citation5,Citation23–26], the question arises as to which approaches are most appropriate for assessing substitution effects in the future, and which results the different approaches provide. The following assessment uses a simulation of the C effects generated in the forests and through timber harvesting wood product use in the German state of North Rhine Westphalia (NRW). The approach and the methods are described in detail by [Citation18,Citation27]. This assessment incorporates conclusions drawn from discussions on carbon neutrality approach (e.g., [Citation28]; see above) and follows a holistic approach, which takes into account the Cstock decrease, for example by harvesting. The aim of this paper is to show which results the different assessment approaches provide: a status quo approach based on existing studies [Citation5,Citation17,Citation18] (see above); an approach that assumes an energy mix with a lower CO2 output; an approach using energy production from lignite as a marginal technology. NRW is a major producer of thermal energy and electricity from lignite. As a CO2-reduction measure, the idea of reducing the burning of lignite in the years to come is currently heavily debated in the political arena.
Material and methods
Modeling of C effects of the forestry and wood use in the German state of North Rhine Westphalia
The state of NRW has a forest cover of 915,000 ha dominated by spruce and beech stands. On average 6.0 million m3 of wood is harvested annually [Citation29]. The modeling used three scenarios for sustainable forest management simulation procedures for the period 2010 to 2100: volume strategy for high wood production, value strategy for high timber value and carbon storage strategy for high forest carbon stocks through limited timber harvesting. A detailed description of the scenarios can be found in [Citation18]. These three forest management scenarios represent extremes. Their goal is not to create a realistic forecast of future developments, but to derive different options for action on the basis of defined scenarios (for the differences between scenario analyses and prognoses see [Citation30]). Generally, the modeling considers the annual growth of biomass (C flux in) and wood harvesting (C flux out) for the above- and belowground biomass and the development of the deadwood (forest residues from harvesting which remain in the forest are considered to contribute to the carbon stock but eventually decompose). The approach is in line with arguments of [Citation21–25,Citation31] to consider C-stock changes in the substitution assessment.
Forest growth was modeled based on data from the two German National Forest Inventories (1986–1989 and 2001–2002) [Citation32], harvest statistics from the State Forestry Administration and yield tables for the main tree species [e.g., Citation33]. The modeling only used verifiable data, which excluded such information as changes in site conditions due to climate change as well as natural disasters like storms and heavy snowfall. In addition, certain simplifications were made: for example, it was assumed that the mix of tree species remains unchanged; that is, it did not take into account a shift to more hardwoods or fast-growing softwoods (such as Douglas fir). The carbon budget was calculated in accordance with IPCC Good Practice Guidance for Land Use, Land Use Change and Forestry [Citation12,Citation13], whereby the carbon stored in the soil and litter was considered constant over time (the default method). The annual change in forest carbon stock was determined for the three analysis scenarios; in addition, each model shows the amount of wood to be harvested each year. A model was developed to describe wood use. For this purpose, the study used national statistics on material flows in the timber industry and the energy sector [e.g., Citation34–38], and regional data (such as sales information from the State Forestry Administration) to develop a tree species-specific utilization model and a regional material flow model tracking the process from harvested wood to end products and energy, respectively. The model was used for the harvested wood calculated in the three forest management scenarios. In accordance with [Citation14,Citation15], the finished products were divided into product groups with long life spans (most timber products), medium life spans (such as wood-based panels) and short life spans (such as paper), and fuel wood. For the purpose of simplification, it was accepted that the wood products were used the same way whether they were used domestically or exported. Changes in the wood product stock were calculated based on [Citation15]. The substitution effects were assessed at the time of occurrence – that is, for material substitution at the time of production and for fuel substitution at the time of combustion.
Calculation of material and fuel substitution
The modeling of the replacement of fossil energy by bioenergy (i.e., coal, oil, gas by biomass) has been the subject of extensive discussion. In early stages, biofuels, especially wood, were considered “C neutral” as a result of a simplified interpretation of the Kyoto Protocol. Today, it is generally understood to be much more complex and the need is now clear for considering the individual situation of the biofuel resource and the process/use chain of the biofuel [Citation16,Citation39]. Bird et al. [Citation39], for example, discuss combustion factors (CoF) between 0 and 1. Sathre and O'Connor [Citation6] give an overview for the calculation of substitution factors and the results for material substitution of wood products. The substitution factors are used to calculate the C effects of the substitution of other fuels (energy substitution) or materials for consumer products (material substitution) for a given amount of biomass or wood, respectively.
In this work, the substitution factor for material substitution SFMa is calculated according to [Citation5,Citation6] based on Equation (1). It compares the CO2 emissions inherent in the production and disposal of wood products with that of non-wood products with the same functionality. While [Citation6] takes into account that non-wood products may contain a small amount of wood, [Citation5] and Equation (1) do not.(1)
The substitution factor for fuel substitution SFFuel is calculated in a similar way and is defined in Equation (2). In this case, the CO2 emissions of different energy sources (wood versus non-wood) are compared; the functional unit is the unit of generated energy (electricity or heat).(2)
The unit used for SF is tCO2/tC or tC/tC. Instead of the mass of carbon, some studies refer to the mass or the volume of wood (kg, m3) [Citation5]. For the sake of conformity with carbon studies in the forestry sector [Citation40] or with inventory studies as part of international greenhouse gas reporting [Citation41,Citation42], according to Equations (1) and (2), the carbon content of the wood product can be used as reference, so tC/tC is used, using appropriate conversion factors [Citation12].
The substitution factors are calculated based on comparative LCA or from comparisons using Environmental Product Declarations (EPD), such as IBU (Institut Bauen und Umwelt e.V.), Germany [Citation43], LCA studies [Citation5,Citation6] or LCA databases.
Calculation of material substitution factors
The substitution factors for material substitution were taken from [Citation18] considering 16 wood product groups representing wood use in Germany. Energy consumption figures for the product groups and their competing products were taken from the literature – that is, LCA studies [Citation5,Citation6] and EPD publications [Citation43]. Taking the volume mix of the 16 product groups in the market [Citation34], an average substitution factor of SFMa = 1.50 tC/tC was calculated. A detailed description of the methods used and the calculation is given in [Citation18,Citation44]. Although the substitution factor is based on overall wood use in Germany, the situation of wood use in NRW specifically does not deviate significantly.
Calculation of fuel substitution factors
The substitution factor was determined based on the fossil fuel mix according to [Citation19]. The amounts of fossil fuel consumed in early stages of the production chain (such as fossil fuel consumed in forest management, timber harvesting, fuel wood processing or transport) are considered up to a maximum of 10% of the lower heating value depending on the specific product (pellets, wood chips, fire wood, etc.) [Citation45]. The modeling considers the change in forest carbon fluxes and forest carbon pools (see section “Calculation of material and fuel substitution”), the processes of fuel wood preparation as well as the efficiency factors for wood energy generation. Using this approach, the substitution factor for energy substitution SFFuel is determined as 0.67 = tC/tC. The approach is both conceptually and quantitatively in line with recommendations in the literature [e.g., in Citation16,Citation20,Citation23,Citation25,Citation28,Citation46].
Developing different assessment approaches
The analysis of material substitution on the basis of a current overall energy mix and the evaluation of fuel substitution on the basis of fossil fuel use (a specific energy source or mix of different fossil fuels) are considered the status quo approach (Approach A) for assessing substitution through wood use. A particular feature of this approach is that an analysis based on an energy mix today is not changed throughout the observation period. The following presents two additional assessment approaches which represent extremes: Approach B refers to the use of the fossil fuel (throughout the observation period) with the highest CO2 emission (marginal fossil approach), and Approach C refers to an energy mix reflecting the politically motivated reduction of CO2 emissions (changing energy mix approach).
Approach B (marginal fossil approach): Lignite is the main energy source in the study region NRW, responsible for 47% of the region's overall generated electricity [Citation47]. The CO2 emissions from lignite are significantly higher than those of other fossil fuels due to lignite's chemical composition and low calorific value. Compared to natural gas, the emissions from lignite are 2 to 3 times higher depending on the combustion technology used and the origin of the lignite [Citation48]. Plans for the use of lignite as an energy source in NRW extend to until the year 2045 [Citation49]. But the exit schedule remains unset; the current level of lignite use in NRW justifies assessing its use for power and heat generation as a margin technology for the present as well as in the future. This approach is referred to as margin fossil and assumes lignite is the energy source for both material and fuel substitution. The simulation is based on a conversion factor to Approach A (status quo approach): a substitution factor of SFMa_margin = SFMa × 2 = 3.0 tC/tC is applied for material substitution, and SFFuel_margin = SFFuel × 1.3 = 0.87 tC/tC for fuel substitution. The conversion factors were determined based on an assessment by [Citation48,Citation50] and are within the range described in the literature [Citation28,Citation39].
Approach C (changing energy mix approach): This approach takes into account the political goal to reduce CO2 emissions in the future [Citation51,Citation52]. The approach is therefore referred to as the changing energy mix approach. According to the NRW Climate Protection Law [Citation53] and the NRW Climate Protection Plan [Citation54], CO2 emissions are to be reduced 80% by 2050 (25% by 2020) based on 1990. The NRW Climate Protection Law makes no statements in relation to the period after 2050. Therefore, it is assumed that between 2050 and 2100, CO2 emissions will decline linearly down to zero (which is certainly a theoretical point). Based on these policy guidelines, and using the status quo approach, the substitution factors for fuel are predicted to develop up to the year 2100 as follows: 2010 SFFuel 2010 = 0.67 tC/tC; 2020 SFFuel 2020 = 0.58 tC/tC; 2050 SFFuel 2050 = 0.16 tC/tC; 2100 SFFuel 2100 = 0.00 tC/tC. Values between the years given are linearly interpolated. The current substitution factor for material substitution of SFMa 2010 = 1.5 tC/tC is adapted for the period 2011–2100 relative to the change in substitution factors for fuel (SFMa 2010 = 1.5 tC/tC; SFMa 2020 = 1.3 tC/tC; SFMa 2050 = 0.36 tC/tC; SFMa 2100 = 0.0 tC/tC).
Results
The three assessment approaches for substitution (status quo, marginal fossil, changing energy mix) were analyzed for each of the three forest management scenarios. The following first presents and discusses the results for the less extreme value strategy forest scenario combined with the three substitution approaches then compares all three forest management scenarios together with the three substitution approaches and finally discusses the consequences for forest management (section “Discussion”).
Three energy substitution approaches combined with the value strategy scenario for forest management
shows the annual C effects for the period 2011 to 2100 for the status quo approach, the C effects for the marginal fossil approach and for the changing energy mix approach. The figures distinguish between the annual change in the forest carbon stock, the wood product stock and the substitution effects of wood use (emission reduction through fuel and material substitution). Because the calculations provided in this report only refer to substitution effects, the forest carbon stock and wood product stock are the same in each of the three figures. Based on international convention, negative values represent a reduction in CO2 emissions. After a period of increasing forest carbon stock levels (C sink), from 2058 onwards the carbon stock levels decline, and the forest becomes a C source until 2097 when it reverts back to a C sink again. The reason for this change and re-change is the age structure of the forest in consideration, since in Germany as a whole and other European countries after World War II large forest areas were harvested and replanted mainly with spruce. About 100 years after re-planting (approx. 2050–2060), the forests will reach maturity and will be harvested. The HWP carbon pool increases until 2092 (C sink), then becomes a C source because of the declining wood harvesting volumes compared to the decades before. The substitution effects are positive (negative values) for the entire period 2011–2100.
Figure 1. C effects (annual) of the value strategy scenario's C effect (2011 to 2100): Forest and harvested wood product (HWP) C stock, fuel and material substitution, total C effect – status quo approach for fossil fuel substitution.
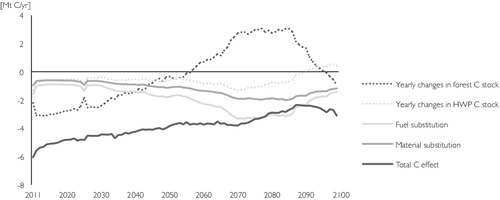
Figure 2. C effects (annual) of the value strategy scenario (2011 to 2100): Forest and harvested wood product (HWP) C stock, fuel and material substitution, total C effect – marginal fossil approach for fossil fuel substitution.
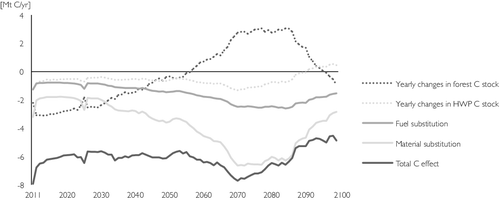
Figure 3. C effects (annual) of the value strategy scenario's C effect (2011 to 2100): Forest and harvested wood product (HWP) C stock, fuel and material substitution, total C effect – changing energy mix approach for fossil fuel substitution.
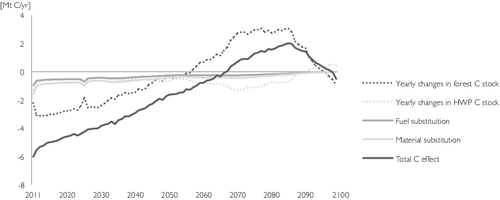
(status quo assumption) shows the highest annual substitution effects (for both material and energy together) with an average of approx. − 5.1 million tC per year between 2070 and 2087 (some 5.6 t C/ha,yr). This is the period with the highest amount of harvested and processed wood. Thus, in this period the effects of material substitution are highest. Second highest are the effects of fuel substitution through increased use of fuel wood from the forest and the use of processing by-products which are predominantly used for energy. The depletion of the forest carbon stock in the period after 2058 is balanced by the higher effects of substitution. Overall, the C effects from 2011 to 2088 decline from − 6.0 million tC per year to approximately − 2.3 million tC per year, and only start to increase at the end of the observation period to − 3.1 million tC per year due to the increased forest carbon stock. That means that the accumulated C effects of the forestry, wood products and wood energy sectors remain positive over the whole period despite the lower forest carbon sink. This shows that wood use can have a higher effect on C emission reduction compared to forest C stock.
In (marginal fossil approach), the substitution effects are, according to the methodological assumptions, 1.3 to 2.0 times higher compared to the status quo approach in . This results in higher absolute C effects. Substitution is highest in the period of increased stock depletion between 2070 and 2090, and the year 2071 shows the highest reduction levels for a single year with − 7.7 million tC per year.
In (changing energy mix), the substitution effects recorded for the year 2011 correspond to those recorded in (status quo) and decline almost linearly to zero by the year 2100. Thus, the substitution effects for any year after 2011 are lower than those shown in . In the year 2050, by definition (see methods), only 23% of the values from 2011 are reported (i.e., 20% relative to the base year 1990). After 2050, the total effect is largely determined by the development of the forest carbon stock and to a lesser extent by the harvested wood product pool. Overall, a positive value (C source) is shown for the period 2068–2097. After 2100 (not shown in the figure), the total effect is solely determined by the forest and harvested wood product carbon pool; substitution, as per definition, no longer contributes to CO2 reduction.
A comparison of the three approaches is shown in . The accumulated yearly values from show the overall development of forest carbon stock (dotted line) and the sum of all C effects of forestry and wood use (HWP pool, material and energy substitution) for the period 2011–2100. It distinguishes among the three approaches for fossil fuel substitution (status quo, marginal fossil, changing energy mix). therefore compiles and summarizes the information of . As for –, the negative values indicate emission reduction. Negative values in – correspond with a positive slope in the graphs in . The dotted line in representing the forest carbon stock shows negative values for the entire period, although the changes are remarkable between 2011 and 2058, and 2058 and 2097, respectively. This shows for the entire observation period that the forest carbon stock is greater than at the starting year, 2011. For the status quo assumption, the overall C effect for forestry and wood use together is − 346 million tC. For the marginal fossil assumption, it is some 60% higher with − 551 million tC. The lowest effect is with the changing energy mix approach, which shows only − 133 million tC. Between 2069 and 2098, the forest and wood use sector becomes a CO2 emitter (see also ). This is dominated by the development of the forest carbon stock. Extrapolated into infinity, it converges with the combined curve for the forest and wood product stock combined, as it can already be recognized from 2050 onwards.
Three forest management scenarios combined with the three substitution approaches
The previous section showed that the findings derived from the forest management scenario value strategy vary depending on the assumptions for substitution approaches. In principle, the findings can also be applied to the other two forest management scenarios, the volume strategy and carbon storage strategy. The following presents and analyzes with aggregated data the results for the three forest management scenarios and the three different approaches for substitution. shows the cumulative development of the carbon stock between 2011 and 2100 for all forest management scenarios for the status quo approach. The development of the forest carbon stock is shown with dotted lines; the sum of all C effects for the combined system forest and wood use as a solid line. shows a different trend for forest carbon stock resulting from different forest management options. However, when including the carbon effect of wood use, the three forest management scenarios differ considerably less in their overall C effect. Lower harvest volumes with the carbon storage strategy result in higher forest (carbon) stocks but lower substitution effects. The volume strategy scenario provides high harvest volumes resulting in lower increase of forest (carbon) stock but high substitution effects. The C effects of the three scenarios are similar for the period until about 2040, after which the curve for the carbon storage strategy flattens reflecting a lower C effect. The curve flattens as well for the value strategy scenario after about 2065. This means that from 2040 on the highest C effects are generated by forest management scenarios promoting more wood use.
Figure 5. C effects (cumulative) for all forest management scenarios (2011 to 2100): forest C stock (dotted line), sum of all C effects for the combined system forest and wood use (forest and harvested wood product [HWP] C stock, fuel and material substitution; solid lines) – fossil fuel substitution: status quo.
![Figure 5. C effects (cumulative) for all forest management scenarios (2011 to 2100): forest C stock (dotted line), sum of all C effects for the combined system forest and wood use (forest and harvested wood product [HWP] C stock, fuel and material substitution; solid lines) – fossil fuel substitution: status quo.](/cms/asset/b14f472c-9829-452f-a708-72f84e832b89/tcmt_a_1166427_f0005_b.gif)
shows, similar to , the cumulated C effects of the three forest management scenarios combined with the substitution approaches marginal fossil and changing energy mix. For marginal fossil, using lignite as a reference, increased wood use (volume strategy) generates significantly higher C effects (almost doubled) than the carbon storage strategy which uses less wood. For the marginal fossil approach, the benefits are highest for all three forest management scenarios during the entire period. For the changing energy mix approach the three forest management scenarios are indifferent with regard to C effects until about 2035, and then largely follow the trend of the forest carbon stock as shown in . In general, the effects are the lowest of all substitution approaches.
Figure 6. C effects (cumulative) for all forest management scenarios (2011 to 2100): forest C stock (dotted line), sum of all C effects for the combined system forest and wood use (forest and harvested wood product [HWP] C stock, fuel and material substitution; solid lines) – fossil fuel substitution: changing energy mix and marginal fossil.
![Figure 6. C effects (cumulative) for all forest management scenarios (2011 to 2100): forest C stock (dotted line), sum of all C effects for the combined system forest and wood use (forest and harvested wood product [HWP] C stock, fuel and material substitution; solid lines) – fossil fuel substitution: changing energy mix and marginal fossil.](/cms/asset/78137382-94b9-45bf-acda-07a30db44925/tcmt_a_1166427_f0006_b.gif)
Discussion
The calculated CO2 or C effects largely depend on which underlying approaches are used to assess the material and fuel substitution. This leads to different recommendations as to which forest management strategy is best or most appropriate from the perspective of climate protection. If policy sets targets to replace fossil fuel with biomass (among other substitution strategies), wood use for energy will increase and this has an influence on forest management strategies. The marginal fossil approach supports a forest management strategy promoting higher wood use (volume strategy). The status quo approach supports the volume strategy as well, but the effect is high only after the year 2060. No generally applicable recommendations for forestry management can be derived from the changing energy mix approach, as carbon stock effects dominate over substitution effects with time until 2100. shows a matrix of the main results and the recommendations drawn for the three forest management scenarios and the three approaches used to assess the substitution effects.
Table 1. Forest management scenarios rated against approaches used to analyze substitution effects (+ positive effect to +++ very positive effect).
The following discusses which approaches are most appropriate to assess the effects of substitution. For this assessment, the criterion of maximum marginal benefit is used. Instead of referring to monetary figures (e.g., CO2 mitigation costs), the economic concept of marginal benefit refers to the benefits in terms of carbon emission reductions. The marginal benefit explains how much atmospheric CO2 is reduced when an additional unit of wood (e.g., 1 t or 1 m3) is used. A marginal benefit analysis justifies why it is appropriate, when using wood for substitution, to refer to those fuel sources that will actually be replaced with wood or have been replaced in the past. In the context of climate change legislation in Germany and the state of NRW, renewable or CO2-free energy sources are being promoted to replace fossil fuel. This may result in an increased use of wood as a renewable energy source. An overall energy mix is less suitable as a basis for assessment because it also already contains renewable sources of energy, which are not intended for substitution (this point does not take into consideration that, for economic and technological reasons, renewable energy sources might replace other renewable energy sources to a limited extent). This finding speaks against the changing energy mix approach in which wood also substitutes for other renewable sources of energy. It is much more appropriate to analyze the substitution of specific fossil fuels only. Which fossil fuels are best suitable as a basis for calculation depends among other things on the energy legislation, the regional energy mix or, finally, the specifics of wood use in a region (e.g., combustion technologies such as household energy – firewood – or biomass-fired combined heat and power plants). For long-term predictions, it is necessary to make assumptions on how such parameters will change in the future. Thus, ecological balance considerations in terms of a consequential life-cycle assessment (cLCA) [Citation55–Citation58] are methodologically appropriate. Current material substitution assessments usually follow an attributional approach to life-cycle assessment (aLCA). Assuming that the fuel type producing a region's highest CO2 emissions per unit of energy is substituted, then for NRW with a high consumption of lignite, the appropriate approach is the marginal fossil approach using lignite as the substituted fuel. The substitution of lignite with wood is controversially discussed in Germany. The German Energy Agency, dena, has determined the co-firing of wood in coal-fired power plants to be highly economical (compared to other renewable energy sources): “A higher level of co-firing tends to reduce CO2 mitigation costs” [Citation59]. That means that the replacement of coal with wood (in NRW specifically, the replacement of lignite) is justified from an economic viewpoint as well as in the context of CO2 emission reduction. This argumentation might be rational according to the stated goal of the most-related policies. On the other hand, it is not self-evident that the effects of those policies will be realized.
The status quo approach is the most appropriate from a conformity and practicality perspective. It offers a compromise between the other two more extreme approaches. Against the backdrop of an uncertain future (Which energy sources will be used in the future and to what extent?), the status quo approach makes sense “as a baseline” which likely will gain the broadest acceptance. But the drawback is that any changes, such as energy policy measures, cannot be evaluated in depth.
If in the distant future (i.e., after 2100) fossil fuels were to be completely replaced by CO2 emission-free energy sources, no substitution effects are to be achieved by wood use or bioenergy or other renewable energy sources, respectively. Wood use would only influence changes in the forest carbon stock and HWP stock. Wood use would continue to make sense for reasons of climate protection because, on one hand, reduced wood use results in a reduced HWP carbon pool and thus results in net CO2 emissions. On the other hand, with increasing tree age the forest would experience a biological equilibrium (equal levels of biomass accumulation and decay) and gradually lose its C-sink function. HWPs become relatively more important, and HWP carbon pools are not subject to intensive decay or oxidation if design and use of wood products favor long-term service lives – that is, timber in buildings. In the case of a CO2-neutral economy, the contribution of the forestry and wood product use to the reduction of CO2 emissions requires new strategies with a focus on combined forest and HWP C pools. Strategies to further optimize harvesting volumes and bring almost all harvested wood into (long life) wood product pools are obvious.
Moreover, in a CO2-neutral economy it would be worth discussing removing wood from the forests and storing it over very long periods, for example in deep mines [Citation60–63]. These considerations, however, ignore the socio-economic effects of wood use and in general the use of renewable resources. This also contradicts the concept of sustainability [Citation64]. Against this background it is useful to discuss how to maximize the forest and wood product storage function, for example through strategic forest management and the use of long-life wooden products.
Acknowledgements
This research was part of the study "Beitrag des NRW Clusters ForstHolz zum Klimaschutz” (NRW cluster ForstHolz's contribution to climate change mitigation [Citation27]). The study was conducted on behalf of the Ministry for Climate Protection, Environment, Agriculture, Nature Conservation and Consumer Protection (MKULNV) of the German State of North Rhine-Westphalia, and the Landesbetrieb Wald und Holz NRW (North Rhine-Westphalia Agency for Forestry and Timber Management). We thank all participants from the State Forest Administration of North Rhine-Westphalia. For scientific co-operation, we are thankful to Michael Köhl, Volker Mues and Konstantin Olschofsky from the Department of World-Forestry, University of Hamburg, Germany, who carried out the forest management modeling/scenario analysis.
Disclosure statement
The authors declare no conflict of interest.
References
- Perez-Garcia J, Lippke B, Comnick J. An assessment of carbon pools, storage, and wood products market substitution using life-cycle analysis results. Wood and Fiber Sci. 37(CORRIM Special Issue), 140–148 (2005).
- Skog KE. Sequestration of carbon in harvested wood products for the United States. Forest Prod. J. 56(6), 56–72 (2008).
- Frühwald A, Solberg B. LCA – a challenge for forestry and forest products industry [Internet]. In: EFI Proceedings No. 8. Frühwald A, Solberg B (Eds.). Joensuu (1995). Available from: http://www.efi.int/files/attachments/publications/proc08_net.pdf.
- Lippke B, Wilson J, Perez-Garcia J, Bowyer J, Meil J. CORRIM: Life-cycle environmental performance of renewable building materials. Forest Prod. J. 54(6), 8–19 (2004).
- Taverna R, Hofer P, Werner F, Kaufmann E, Thürig E. The CO2 Effects of the Swiss Forestry and Timber Industry. Scenarios of future potential for climate-change mitigation. Bern (2007).
- Sathre R, O'Connor J. A Synthesis of Research on Wood Products and Greenhouse Gas Impacts, 2nd Edition [Internet]. FPInnovations, Vancouver (2010). Available from: https://www.canfor.com/docs/why-wood/tr19-complete-pub-web.pdf.
- Reijnders L. Conditions for the sustainability of biomass based fuel use. Energ. Policy. 34(7), 863–876 (2006).
- Gustavsson L, Holmberg J, Dornburg V et al. Using biomass for climate change mitigation and oil use reduction. Energ. Policy. 35(11), 5671–5691 (2007).
- Sathre R, Gustavsson L. A state-of-the-art review of energy and climate effects of wood product substitution. School of Technology and Design Reports 57, University Växjö, Växjö, Sweden (2009).
- UNFCCC. Report of the Conference of the Parties on its seventh Session, held at Marrakesh from 29 October to 10 November 2001. FCCC/CP/2001/13/Add.1 [Internet]. Marrakesh (2002). Available from: http://unfccc.int/resource/docs/cop7/13a01.pdf.
- IPCC. IPCC Guidelines for National Greenhouse Gas Inventories Volume 4 Agriculture, Forestry and Other Land Use [Internet]. Hayama, (2006). Available from: http://www.ipcc-nggip.iges.or.jp/public/2006gl/vol4.html.
- IPCC. Good practice guidance for land use, land-use change and forestry. Institute for Global Environmental Strategies (IGES) [Internet]. Hayama (2003). Available from: http://www.ipcc-nggip.iges.or.jp/public/gpglulucf/gpglulucf_files/GPG_LULUCF_FULL.pdf.
- IPCC. IPCC guidelines for national greenhouse gas inventories; reference manual. Institute for Global Environmental Strategies (IGES). Hayama (2006).
- UNFCCC. Synthesis report of the technical assessments of the forest management reference level submissions. Note by the secretariat, Durban (2011).
- IPCC. Revised Supplementary Methods and Good Practice Guidance Arising from the Kyoto Protocol. Hayama, (2013).
- York R. Do alternative energy sources displace fossil fuels? Nature Clim. Change. 2(6), 441–443 (2012).
- Lundmark T, Bergh J, Hofer P et al. Potential roles of swedish forestry in the context of climate change mitigation. Forests. 5(4), 557–578 (2014).
- Knauf M, Köhl M, Mues V, Olschofsky K, Frühwald A. Modeling the CO2-effects of forest management and wood usage on a regional basis. Carbon Balance and Management. 10(1), 13 (2015).
- BMU (Bundesministerium für Umwelt, Naturschutz und Reaktorsicherheit). Erneuerbare Energien in Zahlen. Nationale und internationale Entwicklung. Berlin, Juli 2012.
- Rüter S. Welchen Beitrag leisten Holzprodukte zur CO2-Bilanz? AFZ der Wald. 15, 15–18 (2011).
- Zanchi G, Pena N, Bird N. Is woody bioenergy carbon neutral? A comparative assessment of emissions from consumption of woody bioenergy and fossil fuel. GCB Bioenergy. 4(6), 761–772 (2012).
- Johnson E, Tschudi D. Baseline effects on carbon footprints of biofuels: The case of wood. Environ. Impact Assess. Rev. 37 IS, 12–17 (2012).
- Holtsmark B. Harvesting in boreal forests and the biofuel carbon debt. Clim. Change. 112(2), 415–428 (2012).
- Holtsmark B. The outcome is in the assumptions: analyzing the effects on atmospheric CO2 levels of increased use of bioenergy from forest biomass. GCB Bioenergy. 5(4), 467–473 (2013).
- Schulze E-D, Körner C, Law BE, Haberl H, Luyssaert S. Large-scale bioenergy from additional harvest of forest biomass is neither sustainable nor greenhouse gas neutral. GCB Bioenergy. 4(6), 611–616 (2012).
- Körner C. Slow in, Rapid out–Carbon Flux Studies and Kyoto Targets. Science. 300(5623), 1242–1243 (2003).
- Knauf M, Frühwald A. Beitrag des NRW Clusters ForstHolz zum Klimaschutz. Langfassung der Studie [Internet]. Landesbetrieb Wald und Holz Nordrhein-Westfalen, Münster (2013). Available from: https://www.wald-und-holz.nrw.de/fileadmin/Publikationen/Broschueren/Broschuere_Studie_Klimaschutz_Langfassung.pdf..
- Haberl H. Net land-atmosphere flows of biogenic carbon related to bioenergy: towards an understanding of systemic feedbacks. GCB Bioenergy. 5(4), 351–357 (2013).
- MKULNV (Ministerium für Klimaschutz Umwelt Landwirtschaft Natur- und Verbraucherschutz des Landes Nordrhein-Westfalen). Landeswaldbericht 2012 [Internet]. Düsseldorf (2012). Available from: https://www.wald-und-holz.nrw.de/fileadmin/Wald-und-Holz/Dokumente/Landeswaldbericht_2012.pdf.
- Lindgren M, Bandhold H. Scenario planning – the link between future and strategy. Palgrave Macmillan, Basingstoke (2002).
- Körner C. Biologische Kohlenstoffsenken: Umsatz und Kapital nicht verwechseln! [Biological Carbon Sinks: Turnover Must Not Be Confused with Capital!]. Gaia. 18(4), 288–293 (2009).
- Schmitz F, Polley H, Hennig P, Dunger K, Schwitzgebel F. Die zweite Bundeswaldinventur – BWI2 Inventur- und Auswertungsmethoden; zu den Bundeswaldinventuren 2001 bis 2002 und 1986 bis 1988. Johann Heinrich von Thünen Institut (vTI). Hamburg (2008).
- Jüttner O. Ertragstafeln für Eichen. Ertragstafeln wichtiger Baumarten bei verschiedener Durchforstung. 2nd edition. Sauerländer, Frankfurt (1975).
- Mantau U, Bilitewski B. Stoffstrom-Modell-Holz. Bestimmung des Aufkommens, der Verwendung und des Verbleibs von Holzprodukten. Forschungsbericht für den Verband Deutscher Papierfabriken e. V. (VDP), Celle (2010).
- Seintsch B. Entwicklungen des Clusters Forst und Holz: Studie “Volkswirtschaftliche Bedeutung des Clusters Forst und Holz“ im Rahmen der “Bundesweiten Clusterstudie Forst und Holz”. Holz-Zentralblatt. 134(49), 1390–1391 (2008).
- Weimar H. Der Holzfluss in der Bundesrepublik Deutschland 2009. Methode und Ergebnis der Modellierung des Stoffflusses von Holz [Internet]. Institut für Ökonomie der Forst- und Holzwirtschaft (Johann Heinrich von Thünen-Institut), Hamburg (2011). Available from: http://literatur.vti.bund.de/digbib_extern/bitv/dn049777.pdf
- Mantau U. Holzrohstoffbilanz Deutschland, Entwicklungen und Szenarien des Holzaufkommens und der Holzverwendung 1987 bis 2015 [Internet]. Hamburg (2012). Available from: http://literatur.vti.bund.de/digbib_extern/dn051281.pdf.
- Dieter M. Holzbilanzen 2005 und 2006 für die Bundesrepublik Deutschland. Institut für Ökonomie der Bundesforschungsanstalt für Forst- und Holzwirtschaft, Arbeitsbericht, Hamburg (2007).
- Bird DN, Pena N, Frieden D, Zanchi G. Zero, one, or in between: evaluation of alternative national and entity-level accounting for bioenergy. GCB Bioenergy. 4(5), 576–587 (2012).
- Wördehoff R, Spellmann H, Evers J, Nagel J. Kohlenstoffstudie Forst und Holz Niedersachsen. Beiträge aus der Nordwestdeutschen Forstlichen Versuchsanstalt Band 6, Göttingen (2011).
- Oehmichen K, Demant B, Dunger K et al. Inventurstudie 2008 und Treibhausgasinventar Wald [Internet]. Landbauforschung vTI Agriculture and Forestry Research, Braunschweig (2011). Available from: http://literatur.vti.bund.de/digbib_extern/dn048141.pdf.
- Umweltbundesamt. Berichterstattung unter der Klimarahmenkonvention der Vereinten Nationen und dem Kyoto-Protokoll 2013. Nationaler Inventarbericht zum Deutschen Treibhausgasinventar 1990 – 2011 [Internet]. Dessau-Roßlau (2013). Available from: http://www.umweltbundesamt.de/sites/default/files/medien/461/publikationen/climate_change_08_2013_nir_2013_gniffke.pdf.
- IBU (Institut Bauen und Umwelt e.V.). Database: Environmental Product Declarations. [Internet]. Berlin (2014). Available from: http://bau-umwelt.de.
- Frühwald A, Knauf M. Carbon aspects promote building with wood. Proceedings of the World Conference on Timber Engineering (WCTE), Quebec (2014).
- Frühwald A, Wegener G, Krüger S, Beudert M. Forst- und Holzwirtschaft unter dem Aspekt der CO2-Problematik. Forschungsbericht. Forstabsatzfonds Bonn (1994).
- Haberl H, Sprinz D, Bonazountas M et al. Correcting a fundamental error in greenhouse gas accounting related to bioenergy. Energ. Policy. 45(0), 18–23 (2012).
- IT NRW (Information und Technik Nordrhein-Westfalen Geschäftsbereich Statistik). Energiebilanz und CO2-Bilanz in Nordrhein-Westfalen 2011. Jan 2014 [Internet]. Düsseldorf (2014). Available from: https://webshop.it.nrw.de/gratis/E449%20201100.pdf.
- Wagner H-J, Koch MK, Burkhardt J, Bockmann TG, Feck N, Kruse P. CO2-Emissionen der Stromerzeugung – ein ganzheitlicher Vergleich verschiedener Techniken. 59(10), 44–52 (2007).
- Erkelenz. Umsiedlungen Garzweiler II [Internet]. Erkelenz (2014). Available from: http://www.erkelenz.de/de/bauen/garzweiler/.
- Icha P. Entwicklung der spezifischen Kohlendioxid-Emissionen des deutschen Strommix in den Jahren 1990 bis 2012. Umweltbundesamt (UBA), Dessau-Roßlau (2013).
- European Commission. Energy 2020 – a strategy for competitive, sustainable and secure energy. Publications Office of the European Union, Brussels. (2010).
- European Parliament and the Council. Directive 2009/28/EC of the European Parliament and of the Council of 23 April 2009 on the promotion of the use of energy from renewable sources and amending and subsequently repealing Directives 2001/77/EC and 2003/30/EC. Brussels. (2009).
- Klimaschutzgesetz NRW. Gesetz zur Förderung des Klimaschutzes in Nordrhein-Westfalen [Internet]. Düsseldorf (2013). Available from: https://recht.nrw.de/lmi/owa/br_bes_text?anw_nr=2&gld_nr=7&ugl_nr=7129&bes_id=22784&aufgehoben=N&menu=1&sg=0.
- Klimaschutzplan NRW. Der Klimaschutzplan [Internet]. Düsseldorf (2014). Available from: http://www.klimaschutz.nrw.de.
- Weidema BP. Market information in life cycle assessment. Environmental Project No. 863. Danish Environmental Protection Agency, Copenhagen (2003).
- Earles JM, Halog A. Consequential life cycle assessment: a review. Int J Life Cycle Assess. 16(5), 445–453 (2011).
- Earles JM, Halog A, Ince P, Skog K. Integrated economic equilibrium and life cycle assessment modeling for policy-based consequential LCA. J. Ind. Ecol. 17(3), 375–384 (2013).
- Zamagni A, Guinée J, Heijungs R, Masoni P, Raggi A. Lights and shadows in consequential LCA. Int J Life Cycle Assess. 17(7), 904–918 (2012).
- Vogel C, Herr M, Edel M, Seidl H. Die Mitverbrennung holzartiger Biomasse in Kohlekraftwerken. Ein Beitrag zur Energiewende und zum Klimaschutz? Deutsche Energie-Agentur GmbH (dena), Berlin (2011).
- Scholz F, Hasse U. Permanent wood sequestration: The solution to the global carbon dioxide problem. ChemSusChem. 1(5), 381–384 (2008).
- Zeng N, King A, Zaitchik B et al. Carbon sequestration via wood harvest and storage: An assessment of its harvest potential. Clim. Change. 118(2), 245–257 (2013).
- Zeng N. Carbon sequestration via wood burial. Carbon Balance Manage. 3(1), 1 (2008).
- Köhl M, Frühwald A. Permanent wood sequestration: No solution to the global carbon dioxide problem. ChemSusChem. 2(7), 609–613 (2009).
- Forest Europe. State of Europe's forests 2011: status and trends in sustainable forest management in Europe. United Nations Economic Commission for Europe (UNECE) and the Food and Agriculture Organization of the United Nations (FAO), Oslo (2011).