Abstract
Addition of organic amendments (OAs) could be a means to sequester carbon (C) in soils. However, the efficiency of C sequestration depends on how OAs evolve in the soil. A field study was installed in arid soil to which was added one of five OAs – olive husk-based compost (CM), palm leaf-based compost (CP), crushed olive pruning (GW), fresh olive mill wastewater (OMW) or fermented ovine manure (OM) – at an equivalent fixed level of 350 g of C/m2. C and N mineralization were followed for 112 days by measuring the evolution of released CO2 and mineral N evolution. The results showed that CM and CP did not disturb soil respiration, with a very low CO2 emission or almost no respiration for the CM, while CO2 release reached 7.6 g of CO2/m2 for GW and OMW. Soil organic carbon (SOC) was sustainably improved by 0.54% and 0.50%, respectively, for CM and CP. N mineralization showed no significant difference between amended and untreated soils. Based on these results, compost amendment was the most efficient for C sequestration to enhance soil fertility and consequently reduce the rate of CO2 emission.
Introduction
Soils can represent a sink for atmospheric greenhouse gases (GHGs) such as CO2, N2O and CH4. Exchanges of carbon (C) between soil and atmosphere are very important and can be positive (sequestration) or negative (CO2 emission), related with soil management (Lal Citation2010). C sequestration in the terrestrial ecosystem mostly occurs by photosynthesis (Voronin Citation2006). The atmospheric C fixed by plants returns into the soil by natural incorporation of the plant residues (Paustian et al. Citation2000) or as organic amendment added by farmers directly or after transformation. The use of food industry organic wastes as organic amendment (OA), either fresh or through composting, is becoming a common practice (Flavel and Murphy Citation2006). Agricultural activities can reduce loss of C and enhance its storage in the soil (Hutchinson et al. Citation2007). Among the C conserving cultural practices, the most important is the application of no-tillage farming (Baker et al. Citation2007; Guo et al. Citation2016), cover crops (Lal Citation2004), agroforestry practices (Lal Citation2005) and OA (Ryals et al. Citation2014). Conversely, as a consequence of the intensity, farmlands that are intensively handled generate huge amounts of GHGs (Hutchinson et al. Citation2007), as well as changes in inland use that comprise deforestation and biomass burning, as well as the transformation of natural to agricultural ecosystems (Lal Citation2004).
Application of OAs is considered the most efficient strategy for the valorization of organic residues (Flavel and Murphy Citation2006; Thangarajan et al. Citation2013) by handling agricultural organic wastes such as crop wastes, agricultural by-products such as ovine manure, pig manure, olive mill wastewater (OMW), olive husk and green manure, and by reducing the problems caused by their accumulation such as their direct incorporation into the soil or after composting (Ferreras et al. Citation2006). Moreover, this alternative increases C sequestration in the soil. Cabrera et al. (Citation2009) and Powlson et al. (Citation2012) proposed that OA led to increased C storage in soils (Ryals et al. Citation2014). In addition, OA enhances soil fertility by improving soil physical and chemical properties, C content, and microbial activity (Bronick and Lalan Citation2005; Thangarajan et al. Citation2013). Consequently, plant growth and fruit yield are improved (Flavel and Murphy Citation2006).
Evolution of organic matter in the soil follows two paths, direct mineralization and humification (Lehmann et al. Citation2007). Labile organic C that contains easily degraded compounds such as sugars, proteins and carboxylates is directly mineralized (Fischer et al. Citation2010). This mineralization produces CO2 and thus C loss due to microbial C decomposition (Bot and Benites Citation2005). Mineralization is a complex phenomenon that is linked to environmental conditions and to the properties of the organic matter. Mineralization is enhanced when external and intrinsic properties are suitable to develop microorganism activity (Xie et al. Citation2017). Indeed, soil temperature, weather temperature and humidity are key factors for microorganisms’ life and, therefore, high weather humidity and temperature enhance soil organic matter (SOM) degradation (Stone et al. Citation2014). Moreover, the higher the content of easily degraded components in the organic matter is, the higher its direct mineralization should be (Zech et al. Citation1997, Martín et al. Citation2012). N mineral availability in the soil and low immobilization of N-NO3− reduce C storage in the soil, and increase organic C mineralization and therefore greenhouse gas emissions (Eduardo et al. Citation2016).
The other path followed by the residual part of organic C after direct mineralization is humification. This process is achieved through successive decomposition and modification of dead organic matter that is converted to humic compounds or humus. These compounds are composed of more complex organic substances after biochemical processing. Humification results in stabilization of the organic matter achieved by its transformation into stable humic compounds including humic and fulvic acids and humine. Humic acids (10,000 and 100,000 Da) are complex aromatic macromolecules enriched with aliphatic compounds that bind to peptides and amino sugars (Stevenson Citation1982). Fulvic acids (1000 to 30,000 Da) contain aromatic and aliphatic structures strongly enriched by functional groups (Buffle Citation1988). Humine, with the highest molecular weight, is considered the most stable compound that is insoluble regardless of the pH (Tan Citation1994; Bot and Benites Citation2005).
However, humification is not the only method of organic matter stabilization. There are several mechanisms of stabilization of SOM, as described by Six et al. (Citation2004). Physical protection is a mechanism of SOM stabilization, which is an occlusion of the organic matter within macro- and micro-aggregates or small pores. However, only the degradation of biochemically labile materials is delayed by physical protection mechanisms. Although the recalcitrant materials decompose slowly anyway, they are hardly affected by physical protection (Krull et al. (Citation2003). SOM can also be stabilized by organo-mineral complexes through chemical protection and its interaction with mineral surfaces or with other organic molecules. These mechanisms are important to decrease the bioavailability and accessibility of SOM for soil microorganisms and soil enzymes (Sollins et al., Citation1996; Kelleher and Simpson, Citation2006; von Lützow et al., Citation2006; Bachmann et al., Citation2008). The selective preservation of certain recalcitrant organic compounds is also considered an important method of stabilization. Decomposition of organic compounds may be restricted due to their molecular-level characteristics such as elemental composition, presence of functional groups, and molecular conformation (Marschner et al. Citation2008).
Consequently, mineralization of exogenous organic matter (EOM) can be subdivided into primary mineralization that affects fresh litter, and secondary mineralization that represents a progressive degradation of stabilized organic matter (Guggenberger Citation2005). The C stored in soil is higher when the amount of stabilized SOM is increased. Consequently, reduction of atmospheric CO2 and soil fertility conservation and improvement are enhanced when C sequestration in soils is improved. Thus, SOM stabilization should be enhanced versus mineralization. For this reason, more efficient organic matters are those containing more humic substances considered stable components, or more compounds suitable for the stabilization process such as lignin and the phenolic compounds that participate in humus formation (Nicolas et al. Citation2012). Additionally, environmental conditions affect the humification rate. Many studies have focused on organic C evolution and dynamic modelling of the organic C introduced (Al-Adamat et al. Citation2007; Falloon and Smith Citation2002; Saffih-Hdadi and Mary Citation2008).
Hence, different models were developed to pursue decomposition and stabilization of the EOM input: RothC-26.3, Century, Daisy, GEFSOC, AMG, etc. (RampazzoTodorovic et al. Citation2014). These models are important to anticipate variations in soil organic carbon (SOC) content under various environmental conditions and management practices. Moreover, many of these situations could improve understanding of the stabilization of C in soil (Ludwig et al. Citation2007).
However, to the best of the authors’ knowledge, no study has investigated the effects of characteristics of intrinsic organic matter on their evolution and their environmental impacts in arid soils, although it has been proposed that soils in semiarid and arid conditions with low C content have a large potential for C sequestration (Lal Citation2004). However, to the best of the authors’ knowledge, this has not been proven independently from organic amendment composition, nor has whether this potential for sequestration is important with all types of organic amendments. The aim of this study was to understand, based on the characteristics of exogenous organic matter, what path SOM evolution would follow and the capacity of arid soils to sequester C. The impact of soil C balance and C gain after EOM application were determined. Moreover, the mechanisms behind the observed impacts were assessed. Five organic amendments at different stages of maturity were used in field experiments. Soil respiration was followed, in addition to determining the part of stable C obtained, and the C balance and C gain or loss.
Materials and methods
Experimental study
A field study was undertaken in an arid climate from the end of February 2016 to the beginning of June 2016. This period was chosen because it is characterized by climatic conditions suitable for soil microbial activity, such as temperature between 20 °C and 30 °C and rainfall in the southern Mediterranean region. During this period, the highest and the lowest temperatures recorded were 35 °C and 8 °C, respectively. Daily soil temperature average was approximately between 17 and 18 during the first week and reached 28 at the end of the experiment. Rainfall was almost absent, reaching only 26.5 mm during the experimentation period of 112 days, much below the normal rainfall average (65 mm). Water was then added to allow microbial development. For this reason, experimental plots were irrigated weekly with 10 L/m2 of water if it was not raining. Rainfall was 11 mm in April and 7 mm in May. Therefore, no irrigation was applied in these periods. Consequently, 130 mm total irrigation was added during the experiment. The total amount of water received during the whole experimental period was 148 mm.
The experimental field was non-cultivated arable land located in Sfax in central Tunisia (34°43’47”N, 10°44’12”E). The soil was classified as a sandy loam with 37.80% sand, 62.12% silt and 0.07% clay. It is an alkaline soil with a pH of 8.9, high electrical conductivity (EC = 15.09 mS/cm) and classified as saline soil according to the Food and Agriculture Organization of the United Nations (FAO) (Mathieu and Pietain Citation2003). This soil had a low amount of organic carbon, 0.6% equivalent to 600 g /m2 soil and 0.025% of total N.
The field trial was installed based on a block design with six treatments and three replicates. Five EOMs were used. The supplied quantity of each EOM was calculated in order to achieve a supply of 350 g/m2 of organic C or almost 0.35% of carbon added depending on EOM intrinsic C content. This amount of organic C corresponds to 3.5 T of C/ha equivalent to 10 T/ha at 10 cm depth of sheep manure, which is the common organic fertilizer used by farmers.
The organic amendments applied were manure, plant residues, manure/waste from fresh food industry, and manure/composts of plant residues including manure and olive husk (CM), a palm leaf-based compost (CP), olive pruning residues (GW), fresh OMW and fermented ovine manure (OM).
To apply 350 g/m2 of organic C, the amounts of the different amendments applied were:
Ovine manure: 1 kg/m-2
Olive mill wastewater: 9 L/m2
Olive husk-based compost: 1.28 kg/m2
Olive pruning residues: 0.79 kg/m2
Palm-based compost: 1.17 kg/m2
The physical and chemical characteristics of these amendments are shown in .
Table 1. Physical and chemical characteristics of the five organic amendments.
The amendments were incorporated into the superficial soil layer (0–10 cm) and were thoroughly mixed with soil by hand to ensure homogeneity. In addition to these treatments, a control plot without EOM addition was included. For all the plots other than OMW, the same amount of water as that brought by OMW addition was included.
During the first week, soil activity was important. For this reason, samples were taken daily during this period, then weekly until the end of the first month and every 14 days after that when soil activity seemed less important. The survey was followed until stabilization of respiration, 112 days after EOM addition.
For each plot, the soil sample was composed of three samples from three sampling points mixed together. Soil samples were taken from the upper layer corresponding to a depth of 0–20 cm.
Physical and chemical analyses of soil samples
Soil pH was measured in a soil–water mixture with a ratio of 1:2.5 (10 g of soil was mixed with 25 mL of water) using a glass electrode and a Neomet pH meter. The soil EC was determined using a conductimeter (inoLab) to measure the liquid extract of soil-saturated paste (Pauwels et al., Citation1992). Soil organic C was obtained using the Heanes method, which is a modified Walkley and Black method with external heating to help oxidize the total organic matter supplement to the heat from the addition of sulfuric acid to a potassium dichromate solution (Conyers et al., Citation2011). The total N content was determined by the Kjeldhal method (Pauwels et al. Citation1992). To determine mineral N content in soil, fresh soil samples were stirred with 50 mL of 1 N KCl, and after filtration, N-NH4+ (ammonium) was oxidized by MgO to be transformed into gaseous NH3 that was extracted by vapour distillation and caught in boric acid, and then the amount of N was evaluated by titration with 0.01 N hydrochloric acid (HCl). Then, N-NO3− (nitrate) N was transformed into NH3 by reduction of aluminum alloy and caught in boric acid after vapour distillation, and the amount of N was determined by titration with 0.01 N HCl (Pauwels et al. Citation1992).
Soil respiration from the soil surface was measured in the field by the alkaline absorption method for a surface covered by sealed cylinders 15 cm high and 10 cm in diameter inserted to a depth of 2–3 cm, with three replicates for each plot. The CO2 released from soil was measured for 24 hours before the sampling dates and determined by catching the CO2 produced in 0.1 N NaOH, and the excess of NaOH was determined in laboratory by titration in the presence of phenolphthalein (Annabi Citation2009). Soil respiration was measured daily during the first week and then weekly, and the values obtained were expressed in mg/m2.
Calculations and statistical analyses
To evaluate C mineralization induced by the different EOMs, the net CO2 produced for each treatment (C-derived amendment) was calculated as the difference between the C-CO2 produced by the amended soil and that produced over the same period of time by the control soil.
The gain or loss of SOC was estimated by calculating the difference between the SOC of the amended soil and the control soil at the end of the experiment, while SOC balance was obtained by subtracting the initial from the final SOC level (Gargouri et al. Citation2014).
Mean comparison analyses using analysis of variance (ANOVA) were achieved using SPSS 13.0 for Windows (SPSS Inc.). In addition, correlations and factorial analyses were performed in order to assess the mechanisms leading to the observed effects of different EOMs.
Results and discussion
Impact of EOM addition on soil pH and EC
Soil pH is an important parameter affecting the decomposition rate of SOM due to the positive correlation between soil pH and CO2 emission from the soil (Reth et al. Citation2005). Soil pH was 7.65 for the control and OM, and 7.55, 7.48 and 7.45 for CM, CP and GW, respectively, whereas for OMW, the soil pH was 7.09, showing a significant difference from the other soils. This may be due to the acidic pH of the OMW ().
Figure 1 Evolution of soil pH on day 0 and on day 112 for the control (S) and amended soils: green waste (GW), ovine manure (OM), compost of olive husk (CM), palm compost (CP) and olive mill wastewater (OWM); lower-case letters indicate significant differences between treatments on day 0 and upper-case letters indicate significant differences between treatments on day 112.
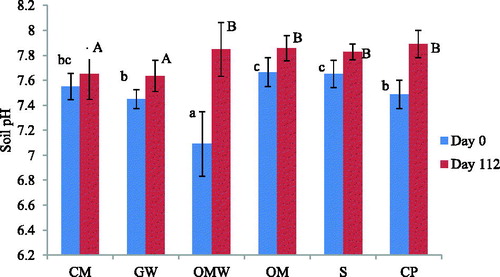
Soil pH increased slightly by the end of the experiment and the values ranged between 7.64 for GW and 7.89 for CP. Thus, the most important increase was observed for OMW, and all the soils had a quasi-neutral pH. The buffering capacity of the soil mitigated the effect of the low pH of OMW. These results agreed with those reported for OMW by López-Piñeiro et al. (Citation2008). The observed increase in pH can be attributed to the ammonia production. Similar findings were described by Odlare et al. (Citation2008), who observed an increase of soil pH after organic amendment. This was explained by the release of NH3 with the mineralization of the organic N fraction. Thus, the differences between the treatments may be related to the different amounts of NH3.
Some investigations showed that the application of organic waste as amendment in saline soil could decrease the soil pH to near neutrality (Oo et al. Citation2013; Diacono and Montemurro Citation2015).
The EC represents the soluble salt fraction of the soil. The soil studied was originally rich in soluble salts with a high EC, which at 15.06 mS/cm was considered saline soil, according to Richards (Citation1954) who proposed that saline soil has an EC above 4 mS/cm.
The EC of the treated soils initially showed insignificant increases with the addition of the amendment, to 15.08 mS/cm for CP, 15.60 for GW and OMW, and 16.83 for CM; only with manure did the EC decrease, to 14 mS/cm (). At the end, the values of soil EC were 9.58, 9.86, 9.00, 8.74, 9.61 and 9.90 mS/cm for CM, CP, GW, OMW, OM and the control, respectively.
Figure 2 Evolution of electrical conductivity on day 0 and on day 112 for the control (S) and amended soils: green waste (GW), ovine manure (OM), compost of olive husk (CM), palm compost (CP) and olive mill wastewater (OWM).
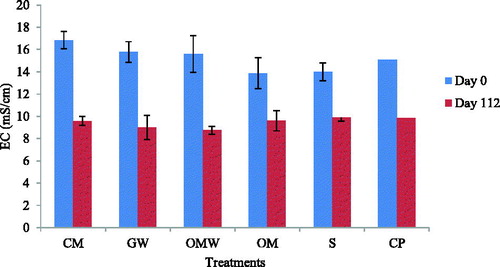
The decrease in EC content can be explained either by the fixing of soluble salts in the organic material and in the resistant micro-aggregates or by the leaching of these salts from the surface with irrigation water poured weekly when the soil samples were mostly taken from the surface (0–20 cm).
Moreover, Oo et al. (Citation2013) reported that after the application in saline soil of compost and vermicompost (compost transformed by various species of earthworms), EC was decreased. They interpreted their findings as caused by the increase of a salt-leaching mechanism. EOM addition to soils with high EC seems to reduce soluble salts within soil solution through their immobilization. However, these findings disagree with results reported by Franco-Otero et al. (Citation2012), who concluded that with the addition of EOM, EC increased from 0.18 to 0.32 dS/m, due to EOM mineralization and the addition of the soluble salts.
Evolution of ammonium and nitrate
The contents of mineral N forms (N-NH4+ and N-NO3−) were investigated in the soil during the experimental period with regard to the important effect of mineral nitrogen on EOM decomposition. It is important to recall that the NH4+ or NH3 forms are derived from the mineralization of the organic N form by heterotrophic soil organisms (Ogbazghi et al. Citation2016; Guntiñasa et al. Citation2012). Thus, ammonium and ammonia undergo nitrification and are converted into nitrate if not consumed by the plants (Whalen and Sampedro Citation2010).
During the first day after EOM application, the NH4+ content started out high, ranging between 9.78 and 11.68 mg/kg for CP and CM, respectively. Then, the NH4+ content decreased between the second and the third day to between 3.83 for OM and 4.74 for CP (, and ).
Figure 3 Ammonium and nitrate content for the control (S) and amended soils: green waste (GW), ovine manure (OM), compost of olive husk (CM), palm compost (CP) and olive mill wastewater (OWM): (a) ammonium content during the experiment; (b) ammonium content during the first week; (c) nitrate content during the experiment; (d) nitrate during the first week.
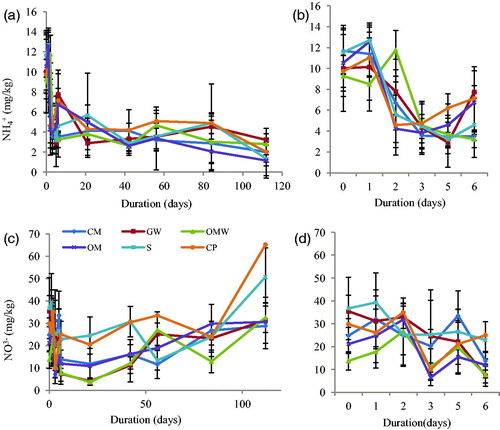
Between the third and 84th days, the ammonium content was almost constant and showed a decrease in trend at the end when the soil NH4+content was 2.01, 2.79 and 3.2 mg/kg for the CP, OMW and GW treatments, respectively, and 1.33 mg/kg for OM and the control soil. Almost the total ammonium pool was lost by immobilization, leaching or nitrification, and also ammonium evolutions in all the treated soils were not significantly different from each other or from the control during the 112 days of the experiment.
From the beginning, the NO3− content was higher than the NH4+content, ranging between 21.12 mg/kg for OMW and 36.71 mg/kg for the control ( and ). Native nitrate content was higher than that in the amended soils with a significant difference in the OMW treatment during the first day. However, after addition of organic matter, no significant difference was observed between the second and fifth days. Nitrate content was almost stable after 84 days for CP and the control. Then, an increase was observed until the 112th day: NO3− content reached 50.71 mg/kg and 65.17mg/kg for the control and CP, respectively. The increase of NO3− content in the soil can be explained by the lack of vegetation and the absence of plant roots to consume the N-NO3−. Moreover, assimilation of the N-NO3− by the soil microbes required more energy than the amount used in NH4+ assimilation. Thus, NH4+ immobilization is more efficient than that of NO3− (Romero et al. Citation2015; Eduardo et al. Citation2016).
For the other treatments, CM, GW, OMW and OM, there was a decrease in soil NO3− content between the fifth and sixth days; it was not as significant as that between the 42nd and 56th days, but it is important to note, especially for OMW and GW. Moreover, nitrate content increased at the end of the experimentation period for all treatments.
As previously mentioned, NO3− in the soil was obtained through nitrification of ammonium, which explains the opposing trends for NH4+ and NO3−. The decrease of NH4+ was accompanied by an increase of NO3− after the nitrification process.
This is based on the assumption that immobilization of NO3− by soil microbes will be faster in the presence of a labile C source used as a source of energy for soil microorganisms (Romero et al. Citation2015), and that the presence of sufficient labile C to fuel denitrification and immobilization in soil with low N content prevents nitrate accumulation in the soil (Quan et al. Citation2016).
Based on these results, and the deduction of Bernal et al. (Citation1998) that fresh organic waste products have higher amounts of C easily degradable by microorganisms than compost, it can be assumed that the reduction in NO3− in soils treated with GM, OMW or OM was probably due to the organic C available in these fresh amendments.
Effect of EOM on total N
In this study, total N content in the soil was determined at the beginning and at the end of the experiment. A significant increase was observed in N content between the treated soils and the control. On day 0, the total N content in the treated soils increased by 88%, 66% and 49% for CP, CM and GW, respectively, while for the OM- and OMW-amended soils, the N gain represented 52% (). After 112 days, the total N content in the control soil remained lower than with the other treatments. The final gain in total N was 42%, 57%, 29%, 25% and 24% for the CM, GW, OMW, OM and CP treatments, respectively, as compared to the control.
Figure 4 Total nitrogen content on day 0 and on day 112 for the control (S) and the amended soils: green waste (GW), ovine manure (OM), compost of olive husk (CM), palm compost (CP) and olive mill wastewater (OWM). Upper-case letters indicate significant differences between treatments on day 0 and lower-case letters indicate significant differences between treatments on day 112.
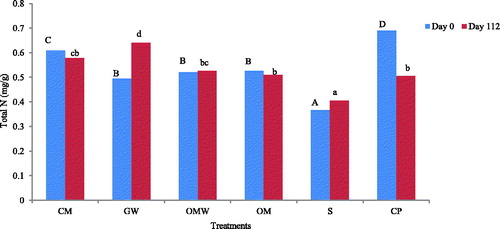
Organic and mineral fertilizers are the main sources of soil N (Flessa et al. Citation2000). Consequently, the observed increase of total N content in the treated soils at the beginning and the end of the experiment could be related to the many amendments comprising N.
The use of organic amendments should increase the total N concentration, with larger increments at higher application rates. It is apparent from these results that a higher amount of N was added to the soils with the CP application than with other EOM applications because it was added at the highest level to achieve the required amount of C. Nevertheless, with OM, the richest N amendment induced a low N content in the soil.
The soil N content was almost constant at the beginning and after 4 months for CM, OM and the control, but it increased in GW from 0.49 to 0.64 mg/g of soil and decreased for CP from 0.69 to 0.5 mg/g. Although CP had the highest NO3− content, there was a decrease in total N by the end of the 4 months.
The loss of N from the soil occurs via leaching or erosion, or by gas emissions into the atmosphere. The main cause of N loss is gas emission through NH3 volatilization or NO, N2O and dinitrogen (N2) release through activation of nitrification and denitrification processes (FAO and IFA Citation2001). Therefore, the GW nitrate content maintained immobilization by microbes to synthesize proteins and retain N in an organic form. This hypothesis is supported by the work of Fortes et al. (Citation2013) who showed that the same microorganisms take up the N mineral fraction to synthesize amino acids, proteins and other organic complexes. Therefore, the microbial biomass retains the N and then acts as a labile pool of N that is only rendered readily available to plants upon cell death, particularly if accompanied by nitrification.
Total N in the soil is constituted mostly by the organic N form which increased with EOM addition, and mineral forms are organic N mineralization products. The amendments used were rich in total nitrogen that induced many reactions and transformations in the soil via microbial activity, resulting in the mineralization of organic N and the production of NH4+ followed by NH3 and finally NO3−.
Effect of EOM on SOM
The soil studied initially had a low content of organic matter. Obviously, organic amendment when added improved SOM content. reveals that the control soil had the lowest organic matter content at the beginning and at the end of the experiment. As for the amended soils, organic matter content was immediately increased with all the treatments. This significant increase was almost the same for all amendments because the same rate of organic C was added (e.g. 350 g/m2). The SOM content ranged between 1.83% for the OMW treatment and 2.04% for the CM treatment. For the other treatments, the SOM content was 1.95, 2.00 and 2.04% for CP, OM and GW, respectively. At the end, SOM was 1.91, 1.87, 1.53, 1.45, 1.40 and 0.99% for CM, CP, GW, OMW, OM and the control, respectively. Then, soils amended with composts showed the highest SOM contents, although the same amount of C had been added at the beginning.
Figure 5 Organic matter content on day 0 and on day 112 for the control (S) and the amended soils: green waste (GW), ovine manure (OM), compost of olive husk (CM), palm compost (CP) and olive mill wastewater (OWM); lower-case letters indicate significant differences between treatments on day 0 and upper-case letters indicate significant differences between treatments on day 112.
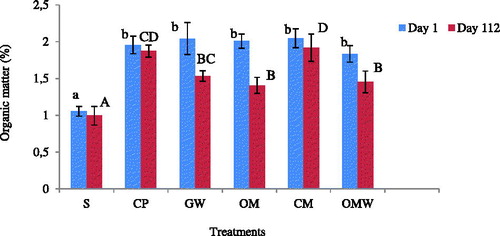
This significant difference in SOM content between treatments observed at the end of the experiment may be related to differences in the chemical composition of the amendments used. Numerous studies have shown that a higher C/N ratio may be associated with a lower decomposition rate of organic material (Majumder et al. Citation2008). This finding is in conflict with the present results. Indeed, initially, the C/N ratios for CP and CM were 19.8 and 24, respectively, lower than those for GW and OMW which were 28.5 and 27.2 (), although the highest amount of C stored in the soil was observed for CM followed by CP. Thus, in this study, C evolution in the soil was not controlled by the C/N ratio. Therefore, it can be estimated that the EOM applied here contained different amounts of resistant components such as lignin and in the soil (Bernal et al. Citation1998). Hence, the composted materials used in this experiment, which are CM and CP, may contain high concentrations of these organic constituents, improving the formation of the stable SOM that could be more resistant than those in the other treatments. This is based on the suggestion of Bruun et al. (Citation2005) and Gul et al. (Citation2014), who consider that residues with high lignin contents are more recalcitrant, are degraded more slowly and persist longer in soils than residues with low lignin contents. Moreover, the input of lignin increases SOM through its participation in humus formation.
Furthermore, the composting process produces stabilized organic matter enriched in humic substances. This process allows these substances to remain.
Soil respiration
Since the experiment was performed on clear soil without vegetation, soil respiration represents the CO2 produced by departing organisms from the soil when degrading organic matter (Schlesinger and Andrew Citation2000). For this reason, the EOM leading to the highest respiration rate is more degraded than the others. In this case, the departing population of the soil, especially microorganisms, increases dramatically (Sollins et al. Citation1996).
Soil respiration was important at the beginning of the experiment, especially for GW, which reached 1.2 g/m2/day on the second day, while composts CP and CM had the lowest respiration values: 0.6 g/m2/day and 0 g/m2/day for CP and CM, respectively (). C mineralization decreased on the third day, reaching a secondary peak on the seventh day. The quick soil respiration decreased on the third day, and its immediate recovery was also observed by other authors (Bernal et al. Citation1998; Martín et al. Citation2012). These authors explained the high CO2 emission between the first and the third days by the presence of a high concentration of easily degradable carbon, which led to a large growth of the microbial population in the soil (Bernal et al. Citation1998), or by the favorable conditions of humidity and temperature in the incubation (Martín et al. Citation2012) . On the other hand, the second peak that occurred in the same experiment may be attributed to the great variety of compounds contained in the samples and their different degrees of degradability (Bernal et al. Citation1998).
Figure 6 Net C mineralization for each treatment: green waste (GW), ovine manure (OM), compost of olive husk (CM), palm compost (CP) and olive mill waste water (OWM) during the experiment. (a) daily respiration during the first week; (b) daily respiration during the experimentation; (c) cumulative respiration.
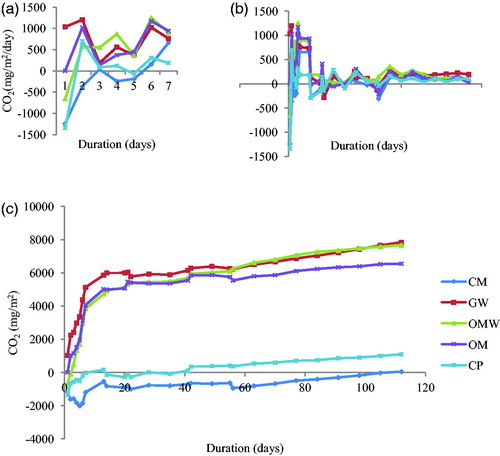
It has been demonstrated that the mineralization process is controlled by the type of organic matter introduced and the labile C content (Smith et al. Citation2015; Yazdanpanah Citation2016).Therefore, the immediate high soil respiration for GW can be explained by the fact that the addition of olive residues with high concentrations of easily degradable components could be used as energy by the decomposer microbial community, resulting in an increase of C mineralization and CO2 emission (Mahmoodabadi and Heydarpour Citation2014). Likewise, López-López et al. (Citation2012) stated that compost amendment composition and the degree of organic matter stabilization could be responsible for differences in soil respiration.
C-CO2 produced by OMW, GM and OM increased during the first week and was stabilized during the second week. The rate of CO2 production observed with these amendments was very important, while for the two types of compost, CM and CP, respiration was almost absent.
The total amount of CO2 produced during the112 days reached 7.6 g/m2 for olive residues and OMW, 6.5 g/m2 for manure, 1 g/m2 for palm compost and only 50 mg/m2 for olive husk compost ().
The large initial CO2 emission during the first week was due to the rapid mineralization of the easily degradable fraction of the organic materials, according to Martín et al. (Citation2012) who concluded that after the depletion of the most labile fraction, a constant and continuous C release from the most resistant fraction takes place. On the other hand, soil temperature increased at the end while the soil respiration was almost constant from the second week. These results confirmed the finding that soil temperature did not affect soil respiration, opposite to what was described by Ajwa and Tabatabai (Citation1994).
Soils needed 2 weeks to recover their equilibrium with regard to CO2 emission after EOM addition when using industrial wastes from fresh food, plant residues and manure. By using compost, the soil balance had not been disturbed. This was observed as a result of the partial mineralization of the compost during its production, leading to C losses throughout microbial decomposition of the labile organic matter (Bernal et al. Citation2009). Hence, during composting, stabilization of the organic matter occurred through humification, leading to the formation of stable organic complexes that are resistant to microbial decomposition (Mahmoodabadi and Heydarpour Citation2014). Therefore, the two types of compost, especially the olive husk-based compost, showed the slowest decomposition as well as the lowest mineralization rate (Cely et al. Citation2014).
Schlesinger and Andrews (2000) proposed that a small variation in the magnitude of soil respiration could strongly influence the atmospheric CO2 concentration. Therefore, compost amendments should be the most efficient for C sequestration in soil and to enhance soil fertility and consequently reduce the rate of CO2 emission, as a greenhouse gas, into the atmosphere. This is due to the fact that the compost is a fermented organic matter that had sustained the humification process (Mustin 1999).Citation
SOC gain and SOC balance
Net C mineralization was the difference between CO2-C produced by the amended soil and that produced over the same period of time by the control soil. Gain in SOC was evaluated by comparing the SOC content of treatments to that in the control, and C balance was obtained by comparing the amount of SOC at the end of the experiment to the initial amount or to every treatment ().
Table 2. Evolution of SOC for all the treatments, and evaluation of SOC gain and SOC balance.
Organic C brought by EOMs was indirectly qualified and analyzed as SOC. Nevertheless, a part of this new SOC was lost during the first few days after its incorporation. The residual part of this SOC is very important to evaluate the efficiency of every amendment for C sequestration.
After 112 days, the C balance was negative for all the treatments. The lowest SOC loss as CO2 was 0.07% for husk olive-based compost and 0.04% for palm leaf-based compost, while 0.22, 0.30 and 0.35% were the percentages of C loss during this period for OMW, GW and OM, respectively. These losses were strongly associated with respiration. Indeed, the treatments that showed the most important amount of CO2 emission had the most important C loss. C balances confirmed that composts were more stable and contain more recalcitrant organic components against microbial degradation.
When evaluating the C gain as compared to the control evolving in the same conditions, the SOC contents were improved in the amended soils. Indeed, SOM increased by 0.24, 0.26 and 0.31% for OM, OMW and GW, respectively, while the CP and CM treatments showed an important SOC gain of 0.50 and 0.53%, respectively.
This amount of C stored in the soil at the end of the experimentation period could be considered the sustainable gain of C. CM, with a low C loss and the lowest respiration, induced the most important sustainable gain of C. This relation suggests that, as for C balances, the C gain after 112 days in the soil was also related to net CO2 emission (). The observed relation confirmed that the gain was strongly and inversely associated with the amount of CO2 released from the amended soil. Moreover, the amount of initial C in the soil seemed not to affect the final C gain. Thus, the EOMs with high labile compounds that induced rapid SOM degradation resulted in weak carbon storage.
Figure 7 Projection of the different studied variables on the factorial plan. Factors 1 and 2 explained 76.42% of total observed variability.
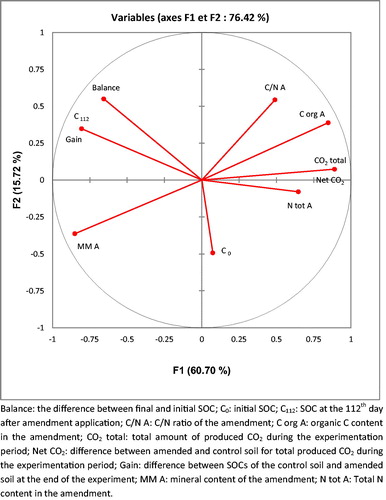
The applied organic amendments led to an increase of SOC content. This result was consistent with those reported by González et al. (Citation2010) and Yazdanpanah (2016), who observed an increase in SOC content after applying organic matter. However, the different types of EOMs did not induce the same SOC gain. Thus, not all the organic amendments could improve soil fertility and C sequestration with the same efficiency. Indeed, the type of material added to the soil had an effect. Sustainable SOC gain was greater for the composts than for other treatments although the same amount of C was added. Moreover, manure and OMW resulted in C gain corresponding to half of that obtained when using composts. Therefore, these amendments could be considered organic fertilizers with low efficiency.
This can be attributed partly to the stability of the compost against microbial degradation; it is rich with humified stable compounds as compared to other organic matter amendments and requires more time to be degraded.
Conclusion
Our results confirm that the evolution of EOM in the soil depends on the composition and maturity of the organic materials. Therefore, fresh organic material (i.e. green waste) seems to have high amounts of labile C and mineralized faster than the other treatments, whose high CO2 emission resulted in C loss. The OWM and OM amendments followed the same trend and were also easily degradable, although for the two types of compost, the stable EOM showed the most important gain in sustainable SOC accompanied by little CO2 emission. Therefore, these amendments were considered the best amendments, compared with the others, for remediation of soil fertility in terms of improving crop yields and reducing environmental problems, such as by minimizing CO2 emission.
Effectively, the two composts were more stable and mineralized slowly. This property allows the gradual release of plant nutrients and more sustainable C sequestration in the soil. Therefore, compost helps to achieve a double objective, agronomic and environmental.
Thus, in the short term, organic amendments can cause temporary disturbances of soil proprieties, but C and N balances of the treated soil were then quickly recovered.
Hence, this alternative path may improve the sustainable SOC gain of arable soil in arid regions, increasing soil C sequestration and soil fertility through the addition of stable EOM. However, the ordinary amendments used by farmers, such as manure, had low efficacy. The use of composts in agricultural lands should be favored over fresh animal and vegetable residues.
Acknowledgements
This work was carried out in the Olive Tree Institute. The facilities and services of the Olive Institute are gratefully acknowledged. The study was performed within the frame of the GEET and APOQP laboratories. It was supported by the Ministry of Agriculture and Water Resources and the Ministry of Higher Education and Scientific Research. Special acknowledgments are extended to Mr. Nabil Soua and Mrs. Mouna Khlifi for their technical help and advice. The authors acknowledge Anne Lise Haenni for her help in improving the quality of this paper.
Disclosure statement
No potential conflict of interest was reported by the authors.
References
- Ajwa, H.A. and M.A. Tabatabai. 1994. “Decomposition of different organic materials in soils.” Biology and Fertility of Soils 18: 175–182.
- Al-Adamat, R., Z. Rawajfih, M. Easter, K. Paustian, K. Coleman, E. Milne, P. Falloon, D.S. Powlson, and N.H. Batjes. 2007. “Predicted soil organic carbon stocks and changes in Jordan between 2000 and 2030 made using the GEFSOC Modelling System.” Agriculture ecosystems & environment 122: 35–45.
- Annabi, M. 2009. “Statut organique et respiration microbienne des sols du nord de Tunisie.” Biotechnologie, Agronomie, Société et Environnement 13(3): 401–408.
- Bachmann, J., G. Guggenberger, T. Baumgartl, R. H. Ellerbrock, E. Urbanek, M.O. Goebel, K. Kaiser, R. Horn, and W. R. Fischer. 2008. “Physical carbon-sequestration mechanisms under special consideration of soil wettability.” J. Plant Nutr. Soil Sci. 171: 14–26
- Baker, J. M., T. E. Ochsner, R. T. Venterea, and T. J. Griffis. 2007. “Tillage and soil carbon sequestration—What do we really know?” Agriculture ecosystems & environment 118: 1–5.
- Bernal, M., J. Alburquerque, and R. Moral. 2009. “Composting of animal manures and chemical criteria for compost maturity assessment.” Bioresource Technology 100 (22): 5444–5453.
- Bernal, M.P., M.A. Sánchez-Monedero, C. Paredes, and A. Roig. 1998. “Carbon mineralisation from organic wastes at different composting stages during their incubation with soil.” Agriculture, Ecosystems & Environment 69 (3): 175–189.
- Bot, A., and J. Benites. 2005. The importance of soil organic matter Key to drought-resistant soil and sustained food production, FAO soils bulletin. Rome, Italy.
- Bronick, C.J., and R. Lalan. 2005. “Manuring and rotation effects on soil organic carbon concentration for different aggregate size fractions on two soils in northeastern Ohio, USA.” Soil and Tillage Research 81 (2): 239–252.
- Bruun, S., B. Stenberg, T.A. Breland, J. Gudmundsson, T.M. Henriksen, L.S. Jensen, A. Korsaeth et al. 2005. “Empirical predictions of plant material C and N mineralization patterns from near infrared spectroscopy, stepwise chemical digestion and C/N ratios.” Soil Biology & Biochemistry 37(12): 2283–2296.
- Buffle J.F. 1988. “Complexation reactions in aquatic systems: An analytical approach.” Chichester UK, Ellis Horwood Ltd.
- Cabrera, V.E., L.J. Stavast, T.T. Baker, M.K. Wood, D.S. “Cram, R.P. Flynn, A.L. Ulery. 2009. Soil and runoff response to dairy manure application on New Mexico rangeland.” Agriculture, Ecosystems & Environment 131(3-4): 255–262.
- Cely, P., A. M. Tarquis, J. Paz-Ferreiro, A. Méndez, and G. Gascó. 2014. “Factors driving the carbon mineralization priming effect in a sandy loam soil amended with different types of biochar.” Solid Earth 5(1): 585–594. Doi: 10.5194/se-5-585.
- Conyers, M.K., G. J. Poile, A. A. Oates, D. Waters, and K. Y. Chan. 2011. “Comparison of three carbon determination methods on naturally occurring substrates and the implication for the quantification of ‘soil carbon’.” Soil Research 49: 27–33.
- Diacono, M., and F., Montemurro. 2015. “Effectiveness of Organic Wastes as Fertilizers and Amendments in Salt-Affected Soils.” Agriculture 5(2): 221–230. Doi: 10.3390/agriculture502022.
- Eduardo, M., L. J. Davey, W. H. Paul, and C.O. T. Paulo. 2016. “Mineral nitrogen forms alter 14C-glucose minralisation and nitrogen transformations in litter and soil from two sugarcane fields.” Applied Soil Ecology 107:154–161.
- Falloon, P., and P. Smith. 2002. “Simulating SOC changes in long-term experiments with RothC and CENTURY: Model evaluation for a regional scale application.” Soil Use and Management 18(2): 101–111. Doi: 10.1111/j.1475-2743.2002.tb00227.x.
- FAO and IFA. 2001. “Global estimates of gaseous emissions of NH3, NO and NO2 from agriculture land.” Food and Agriculture Organization of the United Nations, Rome, Italy and International Fertilizer Industry Association Paris, France.
- Ferreras, L., E. Gomez, S.Toresani, I. Firpo, and R. Rotondo. 2006. “Effect of organic amendments on some physical, chemicals and biological properties in a horticultural soil.” Bioresource Technology 97(4): 635–640.
- Fischer, H., J. Ingwersen, and Y. Kuzyakov. 2010. “Microbial uptake of low-molecular weight organic substances out-competes sorption in soil.” European journal of soil science 61(4) 504–513.
- Flavel, T.C., and D.V. Murphy. 2006. “Carbon and Nitrogen Mineralization Rates after Application of Organic Amendments to Soil.” Journal of environmental quality 35(1): 183–193.
- Flessa H., B. Ludig, Heil Merbach W., 2000. “The origin of soil organic C, DOC, and soil respiration in the continuous maize cropping system of the long term experiment “Eternal Rye” in Halle.” Journal of plant nutrition and soil sciences163, 157–163.
- Fortes, C., A.C. Vitti, R. Otto, D.A. Ferreira, H.C.J. Franco, and P.C.O. Trivelin. 2013. “Contribution of nitrogen from sugarcane harvest residues and urea for crop nutrition.” Sci. Agric 70 (5): 313–320
- Franco-Otero, V.G., P. Soler-Rovira, D. Hernández, E. G. López-de-Sá, and C. Plaza. 2012. “Short-term effects of organic municipal wastes on wheat yield, microbial biomass, microbial activity, and chemical properties of soil.” Biology and Fertility of Soils 48(5): 205–216.
- Gargouri, K., M. Masmoudi, and A. Rhouma. 2014. “Influence of Olive Mill Wastewater (OMW) Spread on Carbon and Nitrogen Dynamics and Biology of an Arid Sandy Soil.” Communications in Soil Science and Plant Analysis, 45: 1–14, Doi: 10.1080/00103624.2013.849727.
- González, M., E. Gomez, R. Comese, M.Quesada, and M. Conti. 2010. “Influence of organic amendments on soil quality potential indicatorsin an urban horticultural system.” Bioresource Technology 101(22): 8897–8901.
- Guggenberger, G.2005. “Microorganisms in Soils: Roles in Genesis and Functions.” Soil Biology 3, 10–85.
- Gul, S., S.F. Yanni, and J.K. Whalen. 2014. “Lignin controls on soil ecosystem services: Implications for biotechnological advances in biofuel crops”, in Fachuang, Lu., Lignin: Structural analysis, applications in biomaterials and ecological significance. Nova Science Publishers Inc., pp. 375–416.
- Guntiñasa, M.E., M.C. Leirósa, C. Trasar-Cepedab, and F. Gil-Sotresa. 2012. “Effects of moisture and temperature on net soil nitrogen mineralization: A laboratory study.” European Journal of Soil Biology 48, 73–80.
- Guo, L., T. Nishimura, H. Imoto, and Z. Sun.2016. “Carbon Mineralization Associated with Soil Aggregates as Affected by Short-term Tillage.” Journal of Resources and Ecology 7 (2) 101–106. Doi: 10.5814/j.issn.1674-764x.
- Hutchinson, J.J., C.A. Campbell, R.L. Desjardins. 2007. “Some perspectives on carbon sequestration in agriculture.” Agricultural and Forest Meteorology 142(1-2): 288–302.
- Kelleher, B. P., A. J. Simpson. 2006. “Humic substances in soils: are they really chemically distinct?” Environ. Sci. Technol. 40, 4605–4611.
- Krull, E.S., J.A. Baldock, and J.O. Skjemstad. 2003. “Importance of mechanisms and processes of the stabilisation of soil organic matter for modelling carbon turnover.” Functional Plant Biolog 30, 207–222.
- Lal, R. 2004. “Soil C sequestration to mitigate climate change.” Geoderma 123(01–22.
- Lal, R. 2005. “Forest soils and carbon sequestration.” Forest Ecology and Management 220 (1-3): 242–258.
- Lal, R. 2010. “Managing soils and ecosystems for mitigating anthropogenic carbon emissions and advancing global food security.” Bioscience 60(9) 08–721.
- Lehmann, J., J. Kinyangi, and D. Solomon. 2007. “Organic matter stabilization in soil microaggregates: implications from spatial heterogeneity of organic carbon contents and carbon forms.” Biogeochemistry 85(1) 45–57.
- López-López, G., M.C. Lobo, A. Negre, M. Colombàs, J.M. Rovira, A. Martorell, C. Reolid, I. Sastre-Conde. 2012. “Impact of fertilisation practices on soil respiration, as measured by the metabolic index of short-term nitrogen input behavior.” Journal of Environmental Management 113: 517–526
- López-Piñeiro, A., A. Albarrán, J. M. Rato Nunes, and C. Barreto. 2008. “Short- and medium-term effects of two-phase olive mill waste application on olive grove production and soil properties under semiarid Mediterranean conditions.” Bioresource Technology 99 (17) 7982–7987.
- Ludwig, B., E. Schulz, J. Rethermeyer, I. Merbach, H. Flessa. 2007. Predictive modelling of C dynamics in the long-term fertilization experiment at Bad Lauchstädt with the Rothamsted Carbon Model. European journal of soil science 58(5) 1155–1163.
- Mahmoodabadi, M and E. Heydarpour. 2014. “Sequestration of organic carbon influenced by the application of straw residue and farmyard manure in two different soils. International Agrophysics 28(2) 169–176.
- Majumder, B., B. Mandal, P.K. Bandyopadhyay, A. Gangopadhyay, P.K. Man, A.L. Kuudu, and D. Mazumdar. 2008. “Organic amendments influence soil organic carbon pools and rice wheat productivity.” Soil Science Society of America Journal 72(3):775–785.
- Marschner, B., S. Brodowski, A. Dreves, G. Gleixner, A.Gude, P. M. Grootes, U. Hamer, A. Heim, G. Jandl, R. Ji, K. Kaiser, K. Kalbitz, C. Kramer, P. Leinweber, J. Rethemeyer, A. Schäffer, M. W. I. Schmidt, L. Schwark, and G. L. B. Wiesenberg. 2008. “How relevant is recalcitrance for the stabilization of organic matter in soils?” J. Plant Nutr. Soil Sci. 171, 91–110.
- Martín, J.V., R. Miralles de Imperial, R. Calvo, M.C. Garcia, C. Leon–Cófreces, M.M. Delgado. 2012. “Carbon mineralisation kinetics of poultry manure in two soils.” Soil Research 50(3):222–228.
- Mathieu, C and F. Pietain. 2003. “Analyse chimique des sols Méthodes choisies.” Londres, Paris New York.
- Mustin, M. 1999. “Le compost: Gestion de la matière organique.” Ed. François Dubusc. Paris, France. pp 954.
- Nicolas, C., G. Masciandaro, T. Hern´andez, C. Garcia. 2012. “Chemical-Structural Changes of Organic Matter in a Semi-Arid Soil after Organic Amendment.” Pedosphere 22 (3): 283–293.
- Odlare, M., M. Pell, K. Svensson. 2008. Changes in soil chemical and microbiological properties during 4 years of application of various organic residues. Waste Management 28 (3): 1246–1253.
- Ogbazghi, Z. M., E. H. Tesfamariam, J. G. Annandale. 2016. Modelling N mineralisation from sludge-amended soils acrossagro-ecological zones: A case study from South Africa. Ecological Modelling 322 (24):19–30.
- Oo, A. N. C. B., Iwai, P. Saenjan. 2013. “Soil properties and maize growth in saline and nonsaline soils using cassava-industrial waste compost and vermicompost with or without earthworms.” Land Degradation & Development 26: 300-310. doi: 10.1002/ldr.2208.
- Paustian, K., J. Six, E.T. Elliott, H.W. Hunt. 2000. “Management options for reducing CO2 emissions from agricultural soils.” Biogeochemistry 48 (1) 147–163.
- Pauwels, J. M., E. Van Rust, M. Verloo, and Z. E. A. Mvondo. 1992. “Manual of soil laboratoryanalytical methods of soil and plants, 28th ed. Belgium: AGCD.
- Powlson, D.S., A. Bhogal, B.J. Chambers, K. Coleman, A. J. Macdonald, K.W.T. Goulding, A.P.Whitmore. 2012. “The potential to increase soil carbon stocks through reduced tillage or organic material additions in England and Wales a case study.” Agriculture, Ecosystems & Environment 146(1): 23–33.
- Quan, Z., B. Huang, C. Lu, Y. Shi, X. Chen, H. Zhang, Y. Fang. 2016. “The fate of fertilizer nitrogen in a high nitrate accumulated agricultural soil.” Scientific Report 6, 21539; Doi: 10.1038/srep21539.
- RampazzoTodorovic, G., G.L. Lair, and W.E.H. Blum. 2014. “Modeling and prediction of C dynamics in soil chronosequences of the critical zone observatory (CZO) Marchfeld/Austria.” Catena 12, 53–67.
- Richards, L.A. (ed.) 1954. “Diagnosis and improvements of saline and alkali soils.” United States Department of Agriculture Agricultural Research Service, Washington DC, USA. 60.160 p.
- Richards, L.A. (ed.) 1954. “Diagnosis and improvements of saline and alkali soils.” USDA. Agriculture Handbook 60. 160 p.
- Romero, C.M., R. Engel, C. Chen, and R. Wallander. 2015. “Microbial immobilization of nitrogen-15 labelled ammonium and nitrate in an agricultural soil.” Soil Science Society of America Journal 79(1): 595–602.
- Ryals, R., M. Kaiser, M. S. Torn, A. A. Berhe, W. L. Silver. 2014. “Impacts of organic matter amendments on carbon and nitrogen dynamics in grassland soils.” Soil Biology and Biochemistry 68, 52–61.
- Saffih-Hdadi, K., and B. Mary.2008. “Modeling consequences of straw residues export on soil organic carbon.” Soil Biology and Biochemistry 40 (3): 594–607. doi:10.1016/j.soilbio.2007.08.022.
- Schlesinger, W. and J, Andrews. 2000. “Soil respiration and the global carbon cycle.” Biogeochemistry 48: 7–20.
- Six, J., H. Bossuyt, S. Degryze, K. Denef. 2004. “A history of research on the link between (micro) aggregates, soil biota, and soil organic matter dynamics.” Soil Till. Res. 79, 7–31.
- Smith, P., M. F. Cotrufo, C. Rumpel, K. Paustian, P. J. Kuikman, J. A. Elliott, R. McDowell, et al .2015. “Biogeochemical cycles and biodiversity as key drivers of ecosystem services provided by soils.” Soil 1, 665–685. doi:10.5194/soil-1-665-2015.
- Sollins, P., P. A. Homann, and B. Caldwell. 1996. “Stabilization and destabilization of soil organic matter: mechanisms and controls.” Geoderma 74: 65–105.
- Stevenson, F.J. 1982. Humus Chemistry. Genesis, Composition, Reactions. John-Wiley & Sons. New York, pp 443.
- Stone, M.M., J.L. DeForest, and A.F. Plante. 2014. “Changes in extracellular enzyme activity and microbial community structure with soil depth at the Luquillo Critical Zone Observatory.” Soil Biology and Biochemistry 75: 237–247.
- Tan, K.H. 1994. Environmental soil science. New York, USA, Marcel Dekker Inc. 304 pp.
- Thangarajan, R. S., N. Bolan, G. Tian, R. Naidu, A. Kunhikrishnan. 2013. “Role of organic amendment application on greenhouse gas emission from soil.” Sci. Total Environ 465 (1): 72–96.
- Von Lützow, M., I. Kögel-Knabner, K. Ekschmitt, E. Matzner, G. Guggenberger, B. Marschner, H. Flessa. 2006. “Stabilization of organic matter in temperate soils: Mechanisms and their relevance under different soil conditions – a review.” Eur. J. Soil Sci. 57, 426–445.
- Voronin, P.Y., 2006. “Chlorophyll index and photosynthetic carbon sequestration in Northern Eurasia.” Russian Journal of Plant Physiology 53(1): 689–697
- Whalen, J. K., and L. Sampedro. 2010. “Soil Ecology and Management.” CAB International, UK.
- Xie, J., M. Hou, Y. Zhou, R. Wang, S. Zhang, X. Yang, and B. Sun. 2017. “Carbon sequestration and mineralization of aggregate-associated carbon in an intensively cultivated Anthrosol in north China as affected by long term fertilization.” Geoderma 296: 1–9.
- Yazdanpanah, N. 2016. “CO2 emission and structural characteristics of two calcareous soils amended with municipal solid waste and plant residue.” Solid Earth 7: 105–114.
- Zech, W., N. Senesi, G. Guggenberger, K. Kaiser, J. M. Lehmann, T. Miano, A. Miltner, G. Schorth.1997. “Factors controlling humification and mineralization of soil organic matter in the tropics.” Geodrema 79: 117–161.