Abstract
The occurrence of 17β-estradiol (E2) in the aquatic environment can lead to damage to the reproductive system, along with other adverse effects including oxidative stress, in aquatic organisms. In the present study, Perinereis aibuhitensis were treated with E2 at 0.1, 1, 10, 100 and 1000 μg/L for 205 d, after which the activities of superoxide dismutase (SOD), catalase (CAT) and glutathione-S-transferase (GST), and the concentrations of glutathione antioxidant biomarker responses were studied. Weight gain and specific growth rate of P. aibuhitensis were not significantly affected by E2 treatment. Although no significant differences in mortality were observed, the group receiving the highest dose of E2 (1000 μg/L) experienced the highest rate of mortality. Treatment with E2 enhanced the levels of total glutathione (T-GSH), but levels of glutathione reduced (GSH) were significantly decreased by 17.02, 20.55, 23.45, 31.91 and 56.08%, with a concomitant increase in the levels of glutathione disulphide (GSSG) by 148.58, 213.05, 232.62, 294.63 and 306.45%, at 0.1, 1, 10, 100 and 1000 μg/L E2, respectively. The redox ratio of GSH/GSSG was significantly decreased (p < 0.01). The results of this study suggest that long-term exposure of P. aibuhitensis to E2 may inhibit antioxidant enzyme activities, thereby reducing the capacity of its detoxification system.
Introduction
17β-estradiol (E2) is the major oestrogen secreted by humans, and is an emerging contaminant that can reach the aquatic environment via wastewater release, livestock waste and agricultural runoff. The occurrence of E2 in wastewater and surface water has been investigated in numerous studies (Servos et al. Citation2005; Coleman et al. Citation2010; Yan et al. Citation2012), thus presenting a potential hazard for aquatic species (Rotchell & Ostrander Citation2003; Caldwell et al. Citation2012). The reported effects of E2 on aquatic species have mainly focused on the endocrine disrupting effects and the mechanisms of action (Osada et al. Citation2003; Maria et al. Citation2008; Ciocan et al. Citation2010). However, some studies have demonstrated that E2 can disrupt non-reproductive endocrine events, namely the stress responses in fish (Teles et al. Citation2005; Maria et al. Citation2008; Moura Costa et al. Citation2010). The immune system of molluscs also represents a significant target for the action of environmental estrogens, as E2 in the nM range (1–100 nM) induces lysosomal destabilisation in Mytilus (Canesi et al. Citation2007). Exposure to E2 can also disturb the intra-cellular environment, increasing the production of reactive oxygen species (ROS), thereby impairing the antioxidant defence system (Maria et al. Citation2008).
The activities of antioxidant enzymes such as superoxide dismutase (SOD, converts O2- to H2O2) and catalase (CAT, converts H2O2 to water) are important biomarkers for investigating the cellular redox system. Glutathione is a major endogenous antioxidant, which plays a crucial role in protecting cells from exogenous and endogenous toxins, and exists in the glutathione reduced (GSH) and glutathione disulphide (GSSG). GSH is the most important endogenous antioxidant for detoxification and elimination of environmental toxins and free radicals, such as ROS that disrupt cellular functions by oxidizing lipids, proteins, and DNA. Similar to SOD and CAT, glutathione-S-transferase (GST) catalyzes the conjugation of reduced glutathione with a variety of xenobiotics via cysteine thiol as a phase II detoxifying enzyme, protecting cells against chemical insults. The post-translational redox modifications of proteins probably depend on the magnitude of the oxidant stimulus and the duration of exposure. A high dose or chronic exposure may lead to irreversible post-translational modification and protein degradation. Such events could damage to biomolecules, cell death and altered homeostasis. Cancer, diabetes, atherosclerosis, neurodegenerative disorders and the aging process have been associated with oxidative stress (OS) due to elevated ROS or to insufficient ROS detoxification in humans (Limón-Pacheco & Gonsebatt Citation2009).
Polychaetes are usually the most abundant taxon in marine benthic communities, are an important source of food for many shore birds and oceanic fishes, and have also been frequently utilised as indicator species in environmental assessments (Dean Citation2008). The activities of GST, CAT, and SOD have been used as biomarkers in environmental field surveys employing polychaetes, including species such as Nereis diversicolor (Durou et al. Citation2007; Bouraoui et al. Citation2010), Perinereis nuntia (Won et al. Citation2011) and Neanthes succinea (Rhee et al. Citation2011). Many polychaete species demonstrate a relatively high capacity to regulate organic contaminants and heavy metals, and are regarded as good bioindicators of metal and organic contamination in estuaries (Dean Citation2008). Despite this frequent use of Nereidids worms for environmental assessment and an understanding of their antioxidant defense systems, knowledge concerning the genotoxic and lipid peroxidative effects of E2 in these animals is scarce.
In the present research, the clam worm P. aibuhitensis was selected as a model organism to evaluate the toxic effects of chronic exposure to E2, and verify the dose-dependent physiological effects of this hormone using biomarkers that cover the cellular redox system. These biomarkers include CAT, SOD and GST enzyme activity, the concentrations of GSH, GSSG and the redox ratio of GSH to GSSG.
Materials and methods
Chemicals
17β-estradiol (analytical standard, 99.6%; CAS. no. 50-28-2) was purchased from Alfa Aesar (China). Stock solutions were prepared by dissolving E2 in acetone and further diluting with artificial seawater (25‰).
Experimental design
Juvenile P. aibuhitensis (8.8 ± 1.2 mg), collected from a breeding colony in our laboratory, were divided into 21 different groups of 15 individuals and placed into with a 10 cm pebble bed glass tanks (20 cm × 20 cm × 40 cm), and containing 1000 mL artificial seawater enriched with different E2 concentrations. Five nominal concentrations of E2 were used in the experiment: 0.1, 1, 10, 100 and 1000 μg/L. A negative control and a solvent control (0.01% [v/v] acetone, the same as the 1000 μg/L E2 exposure) were also included. There were three replicate tanks for each experimental group.
Every evening, all seawater was drained from the tanks and fish powder (purchase from feed factory) was fed. Tanks were supplied with 1000 mL of freshly prepared seawater containing E2 the next morning. Interstitial water quality parameters and ranges for the toxicity tests were as follows: water temperature 4–23 °C, pH 7.1 ± 0.2 and salinity 24 ppt. The experimental period was 205 d, starting in October and lasting until April of the following year. Weight gain ratio [(final body weight − initial body weight)/Initial body weight] and specific growth rate [((ln (final body weight) − ln (initial body weight))/205 d) × 100] were calculated at the end of the experiment.
Biochemical assays
The concentrations of glutathione (μmol/L) and total protein, and the activities of GST (U/mg protein), CAT (U/mg protein) and SOD (U/mg protein), were determined using the Diagnostic Reagent Kits purchased from Nanjing Jiancheng Bioengineering Institute (PR China). The level of GSH was calculated as tGSH–2GSSG, and the redox ratio of GSH/GSSG was calculated as (GSH/GSSG) × 100.
Statistical analysis
All data are presented as mean ± SE. The concentrations of glutathione, SOD activity, CAT activity and GST activity were evaluated by one-way analysis of variance followed by least significant difference multiple comparisons using the statistical package SPSS 16.0 (SPSS Inc., Chicago, IL). Results were considered significantly different when p < 0.05.
Results and discussion
Effects of 17β-estradiol on growth, superoxide dismutase, catalase and glutathione-S-transferase activities
After 205 d, no clam worm mortality was observed in the 1 or 100 μg/L E2 treatment groups. The highest rate of mortality (seven worms, 13.33%) was observed in the 1000 μg/L E2 group. The weight gain and specific growth rate of P. aibuhitensis were not significantly affected by E2 (Table ).
Table 1. Effect of 17β-estradiol on the growth and survival rate of Perinereis aibuhitensis (mean ± SE).
Antioxidant enzyme activities (CAT, SOD and GST) of P. aibuhitensis were significantly affected by E2 exposure. CAT activity being significantly lower in all E2-treated P. aibuhitensis than in the control (Figure ). The CAT activities of P. aibuhitensis treated with 100 μg/L and 1000 μg/L E2 were lower than that of the control by 56.50% and 67.05%, respectively (p < 0.05), and 1000 μg/L E2 treatment group was also significantly lower than other E2 treatment groups (p < 0.05). The activity of SOD was also significantly decreased at all concentrations of E2 compared with control (p < 0.05), and 100 μg/L and 1000 μg/L E2 treatment groups were significantly lower than other E2 treatment groups (p < 0.05) (Figure ). A significant decrease was also observed in GST activity in all E2 treatment groups compared with control (p < 0.05), and 1000 μg/L E2 treatment group was lower than other E2 treatment groups (p < 0.05) (Figure ).
Figure 1. Effect of chronic 17β-estradiol exposure on Perinereis aibuhitensis antioxidant enzyme activities: (a) catalase (CAT) activity, (b) superoxide dismutase (SOD) activity and (c) glutathione-S-transferase (GST) activity (different letters indicates significant difference between groups, p < 0.05).
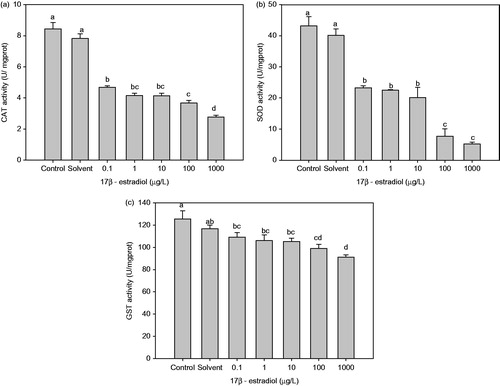
ROS are continually produced as undesirable toxic by-products of various endogenous metabolic processes in aerobic organisms (Livingstone Citation2003). Some studies suggest that exposure to natural estrogens and xenoestrogens increases intracellular ROS levels, which induces damage to nucleic acids, proteins, carbohydrates and lipids, thereby altering the functions of these macromolecules in cells (Filby et al. Citation2007; Rempel-Hester et al. Citation2009; Koutsogiannaki et al. Citation2014). Treatment of Mytilus galloprovincialis hemocytes with 25 nM E2 for 30 min caused a significant increase in ROS production, which led to oxidative damage exemplified by a significant increase in DNA damage, protein carbonylation and lipid peroxidation (Koutsogiannaki et al. Citation2014). Damage of DNA was also increased in the gonads of male hornyhead turbot after a 48 h exposure to 15 mg/L E2 (Rempel-Hester et al. Citation2009). Similar DNA damage was also observed in the tissue of fish exposed to estrogenic wastewaters for 21 d (Filby et al. Citation2007).
The ROS produced in biological systems are detoxified and purportedly held in check by antioxidant defences. The antioxidant enzymes, including CAT, SOD and GST, are widely found in aquatic organisms and are part of the defense system that prevents cellular damage by ROS. A time- and dose-dependent inhibition of GST activity has been reported in fish (Dicentrarchus labrax) exposed to E2 (Vaccaro et al. Citation2005). Hepatic activities of GST and CAT were also significantly decreased in sea bream (Sparus aurata) after E2 administration (Carrera et al. Citation2007). The activities of CAT and SOD were also slightly decreased in EE2 injected common carp (Cyprinus carpio) (Solé et al. Citation2000). In the present study, the weight gain of P. aibuhitensis was not significantly affected by exposure to E2. The rate of mortality was increased in the 1000 μg/L E2 treatment group, although this increase was not significantly different from the control. The decline in the activities of P. aibuhitensis antioxidant enzymes, including CAT, SOD and GST, suggests an impairment of the antioxidant defence system following exposure to E2. Under these conditions, the production of reactive ROS may exceed the capacity of cellular antioxidant defences to remove this toxic species, resulting in oxidative stress.
Effects of 17β-estradiol on total glutathione and glutathione disulphide levels
Treatment with E2 enhanced the levels of total GSH (T-GSH) in comparison with the control. However, GSH levels were significantly decreased by 17.02, 20.55, 23.45, 31.91 and 56.08% (Figure ), with a concomitant increase in the levels of GSSG by 1.49, 2.13, 2.32, 2.95 and 3.06 times (Figure ), at 0.1, 1, 10, 100 and 1000 μg/L E2, respectively, as compared with the control group. Treated with 100 and 1000 μg/L E2 also significantly increased the levels of GSSG compared with other E2 treatment groups. The redox ratio of GSH/GSSG was significantly decreased (p < 0.05) (Figure ).
Figure 2. Effect of chronic 17β-estradiol exposure on the concentrations of glutathione in Perinereis aibuhitensis: (a) GSH (glutathione reduced), (b) GSSG (glutathione disulphide), and (c) the ratio of GSH to GSSG (different letters indicates significant difference between groups, p < 0.05).
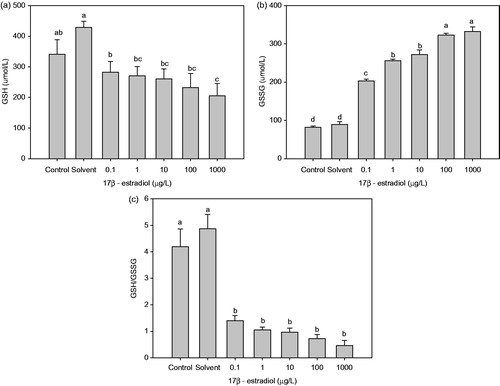
The effects of estrogens on the immune systems of Nereidids worms are still unclear. Some studies suggest that E2 can affect immune function in fish, resulting in changes that include leukocyte production and activity (Yamaguchi et al. Citation2001; Moura Costa et al. Citation2010). As a major intracellular antioxidant, GSH plays a crucial role in the maintenance and regulation of the thiol–redox status of the cell, which prevents the oxidation of protein thiol groups, either directly by reacting with reactive species or indirectly through glutathione transferases. Low intracellular GSH levels would decrease the cellular antioxidant capacity. Several reports have suggested that a decrease in GSH levels is also associated with immune system dysfunction and inflammation in humans (Ballatori et al. Citation2009; Ghezzi Citation2011). In the present study, the depletion of GSH and the elevation of GSSG could result in damage to the immune system, which would decrease the resistance to disease. The death of P. aibuhitensis might also be the result of immune system dysfunction following long-term exposure to E2.
Nonylphenol (NP) exposure resulted in a depletion of GSH in Atlantic cod, while increasing GST activities, leading the author to suggest that the GSH depletion may be due to a phase II-mediated conjugation of nonylphenol with GSH by GST (Sturve et al. Citation2006). Induction of GST activity was also observed following NP treatment in the polychaete Nereis succinea (Ayoola et al. Citation2011). In studies in which GST enzyme activity was used as a biomarker in polychaetes exposed to various heavy metals and/or metal polluted environments, enzyme activity either increased or decreased with different patterns according to the elements studied or the exposure conditions (Mosleh et al. Citation2006; Won et al. Citation2011). In the present study, GSH decreased after exposure to E2, while GST activity did not increase. Further studies are needed to understand the synthesis, consumption, and/or regeneration of GSH in P. aibuhitensis. In addition, the decrease in the activities of SOD, GST and CAT, and the depletion after E2 administration, indicates a decrease in the xenobiotic transformation metabolism in P. aibuhitensis. This is similar to what has been observed in Sparus aurata injected with E2, in which EROD, GST and CAT activities were depleted (Carrera et al. Citation2007).
Conclusions
Chronic exposure to E2 did not affect the weight gain or specific growth rate of P. aibuhitensis. However, the activities of P. aibuhitensis antioxidant enzymes (CAT, SOD and GST) were decreased after exposure to E2, which may indicate a decreased capacity of the cellular antioxidant system. Treatment with E2 also resulted in decreased levels of GSH and GSH/GSSG, which could potentially lead to damage of the immune system, and a reduced resistance to disease.
Funding information
This work was supported by the Natural Science Foundation of Jiangsu Province of China (BK2012675), and Jiangsu Province innovation and entrepreneurship PhD plan (2014).
Disclosure statement
The authors report no conflicts of interest. The authors alone are responsible for the content and writing of this article.
References
- Ayoola JA, García-Alonso J, Hardege JD. 2011. Glutathione-S-transferase in Nereis succinea (Polychaeta) and its induction by xeno-estrogen. Environ Toxicol. 26:559–565.
- Ballatori N, Krance SM, Notenboom S, Shi S, Tieu K, Hammond CL. 2009. Glutathione dysregulation and the etiology and progression of human diseases. Biol Chem. 390:191–214.
- Bouraoui Z, Banni M, Chouba L, Ghedira J, Clerandeau C, Jebali J, Narbonne JF, Hmamadi B. 2010. Monitoring pollution in Tunisian coasts using a scale of classification based on biochemical markers in worms Nereis (Hediste) diversicolor. Environ Monit Assess. 164:691–700.
- Caldwell DJ, Mastrocco F, Anderson PD, Lange R, Sumpter JP. 2012. Predicted-no-effect concentrations for the steroid estrogens estrone, 17β-estradiol, estriol, and 17α-ethinylestradiol. Environ Toxicol Chem. 31:1396–1406.
- Canesi L, Lorusso LC, Ciacci C, Betti M, Rocchi M, Pojana G, Marcomini A. 2007. Immunomodulation of Mytilus hemocytes by individual estrogenic chemicals and environmentally relevant mixtures of estrogens: in vitro and in vivo studies. Aquat Toxicol. 81:36–44.
- Carrera EP, García-López A, del Pilar Martín del Río M, Martínez-Rodríguez G, Solé M, Miguel Mancera J. 2007. Effects of 17β-estradiol and 4-nonylphenol on osmoregulation and hepatic enzymes in gilthead sea bream (Sparus auratus). Comp Biochem Physiol C. 145:210–217.
- Ciocan CM, Cubero-Leon E, Puinean AM, Hill EM, Minier C, Osada M, Fenlon K, Rotchell JM. 2010. Effects of estrogen exposure in mussels, Mytilus edulis, at different stages of gametogenesis. Environ Pollut. 158:2977–2984.
- Coleman HM, Le-Minh N, Khan SJ, Short MD, Chernicharo C, Stuetz RM. 2010. Fate and levels of steroid oestrogens and androgens in waste stabilisation ponds: quantification by liquid chromatography-tandem mass spectrometry. Water Sci Technol. 61:677–684.
- Dean HK. 2008. The use of polychaetes (Annelida) as indicator species of marine pollution: a review. Rev Biol Trop. 56:11–38.
- Durou C, Poirier L, Amiard JC, Budzinski H, Gnassia-Barelli M, Lemenach M, Peluhet L, Mouneyrac C, Roméo M, Amiard-Triquet C. 2007. Biomonitoring in a clean and a multi-contaminated estuary based on biomarkers and chemical analyses in the endobenthic worm Nereis diversicolor. Environ Pollut. 148:445–458.
- Filby AL, Neuparth T, Thorpe KL, Owen R, Galloway TS, Tyler CR. 2007. Health impacts of estrogens in the environment, considering complex mixture effects. Environ Health Persp. 115:1704–1710.
- Ghezzi P. 2011. Role of glutathione in immunity and inflammation in the lung. Int J Gen Med. 4:105–113.
- Koutsogiannaki S, Franzellitti S, Fabbri E, Kaloyianni M. 2014. Oxidative stress parameters induced by exposure to either cadmium or 17β-estradiol on Mytilus galloprovincialis hemocytes. The role of signaling molecules. Aquat Toxicol. 146:186–195.
- Limón-Pacheco J, Gonsebatt ME. 2009. The role of antioxidants and antioxidant-related enzymes in protective responses to environmentally induced oxidative stress. Mutat Res-Gen Tox En. 674:137–147.
- Livingstone D. 2003. Oxidative stress in aquatic organisms in relation to pollution and aquaculture. Rev Med Vet. 154:427–430.
- Maria VL, Ahamad I, Santos MA. 2008. Juvenile sea bass (Dicentrarchus labrax L.) DNA strand breaks and lipid peroxidation response following 17β-estradiol two mode of exposures. Environ Int. 34:23–29.
- Mosleh YY, Paris-Palacios S, Biagianti-Risbourg S. 2006. Metallothioneins induction and antioxidative response in aquatic worms Tubifex tubifex (Oligochaeta, Tubificidae) exposed to copper. Chemosphere 64:121–128.
- Moura Costa DD, Filipak Neto F, Costa MDM, Morais RN, Garcia JRE, Esquivel BM, Oliveira Ribeiro CA. 2010. Vitellogenesis and other physiological responses induced by 17-β-estradiol in males of freshwater fish Rhamdia quelen. Comp Biochem Phys C. 151:248–257.
- Osada M, Takamura T, Sato H, Mori K. 2003. Vitellogenin synthesis in the ovary of scallop, Patinopecten yessoensis: control by estradiol-17β and the central nervous system. J Exp Zoo. 299:172–179.
- Rhee JS, Won EJ, Kim RO, Lee J, Shin KH, Lee JS. 2011. Expression of superoxide dismutase (SOD) genes from the copper-exposed polychaete, Neanthes succinea. Mar Pollut Bull. 63:277–286.
- Rempel-Hester MA, Hong HZ, Wang YS, Deng X, Armstrong J, Gully J, Schlenk D. 2009. Site-specific effects of 17β-estradiol in hornyhead turbot (Pleuronichthys verticalis) collected from a wastewater outfall and reference location. Environ Res. 109:552–558.
- Rotchell J, Ostrander G. 2003. Molecular markers of endocrine disruption in aquatic organisms. J Toxicol Environ Health B Crit Rev. 6:453–496.
- Servos MR, Bennie DT, Burnison BK, Jurkovic A, McInnis R, Neheli T, Schnell A, Seto P, Smyth SA, Ternes TA. 2005. Distribution of estrogens, 17beta-estradiol and estrone, in Canadian municipal wastewater treatment plants. Sci Total Environ. 336:155–170.
- Solé M, Porte C, Barcelo D. 2000. Vitellogenin induction and other biochemical responses in carp, Cyprinus carpio, after experimental injection with 17α-ethynylestradiol. Arch Environ Con Toxi. 38:494–500.
- Sturve J, Hasselberg L, Fälth H, Celander M, Förlin L. 2006. Effects of North Sea oil and alkylphenols on biomarker responses in juvenile Atlantic cod (Gadus morhua). Aquat Toxicol. 78:S73–S78.
- Teles M, Pacheco M, Santos MA. 2005. Sparus aurata L. liver EROD and GST activities, plasma cortisol, lactate, glucose and erythrocytic nuclear anomalies following short-term exposure either to 17beta-estradiol (E2) or E2 combined with 4-nonylphenol. Sci Total Environ. 336:57–69.
- Vaccaro E, Meucci V, Intorre L, Soldani G, Di Bello D, Longo V, Gervasi PG, Pretti C. 2005. Effects of 17β-estradiol, 4-nonylphenol and PCB 126 on the estrogenic activity and phase 1 and 2 biotransformation enzymes in male sea bass (Dicentrarchus labrax). Aquat Toxicol. 75:293–305.
- Won EJ, Kim RO, Rhee JS, Park GS, Lee J, Shin KH, Lee YM, Lee JS. 2011. Response of glutathione-S-transferase (GST) genes to cadmium exposure in the marine pollution indicator worm, Perinereis nuntia. Comp Biochem Physiol C: Toxicol Pharmacol. 154:82–92.
- Yamaguchi T, Watanuki H, Sakai M. 2001. Effects of estradiol, progesterone and testosterone on the function of carp, Cyprinus carpio, phagocytes in vitro. Comp Biochem Physiol C. 129:49–55.
- Yan ZH, Lu GH, Liu JC, Jin SG. 2012. An integrated assessment of estrogenic contamination and feminization risk in fish in Taihu Lake, China. Ecotoxicol Environ Saf. 84:334–340.