Abstract
Sweden aims to increase biogas production from anaerobic digestion (AD) from 2 to 7 TWh/year until 2030. This paper investigates the requirements, challenges and implications of such a development through qualitative and quantitative assessment of three scenarios. Seven key elements—national policies and policy instruments, regional policies and policy instruments, mobilization of feedstock, infrastructure for feedstock and gas, mobilization of actors, new production facilities, and stable and increasing demand—were defined for the scenario construction and were also used to structure the comparative analysis. Quantitatively, increasing the biogas production from 2 to 7 TWh is estimated to require up to 5 times larger digester volume and up to 12 times more AD plants, meanwhile producing 6–8 times more biofertilizers. While a centralized production structure would be more efficient, a decentralized structure with small biogas plants would facilitate the logistics of agricultural substrates and biofertilizers. New production capacity could be incentivized through new and increased production subsidies, as well as an increased demand for renewable energy. Regardless of how the goal is to be achieved, it will require collective efforts from both public and private actors to overcome the many challenges on the way.
Introduction
Biogas solutions—systems for the production and use of biogas, biomethane and digestate—are often praised for their multi-functionality [Citation1–4]. At the same time that they contribute to increased availability of renewable energy, they can address other challenges such as improving waste handling, decreasing methane emissions, and increasing nutrient recycling in society [Citation5]. Traditionally, biogas solutions were primarily connected to waste and wastewater treatment [Citation6], but they have increasingly become a part of the efforts of developing alternative renewable energy to mitigate climate change [Citation7–10]. Concerns for sustainable agriculture [Citation11,Citation12], energy security, rural development and unemployment have also played an important role in motivating the promotion of biogas solutions in many countries [Citation13,Citation14]. This has contributed to the fast and steady growth of biogas production throughout Europe in recent decades.
In EU-28, biogas production more than doubled between 2008 and 2016 [Citation15,Citation16], but there is still great potential for further increases. For example, Kampman et al. [Citation17 estimated that another doubling would be possible within a 15-year time frame. In individual member states, even larger increases have been envisioned [Citation18–20]. However, realizing such developments will require policy measures that will radically strengthen the drivers and remove the barriers for increased production and use of biogas. Policy coherence [Citation21,Citation22], stability and continuity [Citation17, Citation23,Citation24] have been emphasized as central for policies to be effective in areas such as renewable energy that require large investments over a long period. Biogas has long been marginalized in EU policy [Citation25,Citation26], but since the launch of the European Green Deal, anaerobic digestion (AD) has been actively promoted in several policy documents as a key technology to reduce methane emissions [Citation27,Citation28], substitute fossil energy sources [Citation27,Citation29] and create sustainable food chains [Citation30. Early in 2022, the European Commission communicated its intention to double the 2030 goal on biomethane production in the EU from 17 to 35 billion cubic meters as a means to reduce dependence on imported natural gas [Citation31].
In Sweden, AD was first introduced for decreasing the sludge amounts in connection with wastewater treatment in the 1940s [Citation32] and became standard in connection with the great expansion of wastewater treatment in the 1960s [Citation33]. During the 1970s and 1980s, biogas production was introduced on some farms and pulp and paper mills, and landfill gas collection equipment was installed at large landfills [Citation33].
Until the 1990s, the produced biogas was almost only used for covering local heating needs, but as an increasing number of cities introduced biogas buses as a response to local pollution problems, upgrading of biogas to biomethane for use as vehicle fuel became the dominating use in the early twenty first century. The first co-digestion plants—mixing organic waste from industry, food waste from households and restaurants and sometimes manure—opened in the mid-1990s [Citation33]. Between 2005 and 2015, the production of such plants increased more than five times due to additional plants and improved separation of bio-waste.
This development was primarily driven by local waste companies, in close collaboration with regional transport authorities as the most important customers [Citation34]. However, an increasing share of biogas production is owned and managed by private actors, particularly large co-digestion plants [Citation35]. In other sectors such as industry and agriculture, the development of biogas production and use has been very limited. There is a large potential for increasing biogas production from mainly agricultural feedstock [Citation20,Citation36–38]. Only about 10% of the feedstock used in Swedish biogas production is from the agricultural sector, which can be compared to 55% in Denmark, 80% in Italy and France and 90% in Germany, all of which have a significantly larger biogas production than Sweden [Citation39].
Since 2015, the demand for biogas has continued to increase in Sweden, but domestic production has remained at the same level (). High production costs, along with dissonance with other countries’ support systems, have paved the ground for imported gas with green certificates [Citation20,Citation41].
Figure 1. The Swedish biogas market 2005–2020 by source [Citation40].
![Figure 1. The Swedish biogas market 2005–2020 by source [Citation40].](/cms/asset/e0880698-76fc-435c-9ead-f47a60565a21/tbfu_a_2121543_f0001_c.jpg)
Besides the price advantage for the imported gas, the Swedish biogas production seems to have been hampered by a lack of continuity and predictability of the biogas support systems [Citation42]. In contrast to many other countries, where biogas producers can be contracted with a fixed tariff for 10–20 years, the governmental support for biogas in Sweden has been up for renewal or change on a yearly basis, providing a somewhat uncertain situation for investors [Citation39]. In 2019, a national inquiry set up as a response to the stagnation of biogas production presented its results. This inquiry proposed a goal to increase biogas production through AD from 2 TWh/y (2019) to 7 TWh/y (2030). In order to achieve this, a new financial support system with premiums for the production of compressed and liquefied biomethane was proposed. The new support system was intended to be more long-term than existing financial instruments, lasting until 2040. In 2021, the parliament decided to implement a new support system, in line with what the inquiry proposed, from 2022. In the spring of 2022, the government decided to introduce a regulation on the support system, defining the conditions and levels of support [Citation43]. The production goal is formulated from a top-down perspective, specifying the contribution of different types of feedstock but not where any new production activities should take place. Moreover, the inquiry did not consider the amount of digestate produced and how that should be used.
This article presents and discusses scenarios of three alternative pathways to reach the proposed goal for anaerobic biogas production in Sweden within a decade. These scenarios have been identified and developed in a collaborative research project between researchers and biogas stakeholders within the Biogas Research Center (BRC). By investigating how this goal can be achieved, what kind of efforts it would require, and identifying challenges in connection with different developments, the aim has been to contribute to the national debate concerning the further development of biogas strategy and related policies in Sweden. The BRC is a collaboration between private companies, public authorities and organizations, Linköping University and the Swedish University of Agricultural Sciences, which was established in 2013 and is part-financed by the Swedish Energy Authority.Footnote1
In addition to this introduction, the article is structured in four sections. After an introduction to scenario approaches, the methodology section presents the scenario construction process of the study, introduces the three contrasting scenarios and explains the quantification of the different scenarios. In the next section, the scenarios are compared based on the elements that were the basis of the scenario construction. This is followed by a broader assessment and discussion of the scenarios and their respective challenges, and finally the conclusions.
Methodology
This study is centered on three contrasting scenarios for achieving the 7 TWh goal for production of biogas in Sweden. The scenarios were defined by a set a qualitative characteristics as well as quantification of the production and use of anaerobic digestion products, which were then used as basis for analysis, comparison and identification of possible challenges (). The analysis thus included policy implications, infrastructure, relevant actors, production capacity and other practical issues, while economic calculations were not within the scope of the study. The scenarios and the process of constructing them are further described in the following subsections.
Scenario approaches
Scenario approaches are often presented as indispensable tools for discussion, analysis, policy design, and decision-making related to strategic development issues, characterized by large uncertainties [Citation44]. For example, Wiek et al. [Citation45] claim that scenarios can fulfill crucial functions in relation to transition management, a practice oriented field where practitioners and researchers collaboration stimulate changes in different societal contexts [Citation46]. In such efforts, scenarios can provide the basis for knowledge integration, assessment and strategy building. By allowing different experts and stakeholders to participate in a mutual learning process, scenario construction can furthermore contribute to competence building.
Scenario approaches have been established in connection with sustainability issues since the 1960s [Citation47,Citation48]. Scenarios are neither predictions nor forecasts; they provide different possible and often contrasting images of the future and explore different development paths. There are several different typologies and terminologies to characterize scenarios and scenario projects, which sometimes lead to conceptual confusion [Citation49].
However, two basic types of scenario approaches are often distinguished: exploratory and anticipatory scenarios [Citation50]. The exploratory scenarios start in the present and project different trends into the future, which most often are based on observations in the recent past. The exploratory trend-based scenarios are either projective, based on trends observed in the past, or prospective, based on an anticipated trend [Citation51]. The anticipatory scenarios start by producing an image of a future state, which most often is either desirable (based on agreed goals), undesirable (e.g. future crisis situations) or contrasting (radically different futures), followed by asking questions on how to get there, or how to avoid such a situation. The process to concretize such a development through following it back to the present is called backcasting [Citation52], which is sometimes presented as a particular scenario category [Citation49].
Contrasting scenarios are a cornerstone in scenario planning [Citation53], which, by taking alternative diverging futures into consideration, aims to improve policy makers’ ability to act despite large uncertainties. Another useful distinction can be made between methodological and informal approaches [Citation54,Citation55]. In the methodological approach, a certain method or model is employed for ensuring replicability, while the informal scenario construction differs from situation to situation. This does not mean that informal scenarios are unstructured or lack logical consistency but rather have greater flexibility. The methodological scenarios are predominantly quantitative, while the informal scenarios most often are based on qualitative analysis.
In recent decades, there have been increasing efforts to combine quantitative and qualitative data for framing and constraining actor-based storylines [Citation56], and an increasing number of scenario studies addressing the possible transition to a low-carbon-society [Citation57–59]. With the intention to address system innovation and identify possible transition pathways, the concept of a socio-technical scenario was first launched as a reaction to the predominance of computer model-based methodological scenarios and their inability to include sociopolitical developments [Citation60,Citation61]. To better incorporate sociopolitical developments, recent sociotechnical scenario studies [Citation57,Citation59,Citation62] recommend that socio-technical scenario construction combines quantitative scenario modeling, qualitative storylines and socio-technical system analysis. It is not sufficient for a scenario to be logically consistent; it also needs to be plausible, socially acceptable and politically feasible to be taken seriously and serve its purpose [Citation57]. To achieve these goals, the scenario must be efficiently communicated, well-motivated and explained from current conditions, expectations, goals and values. In terms of communication, it is an advantage if the scenario builds on a distinct idea, which is typical for contrasting scenarios.
Scenario construction
The scenarios developed for this study are of the anticipatory type. They are inspired by socio-technical scenarios [Citation57,Citation59,Citation62] in that they combine quantitative and qualitative analysis, and the construction is based on participatory processes. Three different ‘contrasting’ scenarios were constructed to describe alternative ways to reach the common future goal of 7 TWh biogas production from anaerobic digestion in Sweden. The scenarios have a common frame but are based upon different assumptions of changes in key policies and support systems over the period, and they discuss and illustrate the effects of such differences.
The goal of the scenario construction and presentation is to fulfill three major conventional scenario requirements: the scenarios should be easy to understand, the development of the scenarios and their central assumptions should be satisfactorily explained and motivated and the scenarios must be adapted to their purpose [Citation54,Citation57]. In our case, the purpose is to offer a frame for strategic biogas policy discussions in Sweden. To make the scenarios comprehensible, we have chosen to develop contrasting scenarios, which are based on distinct ideas rooted in the history and debate on biogas solutions in Sweden and other countries. We make efforts to explain and motivate the assumptions behind the scenarios to increase their credibility and comprehensibility. The quantification of the different scenarios is based on consistently applied assumptions and procedures, which are explained to increase transparency.
The process of developing the scenarios has been part of the activities of the Biogas Research Centre and the research area focusing on biogas policy in Sweden and internationally. The partners in the research area—researchers and representatives for BRC partners (municipal and county officials, biogas producers, technology providers)—have had four joint meetings per year from 2019 through 2021. To develop scenarios for the future biogas development was part of the agenda of the research area from its start, but we awaited the results of the national inquiry in December 2019 to launch the process.
The scenario development process is summarized in , which describes it as a six-step process. The first step consisted of establishing the goal. We decided to focus on achieving the goal of 7 TWh biogas from anaerobic digestion that was proposed by the national inquiry. From a scenario development point-of-view, this goal is appropriate since it is a very bold one. What is more problematic from a scenario development perspective is the year the goal should be fulfilled since it is difficult to make such a radical development plausible within such a short time frame. Due to the slow policy process following the national inquiry, such a radical development until 2030 has become even less plausible. Therefore, we have rephrased the time frame of the scenarios, from ‘until 2030’ to ‘within a decade’.
From 2020 to 2021, we devoted two research area meetings to scenario workshops. In the first workshop, we focused on the question, ‘How is the 7 TWh goal achieved by 2030?’. The workshop discussion provided a base for the scenario definition, where the type of scenarios, key elements in the scenarios and three different contrasting development paths for production and use of the biogas and biofertilizers were addressed. Following the first workshop, three different scenarios were defined:
Large-scale centralized—Accelerated, but similar development as in the last few decades, dominated by larger co-digestion plants
Decentralized—Focused on small-scale agricultural AD for maximized utilization of agricultural substrates and nutrient recycling
Regional systems—Demand-driven development with large industries in focus
Furthermore, seven key elements were defined for characterizing and comparing the scenarios. The key elements each represent conditions that have to be met for the stipulated development to take place:
National policies and policy instruments
Regional policies and policy instruments
Mobilization of feedstock
Infrastructure for feedstock and gas
Mobilization of actors
New production facilities
Stable and increasing demand
These elements were first used for characterizing the scenarios and later as a basis for the comparative analysis in Section 3.
At Workshop 2, the three scenarios were further elaborated on with discussions particularly focusing on how they can be realized, identification of particular challenges for their realization and how to overcome these challenges.
After further elaboration of the scenarios, they were discussed and assessed in a series of workshops with four junior researchers focusing on the particular challenges of each scenario.
Overview of the scenarios
Scenario 1: Large-scale centralized development
The first scenario is essentially an extension of current strategies in Sweden, with a focus on large substrate streams and large production facilities.Footnote2 In order to maximize the advantages of scale, new AD plants will mainly be located in areas where large amounts of substrates can be procured within a feasible distance, rather than having several smaller AD plants closer to each substrate source. Large, private biogas companies continue to lead the expansion, favoring new AD plants that can give a good turnover financially. Most of the biogas is produced in relatively large co- digestion plants, upgraded and used as a transport fuel.
Scenario 2: Decentralized development
In contrast, the second scenario builds on a more decentralized expansion of the biogas production, where challenges of nutrient circulation, agricultural methane emissions and circular agriculture are in focus, similar to the biogas development in France [Citation39,Citation63] and the explorative study by Metson et al. [Citation64], which investigated how the use of domestic excreta resources could be maximized in Sweden. New AD plants are generally much smaller and are located at or near cattle farms, close to agricultural substrate sources as well as crop fields where digestate from the AD can be applied as fertilizer. The smaller scale makes upgrading to biomethane less feasible, and the local use of biogas for heat and electricity production increases at the expense of use in transports and industries.
Scenario 3: Regional systems-driven development
In the third scenario, significant consumers of gas such as large factories, transport companies and shipping companies drive the development through an increased demand for biomethane as an energy carrier or AD technology for waste management. This scenario was inspired by a research project involving a Swedish steel producer [Citation65], and it departs from the challenge of increasing the share of renewable energy in manufacturing and transport. Stricter regulations on renewable energy use and GHG emissions, as well as customer requirements on more sustainable products and services, make these actors take on an active role in the development of biogas production, in collaboration with biogas companies. Hence, the largest share of the biogas produced will be used in the manufacturing industry. The production development in this scenario is a mix of large centralized and small decentralized AD plants, maximizing the regional production for use in major industries.
presents a summary of the three scenarios, characterized through the key elements identified.
Table 1. Summary of the three scenarios for production and use of biogas/biomethane and biofertilizers.
All scenarios are based on a similar basic national policy framework, which consists of:
Continued tax exemption for the use of biogas and biomethane
No tax exemption for imported gas without green certificates
Continued investment support for new facilities and gas vehicles
Continued methane reduction support for manure digestion
New, long-term production support for biogas and biomethane
Facilitated procurement of gas vehicles and use of biomethane in road transport
The combination of these measures was considered required for the fast growth in all the scenarios, but the set-up, level and importance of various instruments differ to some extent between the scenarios. Exemption from energy and CO2 taxes has been a cornerstone in the Swedish support system for bioenergy [Citation20].
Keeping the tax exemption is favorable over an equivalent increase in production support for several reasons. As a part of the state tax system, the tax exemption is potentially more stable and foreseeable than support granted through the governmental budgets. Secondly, supporting both the production and use of biogas may increase the possibilities that both production and use take place within the country and that benefits are gained from the whole value chain [Citation20, Citation39]. It should, however, be kept in mind that the total subsidies for renewable energy must not exceed the difference in production costs compared to cheaper alternatives [Citation66], such as fossil fuels. Furthermore, introducing a CO2 tax on renewable energy sources would equate renewable carbon with fossil carbon. For the same reason, gas that cannot be proven to be renewable through, for example, certificates of origin should not be eligible for tax exemption. Currently, large volumes of biomethane are imported to Sweden from Denmark [Citation40], as the Swedish tax exemption in combination with the Danish support system for domestic biogas production makes Danish biogas very competitive on the Swedish market. While the customers in Sweden can count their gas consumption as renewable, the green value on a national level is only accounted for in the Danish system [Citation20,Citation67].
Investment support for new biogas production facilities, upgrading and liquefaction plants, fueling stations and vehicles will continue to be important for decreasing barriers related to capital costs for producers and customers. In addition, continued methane reduction support for manure digestion and the new production support system introduced in 2022 are deemed to be required to make the biogas price more competitive.
Municipalities, regions and companies buying transport services are important for the use of biomethane in the transport sector, which is the major offset for biogas in Sweden [Citation40]. Several studies have shown biomethane to dramatically reduce the climate impact of road transports compared to fossil fuels [Citation9,Citation67–70]. Procurement of biomethane in public transport and heavy goods transports can create a stable demand for the produced biogas, thereby stimulating an increased production. It is, therefore, important that the climate performance of gas vehicles is acknowledged in national policy to enable their inclusion in green procurement.
Quantification of the scenarios
Using 2020 data as the starting point, the production and use of biogas and biofertilizers, as well as the number and volume of biogas plants, were calculated for each scenario. Regarding the AD plants, the required digester volume and number of AD plants were calculated based on the average size of AD plants of the respective category in Sweden (Appendix A, ). For all scenarios, it was assumed that losses are kept at a similar level as in 2020, and flaring is reduced from 11% to 1%, as the market conditions and technical development will enable nearly full utilization of the biogas that is produced. The share of digestate available for use as biofertilizers was assumed to be on the same level as in 2020 for different types of biogas plants: 100% from agricultural and co-digestion plants, 43% from wastewater treatment plants (WWTP) and 33% from industrial AD plants [Citation40].
The calculations of biogas and digestate production were based on the biogas production per substrate type, the assumed division of substrates per type of biogas plant (Appendix A, ), the biogas yield per substrate type (Appendix A, ) [Citation36–38,Citation40,Citation71–74] and the typical methane content in produced biogas (Appendix A, ) [Citation40]. The biogas production per substrate type was based on the estimated potential for each substrate type (Appendix A, ), with some variation in the use of substrates between the scenarios. Six substrate types (municipal solid organic waste
(MSOW), municipal wastewater, manure, industrial food waste, ILUC-free cropsFootnote3 and industrial wastewater) were considered. In all three scenarios, the majority of the increased biogas production was assumed to come from manure and ILUC-free crops, as this is where the largest potential exists [Citation20,Citation36–38]. The biogas production from MSOW was assumed to be limited by the degree of sorting food waste from other household waste, as well as by efforts to reduce food waste [Citation38]. For municipal wastewater, increasing population and technical efficiency improvements were assumed to make a small increase in biogas production possible [Citation20]. The substrates were divided among four types of biogas plants: wastewater treatment plants (WWTPs), co-digestion plants, agricultural plants and industrial plants. In the statistics for 2020, 300 GWh of the biogas production is categorized as landfill gas or ‘other’, which are not included in the calculations.
Comparative analysis of the scenarios
National policies and policy instruments
In the large-scale centralized scenario, investment support for new production plants is directed mainly toward relatively large AD plants. In addition, policy instruments promoting the production and use of compressed and liquefied biomethane are central on the national level. Production support for upgraded and liquefied gas may also indirectly favor large-scale production, as these technologies require a certain scale to be feasible. Similarly, the regional systems scenario requires support for upgrading and liquefaction, although with industrial use rather than transport as the main target for the use of biogas. This scenario also demands stricter requirements on corporate environmental management, GHG emission reduction and the use of renewable energy. The reason for this is that the energy prices for Swedish industry have until recently been too low to motivate large investments in renewable energy. Increased CO2 emission price in the EU Emissions Trading System (ETS), which large industrial plants are part of, will contribute to this scenario. For the decentralized scenario, national policies will instead focus more on sustainable agriculture, nutrient circulation and relatively small (agricultural) biogas plants. This includes increased incentives for anaerobic digestion of agricultural waste and by-products as well as for the use of digestate as biofertilizers.
Regional policies and policy instruments
On the regional and local level, collaboration between regions and municipalities will be required to get the most out of the biogas potential in the large-scale scenario. The feedstock uptake area and gas market for new large-scale biogas plants may stretch across more than one municipality or region. The planning of new biogas plants must therefore be coordinated to avoid local feedstock deficit. When it comes to the market for biogas, regions and municipalities will contribute by requesting gas vehicles in transport procurements.
In the decentralized scenario, municipalities and regions will be important for coordinating local efforts for biogas solutions, sustainable agriculture and rural development. Farmers may not have the know-how and the resources needed to initiate a biogas project, in which case the municipality, region or national agriculture organization can act as intermediaries between, for example, farmers and biogas companies [Citation75]. Regional or municipal sustainability goals can help to motivate biogas development.
Similarly, the regional systems scenario requires regions to coordinate industries, biogas producers and substrate owners such as farmers. Regional sustainability and self-sufficiency goals inspire a holistic view of local production and use of energy from renewable sources. Competition to become the most sustainable city or region can be a regional driving force for increased engagement in biogas solutions.
Mobilization of feedstock
All three scenarios are based on that the largest potential for biogas production in Sweden is found in agricultural substrates (manure and crops). The large-scale centralized and regional systems scenarios also rely on increasing the use of other feedstock, such as municipal solid organic waste (MSOW), and industrial food waste and wastewater (). The decentralized scenario mainly exploits the potential of agricultural substrates—both manure and ILUC-free crops—which demands small AD plants in remote agricultural areas and rather isolated farms. Compared to the current situation, all the future scenarios require a dramatic increase in the digestion of manure and ILUC-free crops such as ley crops from fallow land and sequential crops.
Infrastructure for feedstock, digestate and gas
Depending on the location of new biogas plants in relation to substrate providers, farmland and biogas users, the infrastructure for feedstock, digestate and gas will require improvements as well as expansion. In the large-scale centralized and regional systems scenarios, the distance from production plant to user can, in many cases, be quite long and require liquefaction or access to a gas grid (which is currently limited to the southwest coast and a few local grids). Distribution of feedstock and digestate will—especially in the large-scale centralized scenario—require transports over longer distances than in the decentralized scenario, where the biogas plants will be located near both the feedstock supply and farmlands where the digestate can be applied with a minimum of transport. In some cases, dewatering of wet substrates and digestate will be required in the large-scale centralized scenario to make distribution more efficient.
Some of the large-scale AD plants will be placed in rural areas to minimize the transport distance for agricultural substrates and digestate, with additional feedstock being transported from urban areas. In the decentralized scenario, biogas from nearby agricultural AD plants will sometimes be distributed via a low-pressure pipeline to centralized upgrading and liquefaction plants to enable agricultural biomethane production.
Mobilization of actors
Large, established companies specializing in the production and sale of biogas, with their expertise and financial strength, will be important actors in all three scenarios. Meanwhile, as most of the unused potential for biogas production lies in agricultural substrates, farmers will also be important for the supply of substrates, production of biogas and use of digestate. The large-scale centralized scenario relies mainly on large, existing biogas producers to continue to expand their business and produce more biogas. In the decentralized and regional systems scenarios, farmers and industries, respectively, will take on more advanced roles with the support of large biogas companies that provide expertise. The biogas companies will own a large part of the infrastructure and run production in these scenarios. The regional systems scenario is the most user-centered of these scenarios, as industries that are interested in using the biogas will play a major role as customers and organizers in different regional developments, while the decentralized scenario is framed around the agricultural substrate and digestate streams and the farmers that own them.
New production facilities
A dramatically increased biogas production will require an equally radically increased volume of anaerobic digesters and a large number of new biogas plants (). The substrate mixes and production strategies in the three scenarios will lead to different compositions of biogas plants. Co-digestion, agricultural and industrial plants will all increase significantly in all three scenarios, but to varying degrees. Agricultural AD plants are generally much smaller than co-digestion plants, and the methane yield of manure is relatively low, which means that the decentralized scenario requires a larger digester volume and a larger number of new plants. In the large-scale centralized scenario, new AD plants will be concentrated in regions with high feedstock density, for example, areas with large populations of people or cattle. This means that the development of new AD plants will mainly be concentrated in the southern parts of Sweden. In the regional systems scenario, the placement of new AD plants will depend both on feedstock density and the vicinity to large biogas-using industries. In the decentralized scenario, the AD plants will be more evenly distributed across agricultural areas all over the country.
Figure 5. Number of biogas plants and total digester volume by type of production plant in 2020 and the three future scenarios.
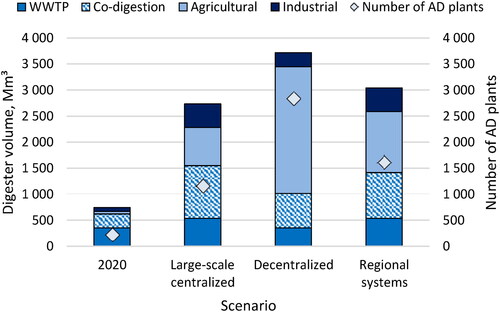
Most of the biogas production increase toward the 7 TWh goal will take place in co-digestion and agricultural AD plants (). Co-digestion plants will account for a majority of the biogas production in the large-scale centralized and regional systems scenarios, while in the decentralized scenario, the biogas production for agricultural plants is slightly higher than for co-digestion plants.
The digestion of agricultural substrates is currently divided between co-digestion plants (64%) and agricultural plants (36%) [Citation40]. In the three future scenarios, the division is assumed to be 75/25, 40/60 and 60/40, respectively (see in Appendix A). Thus, in the decentralized scenario, there will be more dedicated agricultural plants taking care of local substrates, while in the large-scale centralized scenario, a larger share of the agricultural substrates will be co-digested with other types of substrates. The regional systems scenario represents something of a mean when it comes to where the agricultural substrates are handled, with a larger share going to agricultural plants than the large-scale centralized scenario but a smaller share than in the decentralized scenario.
Likewise, the production of biofertilizers will depend on the amount and type of substrates used (). The highest biofertilizer production is seen for the decentralized scenario and is linked to the low yield of methane per ton substrate. In all scenarios, the share of digestate used as biofertilizer is very high, 96–98%. This is because of the large share of biogas being produced in agricultural or co-digestion plants, where 100% of the digestate is currently used as biofertilizer. Compared to 2020, the future scenarios have 6–8 times higher biofertilizer production, in terms of wet weight. This will mean certain logistical challenges, especially for centralized biogas plants, but will, on the other hand, give improved opportunities for organic farming.
Stable and increasing demand
Due to the importation of biomethane and trading of gas with green certificates, the use of biogas and biomethane in Sweden was about twice as high as the domestic production in 2020 (). In the scenarios, it was assumed that the imported gas is either replaced by domestic biogas or the demand is high enough to accommodate both 7 TWh domestic production and possible imports. However, the figure only includes future use of domestically produced biogas and biomethane. In the large-scale centralized scenario, a majority of the biogas will be upgraded (about 6 TWh) and used as a transport fuel (2/3 of the upgraded biogas) or in industrial applications (1/3 of the upgraded biogas). In the regional systems scenario, an equal amount of biogas will be upgraded, but the relation between industrial use and use as a transport fuel will be the opposite, as a large share of the demand will come from the industrial sector. The decentralized scenario has a lower share of upgraded biogas and higher shares of local use for heat and electricity production.
Scenario assessment and discussion
Centralized or decentralized production?
In the workshops, the decentralized scenario was deemed the most difficult to realize due to the practical and economic disadvantages of establishing and running such a large number of small biogas plants. Historically, the development of the production and use of biogas in Sweden mostly resembles the large-scale centralized scenario, mainly driven by municipalities and large companies and based on large waste streams. Although centralized production is more efficient, a large share of the unused substrate potential in Sweden is distributed over large parts of the country and, therefore, perhaps more accessible with a decentralized production model [Citation75]. Thus, a combination of these strategies may be required to achieve the production goal.
Moreover, a decentralized production would facilitate the use of digestate as a biofertilizer, thereby improving nutrient circulation [Citation64], which would align well with the EU’s strategy on sustainable food production [Citation30]. While a more detailed analysis would be required to estimate the nutrient contents, it is clear from the scenario results that the amount of digestate available as biofertilizer will increase a lot if the biogas production in Sweden reaches 7 TWh/y. From a climate impact perspective, focusing biogas production on agricultural feedstock may be preferable, as anaerobic digestion of manure is an efficient way of reducing methane emissions [Citation28,Citation76].
Administration
Which strategy will be employed may depend on which authority will be responsible for the biogas development. So far, the administration of biogas issues in Sweden has been split between several authorities in different sectors [Citation39]. The national inquiry on biogas development proposed in 2019 a more simplified governance, placing most of the responsibility with the Ministry of Agriculture [Citation20], which could give an increased focus to decentralized biogas solutions in the agricultural sector. In March 2022, the Swedish government decided to hand the responsibility of the new biogas support system to the Energy Agency [Citation43], which could bring a stronger emphasis on biogas as an energy carrier and less focus on digestate and the agricultural sector. While a centralized administration can be positive with respect to policy harmonization and overview, it is also important to acknowledge the multifunctionality of biogas solutions and to avoid suboptimal strategies that ignore some of their functions.
New production capacity
The development of new biogas plants in the decentralized scenario can be compared with the study by Metson et al. [Citation64], where new AD production facilities are distributed across Sweden to optimize the transport of substrates and digestate while also accommodating the agricultural demand for nutrients. Unlike their idealized model, however, the location of new biogas plants will most likely not be planned top-down to optimize logistics. Instead, each plant will probably be planned and built without much consideration of other new and future projects, based on the availability of substrates or demand for AD products. This may lead to situations where two or more biogas plants compete for the same substrates. In other cases, there will be potential sources of substrates that are not used because they are considered too remote for transport to a large biogas plant or too small to support a local biogas plant. One important observation from this study, supported by Metson et al. [Citation64], is that the logistics of substrates and digestates will become increasingly important with a growing interest and priority in the use of agricultural substrates. This may lead to a larger number of biogas plants in agricultural areas, as it is easier to transport gas than large volumes of agricultural substrates and digestate. The development of agricultural biogas production can be facilitated by improved technologies for small-scale gas upgrading and liquefaction, allowing smaller AD plants to deliver compressed or liquid biomethane, for example, for transports and receiving a higher income on their product. Furthermore, power-to-gas technologies for the methanation of CO2 from biogas have the potential to increase the methane yield of AD plants [Citation77,Citation78] and contribute toward the 7 TWh goal, the main barrier being the access to hydrogen for methanation.
Lessons to learn from other countries
Countries like France, Denmark, Germany and Italy have—for various reasons—been far more successful than Sweden when it comes to utilizing agricultural substrates for AD [Citation39]. This can partly be explained by the access to a gas grid, which facilitates the distribution of produced biomethane, or that relatively high electricity prices have made agricultural AD for electricity production more feasible, but there are also differences when it comes to historic strategic choices and how the biogas sectors have spawned in the respective countries [Citation39]. In contrast to the Swedish case, France has had a more pronounced strategy on nutrient circulation [Citation63], Germany and Italy have gone for AD with energy crops to produce renewable electricity [Citation79], and in Denmark, AD started as a way to produce energy from manure [Citation80].
Moreover, there are large differences in scale of AD plants in different countries; in 2020, the average AD plant in France or Italy produced nearly twice as much energy as the average Swedish AD plant, while the energy production of Danish AD plants was on average more than four times as high compared to the Swedish AD plants [Citation39]. Thus, it is difficult to draw from the development in these countries and translate to the Swedish situation. On the other hand, Norway and Finland both have similar challenges as Sweden when it comes to gas infrastructure and utilizing agricultural substrates and similar support systems for AD [Citation39]. Therefore, the findings of this study should be transferable to those countries to some extent.
Supply and demand
Up to this point, the demand for biogas in Sweden has increased continuously.
However, due to the limited gas infrastructure in Sweden, the markets for biogas and biomethane are still mainly local. For new biogas projects, there is, therefore, a risk that the mutual dependence between production and demand creates a ‘Catch-22’ situation, where the lack of a local market limits the willingness to invest in new production, and the lack of local supply makes companies and municipalities reluctant to invest in, for example, gas vehicles. The absence of stable long-term policy frameworks and support systems increases the risk of such investments for both producers and consumers [Citation24,Citation81,Citation82], and this is likely to have impeded several potential biogas projects in Sweden. In many of the biogas projects that have been realized, municipalities have provided stability in ownership and market by being involved both in the production and use of biogas [Citation35,Citation83,Citation84]. The regional systems scenario, with private customers taking more responsibility for driving the demand, can be expected to have a similar effect on the production. It requires, however, an increased motivation from these customers to switch to renewable energy and a higher willingness to pay, which can be created either by stricter regulations or through requirements from their customers on a greener value chain.
EU policy development
On the EU level, the policy framework for biogas is going through a phase of rapid development in the wake of the European Green Deal. Still, it is not very supportive when it comes to the use of biomethane as a transport fuel, at least not for light-duty vehicles. In 2021, the European Commission announced an intention to ban new cars that are not so-called ‘zero-emission vehicles’ by 2035 [Citation85]. Many member states have followed by introducing corresponding national targets, although a Swedish inquiry on this subject proposed to ban fossil fuels rather than specific engine technologies [Citation86]. Norway, which is not an EU member but is implementing most EU directives and regulations related to energy and the environment, recently included biogas in the ‘zero-emission’ definition [Citation87]. Meanwhile, heavy-duty transports remain as a feasible offset for biomethane, as they are not under the same ‘zero-emission’ requirements. When it comes to the maritime sector, the life-cycle perspective is currently more pronounced in EU policy than it is for road transports, and the revised Fuel EU Maritime Regulation introduced a ‘well-to-wake’ (from the extraction of energy resources to use in the vessel) perspective, encouraging the further reduction of GHG emissions and reduced use of fossil fuels [Citation88]. This opens up for increased use of liquefied biomethane, which is technically uncomplicated to mix with liquefied natural gas, in the maritime sector.
Energy security as a possible driver for biogas
Recent studies have brought up the potential contribution of biogas and biomethane to a more secure and flexible energy supply to prevent dramatic price fluctuations and increase local self-sufficiency [Citation89,Citation90]. In light of the historically high energy prices in Europe during the winter of 2021–2022, the interest in locally produced renewable energy may increase more rapidly than expected. The price of natural gas has varied from 75 to 190 €/MWh [Citation91], which can be compared to the average production cost of biomethane in Sweden at 60–100 €/MWh [Citation20]. Furthermore, the Russian war on Ukraine in 2022 immediately led to economic sanctions from the EU [Citation92] and made the European Commission set a target to produce 35 billion m³ (about 350 TWh) of biomethane in the EU by 2030, to reduce the European dependence on Russian natural gas [Citation31]. Such sudden changes of conditions, or ‘landscape shocks’ [Citation93], can sometimes completely change the trajectory of the ongoing development. For example, the 1973 oil crisis incited efforts toward energy efficiency and finding alternative energy sources [Citation94,Citation95], and the 2011 nuclear accident in Fukushima, Japan, led to Germany rapidly phasing out all its nuclear power [Citation96]. Combined with the COVID pandemic outbreak in 2020—which can also be described as a landscape shock of severe magnitude [Citation97]—the situation on the European energy market may indeed contribute to an accelerated development of resilient, renewable energy production.
Reaching the 7 TWh goal
Through the assessment of the three scenarios, this study contributes to the knowledge on the many barriers and challenges to overcome for biogas projects to be realized [Citation98–100]. Having explored these three scenarios and their respective challenges, it should be noted that increasing the biogas production from 2 to 7 TWh/year will require collective efforts at a scale unprecedented in the history of the Swedish biogas sector. Doing it by 2030 seemed like a herculean task in 2019 when the goal was launched, and the development has not really picked up speed since. The decision to implement a new production support system could give the sector a firm push in the right direction. However, the main critique against the new production support from our workshop participants was that it would probably only suffice to salvage the current level of biogas production and level out the competition against imported biogas. A few other countries, like Germany and Denmark, have succeeded in achieving similar levels of biogas production (per capita) as Sweden is aiming for [Citation39]. The transferability of support systems and other policy instruments is of course limited due to differences in contextual factors such as national policy frameworks and structures. Both Germany and Denmark have been successful in implementing support systems that provide strong incentives for biogas producers, both in terms of financial value and duration, which makes investments less risky [Citation39]. These factors were evidently taken into account when the new, long-term support system for Swedish biogas producers was proposed [Citation20]. As this scenario study shows, there are also challenges related to mobilization of actors and feedstock, infrastructure, administration and market for the AD products to deal with if the 7 TWh quest is to succeed.
Conclusions
This study has presented three scenarios constructed to represent three distinct conceptual pathways toward the Swedish 7 TWh goal for biogas from anaerobic digestion. Through a quantitative and qualitative assessment, the challenges, strengths, weaknesses and implications of each scenario were discussed based on seven key elements: national policies and policy instruments, regional policies and policy instruments, mobilization of feedstock, infrastructure for feedstock and gas, mobilization of actors, new production facilities, and stable and increasing demand.
Sweden has come relatively far when it comes to implementing AD around urban and industrial feedstock sources, but the rural (agricultural) potential is largely unexploited. Going from the current 2 TWh/year to 7 TWh/year, the agricultural sector would have a more central role in biogas production, as well as in the use of both biogas and digestate. Because of the relatively low methane yield and the geographical distribution of agricultural substrates, an increase from 2 to 7 TWh would require up to 5 times larger digester volume and up to 12 times more AD plants. Meanwhile, it would render 6–8 times more biofertilizers, thus improving the conditions for organic farming and nutrient recycling. The use of agricultural substrates and the distribution of biofertilizers are perhaps best facilitated through a decentralized production strategy, with a large number of small AD plants across the country. However, given the challenges with the efficiency of small-scale biogas and biomethane production, a more centralized model may be easier to realize, even though the logistics of substrates and digestates would be more complex. For a decentralized structure to develop, more focus would have to be put on agricultural methane emissions reduction and nutrient management.
The 7 TWh production goal is the first step toward a more organized, strategic and holistic handling of the Swedish biogas sector. The next step, as explored in this article, is to recognize the implications of this goal and how it can be achieved. In qualitative terms, it will take collective efforts from both public and private actors on different levels to make the necessary policy decisions, build up infrastructure and production capacity, and develop the market for AD products. Long-term conditions and financial instruments could stimulate investments in new production capacity, as examples from other countries have shown. In addition, regional and local planning and coordination could facilitate the development and contribute toward the 7 TWh goal. Future studies could include the economic implications and cost-efficiency of different strategies for achieving this goal.
Acknowledgements
We would also like to thank the workshop participants for providing valuable inputs to the study, as well as our internal review group: Sofia Dahlgren, Linda Hagman, Wisdom Kanda, Axel Lindfors and Thomas Magnusson.
Disclosure statement
No potential conflict of interest was reported by the authors.
Additional information
Funding
Notes
1 In 2022, BRC transformed into the Biogas Solutions Research Center (BSRC), a 5-year programme with a similar set-up as the BRC but with an expanded number of partners.
2 For reference, in 2020, the average Swedish co-digestion plant produced 30 GWh biogas per year, while an agricultural AD plant produced on average 1 GWh/year.
3 ILUC-free crops = crops that do not cause indirect land-use change (ILUC), such as ley crops from fallow land, catch crops and crop residues, but not including dedicated energy crops.
References
- Hagman L, Blumenthal A, Eklund M, et al. The role of biogas solutions in sustainable biorefineries. J Clean Prod. 2018;172:3982–3989. https://doi.org/10.1016/j.jclepro.2017.03.180.
- Kiselev A, Magaril E, Magaril R, et al. Towards circular economy: evaluation of sewage sludge biogas solutions. Resources. 2019;8(2):91. https://doi.org/10.3390/resources8020091.
- Lindfors A, Feiz R, Eklund M, et al. Assessing the potential, performance and feasibility of urban solutions: methodological considerations and learnings from biogas solutions. Sustainability. 2019;11(14):3756. https://doi.org/10.3390/su11143756.
- Richardson D. Farmers in rural Africa turn to biogas solutions to enable energy independence and cut deforestation rates. Gas Int. 2012;9:22–23.
- Hagman L, Eklund M. The role of biogas solutions in the circular and bio-based economy. Linköping, Sweden: Swedish Biogas Research Center; 2016.
- Scarlat N, Dallemand J-F, Fahl F. Biogas: Developments and perspectives in Europe. Renewable Energy. 2018;129:457–472. https://doi.org/10.1016/j.renene.2018.03.006.
- Ahmadi Moghaddam E, Ahlgren S, Hulteberg C, et al. Energy balance and global warming potential of biogas-based fuels from a life cycle perspective. Fuel Process Technol. 2015;132:74–82. https://doi.org/10.1016/j.fuproc.2014.12.014.
- Hosseini SE, Wahid MA. Development of biogas combustion in combined heat and power generation. Renewable Sustainable Energy Rev. 2014;40:868–875.
- Lyng K-A, Brekke A. Environmental life cycle assessment of biogas as a fuel for transport compared with alternative fuels. Energies. 2019;12(3):532. https://doi.org/10.3390/en12030532.
- Salvador R, Barros MV, Rosário J, et al. Life cycle assessment of electricity from biogas: a systematic literature review. Environ Prog Sustainable Energy. 2019;38(4):13133. https://doi.org/10.1002/ep.13133.
- Chen S, Chen B, Song D. Life-cycle energy production and emissions mitigation by comprehensive biogas–digestate utilization. Bioresour Technol. 2012;114:357–364. https://doi.org/10.1016/j.biortech.2012.03.084.
- Yasar A, Nazir S, Tabinda AB, et al. Socio-economic, health and agriculture benefits of rural household biogas plants in energy scarce developing countries: a case study from Pakistan. Renewable Energy. 2017;108:19–25. https://doi.org/10.1016/j.renene.2017.02.044.
- Conti C, Mancusi ML, Sanna-Randaccio F, et al. Transition towards a green economy in Europe: innovation and knowledge integration in the renewable energy sector. Res Policy. 2018;47(10):1996–2009. https://doi.org/10.1016/j.respol.2018.07.007.
- McCarty T, Sesmero J. Uncertainty, irreversibility, and investment in second-generation biofuels. Bioenerg Res. 2015;8(2):675–687. https://doi.org/10.1007/s12155-014-9549-y.
- EurObserv’ER. Biogas barometer. 2017.
- EurObserv'ER. Biogas barometer. 2010.
- Kampman B, Leguijt C, Scholten T, et al. Optimal use of biogas from waste streams – an assessment of the potential of biogas from digestion in the EU beyond 2020. Brussels: European Commission; 2016.
- Danish Energy Agency. Perspektiver for produktion og anvendelse af biogas i Danmark [Perspectives on production and use of biogas in Denmark]. Copenhagen, Denmark: Energistyrelsen; 2018.
- French Ministry of Ecological and Solidarity Transition. Decree no. 2020-456 of April 21, 2020 relating to the multi-year energy programme (Décret no 2020-456 du 21 avril 2020 relatif à la programmation pluriannuelle de l’énergie) (no. TRER2006667D). J Officiel Répub Française. 2020:3.
- Westlund Å, Berggren R, Jacobsson R, et al. More biogas! For a sustainable Sweden (Mer biogas! För ett hållbart sverige) (no. SOU 2019:63). tockholm, Sweden: Biogasmarknadsutredningen; 2019.
- Huttunen S, Kivimaa P, Virkamäki V. The need for policy coherence to trigger a transition to biogas production. Environ Innovation Soc Transitions. 2014;12:14–30. https://doi.org/10.1016/j.eist.2014.04.002.
- Nilsson M, Zamparutti T, Petersen JE, et al. Understanding policy coherence: analytical framework and examples of Sector-Environment policy interactions in the EU: understanding policy coherence. Env Pol Gov. 2012;22(6):395–423. https://doi.org/10.1002/eet.1589.
- Hermann L, Hermann R. Report on regulations governing AD and NRR in EU member states SYSTEMIC, Grant Agreement no 730400; Wageningen, Netherlands: PROMAN Consulting; 2018.
- van Grinsven A, Leguijt C, Tallat-Kelpsaite J. Supporting mechanisms for the development of biomethane in transport (no. 17.3K49.31). Delft, Netherlands: CE Delft; 2017.
- Gustafsson M, Anderberg S. Dimensions and characteristics of biogas policies – modelling the European policy landscape. Renewable Sustainable Energy Rev. 2021;135:110200. https://doi.org/10.1016/j.rser.2020.110200.
- Rogstrand G. Overview on administrative and legal conditions as well as on financial and other support programs, for small to medium scale biomethane production and supply. Record Biomap, Grant Agreement no. 691911; The Research Institute of Sweden (RISE); 2018.
- European Commission. Commission proposes new EU framework to decarbonise gas markets, promote hydrogen and reduce methane emissions [WWW Document]. European Commission, Press corner; 2021a [cited 2021 Dec 20]. Available from https://ec.europa.eu/commission/presscorner/detail/en/IP_21_6682.
- European Commission. Communication from the Commission to the European Parliament, the Council and the European Economic and Social Committee of the Regions on an EU strategy to reduce methane emissions (no. COM(2020) 663). Brussels, Belgium: European Commission; 2020a.
- European Commission. Communication from the Commission to the European Parliament, the Council and the European Economic and Social Committee of the Regions – Powering a climate-neutral economy: an EU strategy for energy system integration (no. COM(2020) 299). Brussels, Belgium: European Commission; 2020b.
- European Commission, Communication from the Commission to the European Parliament, the Council and the European Economic and Social Committee of the Regions – A farm to fork strategy for a fair, healthy and environmentally-friendly food system (no. COM(2020) 381). Brussels, Belgium: European Commission; 2020c.
- European Commission. Communication from the Commission to the European Parliament, the Council and the European Economic and Social Committee of the Regions – REPowerEU: Joint European Action for more affordable, secure and sustainable energy (no. COM(2022) 108). Brussels, Belgium: European Commission; 2022a.
- Swedish Environmental Protection Agency. Biogas from manure, waste and residual products – Good Swedish examples (Biogas ur gödsel, avfall och restprodukter – Goda svenska exempel) (no. 6518). Stockholm, Sweden: Naturvårdsverket; 2012.
- Swedish Gas Association. Biogas in Sweden – English summary of the Swedish website Biogasportalen.se; 2011.
- Olsson L, Fallde M. Waste(d) potential: a socio-technical analysis of biogas production and use in Sweden. J Clean Prod. 2015;98:107–115. https://doi.org/10.1016/j.jclepro.2014.02.015.
- Ottosson M, Magnusson T, Andersson H. Shaping sustainable markets—a conceptual framework illustrated by the case of biogas in Sweden. Environ Innov Soc Transitions. 2020;36:303–320. https://doi.org/10.1016/j.eist.2019.10.008.
- Dahlgren S, Liljeblad A, Cerruto J, et al. Realisable biogas potential in Sweden by 2030 through digestion and gasification (Realiserbar biogaspotential i Sverige år 2030 genom rötning och förgasning). Stockholm, Sweden: WSP; 2013.
- Lönnqvist T. Biogas in Swedish transport – a policy-driven systemic transition. Stockholm: KTH Royal Institute of Technology; 2017.
- Prade T, Björnsson L, Lantz M, et al. Can domestic production of iLUC-free feedstock from arable land supply Sweden’s future demand for biofuels? J Land Use Sci. 2017;12(6):407–441. https://doi.org/10.1080/1747423X.2017.1398280.
- Gustafsson M, Anderberg S. Biogas policies and production development in Europe: a comparative analysis of eight countries. Biofuels. 2022;13(8):931–944.
- Klackenberg L. Production of biogas and digestate and their use in 2020 (Produktion av biogas och rötrester och dess användning år 2020). Eskilstuna, Sweden: Swedish Energy Agency (Energimyndigheten); 2021b.
- Klackenberg L. Biomethane in Sweden – market overview and policies. Stockholm, Sweden: Swedish Gas Association (Energigas Sverige); 2021a.
- Lönnqvist T, Grönkvist S, Sandberg T. Forest-derived methane in the Swedish transport sector: a closing window? Energy Policy. 2017;105:440–450. https://doi.org/10.1016/j.enpol.2017.03.003.
- Swedish Ministry of Infrastructure. Biogas production support (Stöd till produktion av biogas) [WWW Document]; 2022[cited 2022 Mar 28]. Available from: https://www.regeringen.se/pressmeddelanden/2022/03/stod-till-produktion-av-biogas/.
- Varho V, Tapio P. Combining the qualitative and quantitative with the Q2 scenario technique—the case of transport and climate. Technol Forecast Soc Change. 2013;80(4):611–630. https://doi.org/10.1016/j.techfore.2012.09.004.
- Wiek A, Binder C, Scholz RW. Functions of scenarios in transition processes. Futures. 2006;38(7):740–766. https://doi.org/10.1016/j.futures.2005.12.003.
- Rotmans J, Kemp R, van Asselt M. More evolution than revolution: transition management in public policy. Foresight. 2001;3(1):15–31. https://doi.org/10.1108/14636680110803003.
- Kahn H, Wiener AJ. The year 2000: a framework for speculation on the next thirty-three years. Polit Sci Quart. 1968;83(4):663. https://doi.org/10.2307/2146851.
- Meadows DH, Meadows DL, Randers J, et al. The limits to growth: a report for the club of rome’s project on the predicament of mankind. New York: Universe Books; 1972.
- Börjeson L, Höjer M, Dreborg K-H, et al. Scenario types and techniques: towards a user’s guide. Futures. 2006;38(7):723–739. https://doi.org/10.1016/j.futures.2005.12.002.
- Mahmoud M, Liu Y, Hartmann H, et al. A formal framework for scenario development in support of environmental decision-making. Environ Modell Software. 2009;24(7):798–808. https://doi.org/10.1016/j.envsoft.2008.11.010.
- Silva Oliveira A, Duarte de Barros M, de Carvalho Pereira F, et al. Prospective scenarios: a literature review on the Scopus database. Futures. 2018;100:20–33. https://doi.org/10.1016/j.futures.2018.03.005.
- Robinson JB. Energy backcasting a proposed method of policy analysis. Energy Policy. 1982;10(4):337–344. https://doi.org/10.1016/0301-4215(82)90048-9.
- Lyons G, Davidson C. Guidance for transport planning and policymaking in the face of an uncertain future. Transport Res Part A Policy Pract. 2016;88:104–116. https://doi.org/10.1016/j.tra.2016.03.012.
- Anderberg, S., Prieler, S., Olendrzynski, K., de Bruyn, S., editors. Old sins: industrial metabolism, heavy metal pollution, and environmental transition in Central Europe. Tokyo: United Nations University Press; 2000.
- Prieler S, Leskó AP, Anderberg S. Three scenarios for land-use change: a case study in Central Europe, research report/IIASA. Laxenburg: International Institute for Applied Systems Analysis; 1998.
- Auvinen H, Ruutu S, Tuominen A, et al. Process supporting strategic decision-making in systemic transitions. Technol Forecast Soc Change. 2015;94:97–114. https://doi.org/10.1016/j.techfore.2014.07.011.
- Geels FW, McMeekin A, Pfluger B. Socio-technical scenarios as a methodological tool to explore social and political feasibility in low-carbon transitions: bridging computer models and the multi-level perspective in UK electricity generation (2010–2050). Technol Forecast Soc Change. 2020;151:119258. https://doi.org/10.1016/j.techfore.2018.04.001.
- Söderholm P, Hildingsson R, Johansson B, et al. Governing the transition to low-carbon futures: a critical survey of energy scenarios for 2050. Futures. 2011;43(10):1105–1116. https://doi.org/10.1016/j.futures.2011.07.009.
- van Sluisveld MAE, Hof AF, Carrara S, et al. Aligning integrated assessment modelling with socio-technical transition insights: an application to low-carbon energy scenario analysis in Europe. Technol Forecast Soc Change. 2020;151:119177. https://doi.org/10.1016/j.techfore.2017.10.024.
- Hillman KM, Sandén BA. Exploring technology paths: the development of alternative transport fuels in Sweden 2007–2020. Technol Forecast Soc Change. 2008;75(8):1279–1302.
- Hofman PS, Elzen BE, Geels FW. Sociotechnical scenarios as a new policy tool to explore system innovations: co-evolution of technology and society in the Netherland’s electricity domain. Innovation. 2004;6(2):344–360. https://doi.org/10.5172/impp.2004.6.2.344.
- Turnheim B, Berkhout F, Geels F, et al. Evaluating sustainability transitions pathways: bridging analytical approaches to address governance challenges. Global Environ Change. 2015;35:239–253. https://doi.org/10.1016/j.gloenvcha.2015.08.010.
- Eden A. Bio-Methane support policy in France. Federal Ministry for the Environment. Berlin, Germany: Adelphi, Nature Conservation and Nuclear Safety (BMU); 2018.
- Metson GS, Feiz R, Quttineh N-H, et al. Optimizing transport to maximize nutrient recycling and green energy recovery. Resour Conserv Recycling X. 2020; 9–10:100049. https://doi.org/10.1016/j.rcrx.2021.100049.
- Lindfors A, Eklund M, Brunzell A, et al. Världens bästa biogassystem – Ett BRC innovationsprojekt. Linköping, Sweden: Swedish Biogas Research Center; 2022.
- European Commission. Communication from the Commission – Guidelines on state aid for climate, environmental protection and energy 2022 (no. C(2022) 481). Brussels, Belgium: European Commission; 2022b.
- Gustafsson M, Anderberg S, Fredriksson Möller B, et al. The institutional conditions of biogas (Biogasens institutionella villkor) (no. 2021:4). Linköping, Sweden: Swedish Biogas Research Center; 2021a.
- Gustafsson M, Svensson N, Eklund M, et al. Well-to-wheel climate performance of gas and electric vehicles in Europe. Transport Res Part D Transport Environ. 2021c;97:102911. https://doi.org/10.1016/j.trd.2021.102911.
- Gustafsson M, Svensson N, Eklund M, et al. Well-to-wheel greenhouse gas emissions of heavy-duty transports – influence of electricity carbon intensity. Transport Res Part D Transport Environ. 2021b;93:102757. https://doi.org/10.1016/j.trd.2021.102757.
- Prussi M, Yugo M, De Prada L, et al. JEC well-to-wheels report v5: Well-to-Wheels analysis of future automotive fuels and powertrains in the European context (no. EUR 30284 EN), JRC Technical reports. Luxembourg: JRC; 2020.
- Ahlgren S, Björnsson L, Prade T, et al. Biodrivmedel och markanvändning i Sverige. Miljö-och energisystem, LTH, Lunds universitet. Lund, Sweden; 2017.
- Björn A, Borgström Y, Ejlertsson J, et al. Biogas production in Swedish pulp and paper production – synthesis of opportunities and limitations and technical evaluation (Biogasproduktion inom svensk pappers- och massaproduktion – Syntes av möjligheter och begränsningar samt teknisk utvärdering). Linköping, Sweden: Linköping University, Scandinavian Biogas Fuels and Pöyry; 2016.
- Carlsson M, Uldal M. Substrathandbok för biogasproduktion (no. SGC 200). Malmö, Sweden: Svenskt Gastekniskt Center; 2009.
- Ekstrand E-M, Larsson M, Truong X-B, et al. Methane potentials of the Swedish pulp and paper industry – a screening of wastewater effluents. Appl Energy. 2013;112:507–517.
- Lindfors A, Gustafsson M, Anderberg S, et al. Developing biogas systems in Norrköping, Sweden: An industrial symbiosis intervention. J Clean Prod. 2020;277. https://doi.org/10.1016/j.jclepro.2020.122822
- Tufvesson LM, Lantz M, Börjesson P. Environmental performance of biogas produced from industrial residues including competition with animal feed – life-cycle calculations according to different methodologies and standards. J Clean Prod. 2013;53:214–223. https://doi.org/10.1016/j.jclepro.2013.04.005.
- Entesari N, Divsalar A, Tsotsis TT. Modeling and simulation of a reactive separation system for carbon capture and utilization in biogas streams. Chem Eng Process – Process Intensification. 2020;156:108093. https://doi.org/10.1016/j.cep.2020.108093.
- Voelklein MA, Rusmanis D, Murphy JD. Biological methanation: strategies for in-situ and ex-situ upgrading in anaerobic digestion. Appl Energy. 2019;235:1061–1071. https://doi.org/10.1016/j.apenergy.2018.11.006.
- EBA. European Biogas Association Statistical Report: 2019 European Overivew. Brussels, Belgium: EBA; 2020.
- Raven R, Gregersen KH. Biogas plants in Denmark: successes and setbacks. Renewable Sustainable Energy Rev. 2007;11(1):116–132. https://doi.org/10.1016/j.rser.2004.12.002.
- Dahlgren S, Kanda W, Anderberg S. Drivers for and barriers to biogas use in manufacturing, road transport and shipping: a demand-side perspective. Biofuels. 2022;13(2):1–12. https://doi.org/10.1080/17597269.2019.1657661.
- Lönnqvist T, Anderberg S, Ammenberg J, et al. Stimulating biogas in the transport sector in a Swedish region – an actor and policy analysis with supply side focus. Renewable Sustainable Energy Rev. 2019;113:109269. https://doi.org/10.1016/j.rser.2019.109269.
- Ammenberg J, Anderberg S, Lönnqvist T, et al. Biogas in the transport sector—actor and policy analysis focusing on the demand side in the Stockholm region. Resour Conserv Recycling. 2018;129:70–80. https://doi.org/10.1016/j.resconrec.2017.10.010.
- Xylia M, Silveira S. On the road to fossil-free public transport: the case of Swedish bus fleets. Energy Policy. 2017;100:397–412. https://doi.org/10.1016/j.enpol.2016.02.024.
- European Commission. European Green Deal: Commission proposes transformation of EU economy and society to meet climate ambitions [WWW Document]; 2021b [cited 2022 Jan 28]. Available from: https://ec.europa.eu/commission/presscorner/detail/en/ip_21_3541.
- Hunhammar S, Pucher M, Jernbäcker E, et al. In a world in transition – Sweden without fossil fuels 2040 (I en värld som ställer om – Sverige utan fossila drivmedel 2040) (no. SOU 2021:48). Stockholm, Sweden: Utfasningsutredningen; 2021.
- Wiborg E, Dyveke Søttar H, Johnsen TA, et al. Proposal to remove the governmental barriers for increased production and use of biogas (Innstilling om å fjerne de statlige barrierene for økt produksjon og bruk av biogass) (no. 8:231 S). Norway: Government of Norway (Stortinget); 2021.
- European Commission. Proposal for a regulation of the European Parliament and of the Council on the use of renewable and low-carbon fuels in Maritime transport and amending directive 2009/16/EC (no. COM(2021) 562). Brussels, Belgium: European Commission; 2021c.
- Ammenberg J, Gustafsson M, O’Shea R, et al. Perspectives on biomethane as a transport fuel within a circular economy, energy, and environmental system (no. 2021:12). Linköping, Sweden: (IEA Bioenergy Task 37); 2021.
- Lönnqvist T, Fagerström A, Feiz R, et al. Security of supply and circularity of transport biofuels – method development (no. FDOS 24:2022). Stockholm, Sweden: f3 The Swedish Knowledge Centre for Renewable Transportation Fuels & Swedish Energy Agency; 2022.
- Trading Economics. EU Natural Gas [WWW Document]; 2022 [cited 2022 Mar 3]. Available from: https://tradingeconomics.com/commodity/eu-natural-gas.
- Council of the EUE:\Praveen\TF-TBFU220054\12. Russia’s military aggression against Ukraine: EU imposes sanctions against President Putin and Foreign Minister Lavrov and adopts wide ranging individual and economic sanctions [WWW Document]; 2022 [cited 2022 Mar 3]. Available from: https://www.consilium.europa.eu/en/press/press-releases/2022/02/25/russia-s-military-aggression-against-ukraine-eu-imposes-sanctions-against-president-putin-and-foreign-minister-lavrov-and-adopts-wide-ranging-individual-and-economic-sanctions/?utm_source=dsms-auto&utm_medium=email&utm_campaign=Russia%u2019s+military+aggression+against+Ukraine%3a+EU+imposes+sanctions+against+President+Putin+and+Foreign+Minister+Lavrov+and+adopts+wide+ranging+individual+and+economic+sanctions.
- Van Driel H, Schot J. Radical innovation as a multilevel process: Introducing floating grain elevators in the port of Rotterdam. Technol Culture. 2005;46(1):51–76.
- Lindquist H. The journey of reinventing the European Electricity Landscape—challenges and pioneers. In: Lawrence EJ, editors. Renewable energy integration. Amsterdam: Elsevier; 2014. p. 3–12. https://doi.org/10.1016/B978-0-12-407910-6.00001-6
- Soeder DJ, Borglum SJ. Energy security, in: the fossil fuel revolution: shale gas and tight oil. Amsterdam: Elsevier; 2019. p. 251–277. https://doi.org/10.1016/B978-0-12-815397-0.00009-4
- Upham P, Eberhardt L, Klapper RG. Rethinking the meaning of “landscape shocks” in energy transitions: German social representations of the Fukushima nuclear accident. Energy Res Soc Sci. 2020;69:101710. https://doi.org/10.1016/j.erss.2020.101710.
- Kanda W, Kivimaa P. What opportunities could the COVID-19 outbreak offer for sustainability transitions research on electricity and mobility? Energy Res Soc Sci. 2020;68:101666. https://doi.org/10.1016/j.erss.2020.101666.
- Edwards J, Othman M, Burn S. A review of policy drivers and barriers for the use of anaerobic digestion in Europe, the United States and Australia. Renewable Sustainable Energy Rev. 2015;52:815–828. https://doi.org/10.1016/j.rser.2015.07.112.
- Lantz M, Svensson M, Björnsson L, et al. The prospects for an expansion of biogas systems in Sweden—incentives, barriers and potentials. Energy Policy. 2007;35(3):1830–1843. https://doi.org/10.1016/j.enpol.2006.05.017.
- Nevzorova T, Kutcherov V. Barriers to the wider implementation of biogas as a source of energy: a state-of-the-art review. Energy Strategy Rev. 2019;26:100414. https://doi.org/10.1016/j.esr.2019.100414.
Appendix A
Table A1. Division of substrates per type of biogas plant in 2020 [Citation51] and the three future scenarios.
TABLE A2. Biogas yield and production potential per substrate type.
Table A3. Typical properties (2020 average) of different types of biogas plants in Sweden.