Abstract
The aim of this study was to investigate the effect of milking dairy cows using conventional milking parlour (CMP) and automatic milking system (AMS) on milk coagulation properties and fatty acid (FA) composition. Milk coagulation traits were rennet coagulation time, curd-firming time and curd firmness. Data consisted of 10,476 individual milk samples collected from 918 Holstein–Friesian cows in 8 herds: four herds milked cows using a CMP and four using an AMS. A linear mixed model was used to investigate sources of variation for milk yield, traditional quality traits, coagulation properties and FA profile. On average, cows from AMS produced 1 kg/d more milk than cows from CMP. Rennet coagulation time was slightly longer (+1.2 min) and free FA content was greater (+0.16 mmol/100 g milk fat) in milk from cows milked in AMS than CMP. Overall, the milking system did not affect the FA concentration of milk. Results of the present study suggest that AMS can be adopted without detrimental effects on milk features.
Introduction
Automatic milking systems (AMS) have been available in Europe since the beginning of 1990s. The major advantages of AMS are the reduction of labour for milking (Dijkhuizen et al. Citation1997) and the enhanced production per cow due to higher milking frequency than conventional milking parlour (CMP) (Klei et al. Citation1997). Milk yield increases from 2% to 8% (Svennersten-Sjaunja & Pettersson Citation2008) and labour decreases by about 18%.
The effect of AMS on milk quality traits is negligible (Svennersten-Sjaunja & Pettersson Citation2008), whereas increasing milking frequency increases free fatty acids (FFA) content (Klei et al. Citation1997; Klungel et al. Citation2000; Wiking et al. Citation2006), which has a negative impact on milk flavour (Shipe et al. Citation1980) and cheese-making process (Sapru et al. Citation1997). The effect of AMS on somatic cell count (SCC) is contradictory; several authors reported an increase in SCC after the introduction of AMS (Kaartinen et al. Citation1990; Rasmussen et al. Citation2002), whereas other studies reported no effect of AMS on SCC (Klungel et al. Citation2000; Berglund et al. Citation2002; Mollenhorst et al. Citation2011).
Italy has a long tradition in producing high-quality cheeses and thus milk coagulation properties (MCP), protein composition, acidity and mineral composition are crucial to improve profitability of the dairy industry. Milk coagulation properties have been widely investigated (Pretto et al. Citation2013; Penasa et al. Citation2014) and proposed as innovative technological traits to enhance the efficiency of the dairy sector (De Marchi et al. Citation2013; Tiezzi et al. Citation2013). Moreover, mid-infrared spectroscopy (MIRS) prediction models have been implemented in several Italian milk quality laboratories to routinely predict MCP (De Marchi et al. Citation2014). The accuracies of these prediction models implemented by Foss (Foss Electric A/S, Hillerød, Denmark) were reported by Penasa et al. (Citation2014) and Penasa et al. (Citation2015) for MCP and fatty acid (FA) composition, respectively.
Although several researches have been focussed on the effect of AMS or milking frequency on milk chemical composition and SCC, very limited information is available on the effect of AMS on milk technological traits. Abeni et al. (Citation2003) and Innocente and Biasutti (Citation2013) did not find any significant differences for technological properties between milk derived from AMS and CMP. The aim of this study was to investigate the effect of CMP and AMS on MCP and FA composition, as predicted by MIRS, in Holstein–Friesian (HF) dairy cows.
Materials and methods
Data and editing
Individual milk samples from HF cows were collected between September 2011 and July 2014, as part of the official monthly milk recording, by the Breeders Association of Veneto Region (Padova). Samples were from 8 dairy herds located across the Veneto Region (northeast Italy). Cows were milked in 4 herds using a CMP and in 4 other using an AMS. Herds selected for this study were commercial farms of comparable size and their management, feeding conditions and production area were similar. Briefly, cows were reared in indoor barn with access to exercise paddock and diet was based on corn silage (no grazing pasture) and concentrates. Farms with AMS had DeLaval (VMS, DeLaval, Tumba, Sweden) group milking system equipped with Milko-Scope MKII for milk sampling.
Samples were processed according to International Committee for Animal Recording (ICAR Citation2014) procedures, combined with preservative immediately after collection (Bronopol; Knoll Pharmaceuticals, Nottingham, UK) and analysed in the laboratory of the Breeders Association of Veneto Region (Padova, Italy). Traditional milk quality traits (protein, fat, casein and lactose percentages), and pH, MCP and FA composition were assessed using Milko-Scan FT6000 (Foss Electric A/S, Hillerød, Denmark). Prediction models were implemented for routine prediction of MCP, FFA and FA composition, as reported by Tiezzi et al. (Citation2013) and Penasa et al. (Citation2015).
A production record consisted of cow identity code and parity, dates of calving and sampling, herd code and the following traits: milk yield (MY, kg/d), fat percentage (FP, %), protein percentage (PP, %), casein percentage (CP, %), lactose percentage (LP, %), SCC (n/mL), pH, rennet coagulation time (RCT, min), curd-firming time (k20, min), curd firmness (a30, mm), saturated FA (SFA, g/100 g milk), unsaturated FA (UFA, g/100 g milk), monounsaturated FA (MUFA, g/100 g milk), polyunsaturated FA (PUFA, g/100 g milk), FFA (mmol/100 g milk fat) and four single FA, namely C14:0, C16:0, C18:0 and the sum of C18:1 (g/100 g milk). Somatic cell count was log-transformed to somatic cell score (SCS) using the following formula: SCS =3 + log2(SCC/100,000).
Cows were retained if they were from parity 1 to 9 and from 5 to 480 days in milk. Moreover, lactations with less than 3 tests and herd-test-date (HTD) with less than 3 cows were removed from the dataset. After editing procedure, 10,476 records from 918 cows were available for statistical analysis. The average herd size during the period of the study was 115 (range: 81–179), and the average number of records per cow and HTD was 11 (range: 3–27) and 53 (range: 21–102), respectively.
Statistical analysis
A preliminary analysis indicated that all studied variables were normally distributed and thus a linear mixed model (SAS Institute Inc., Cary, NC) was used to investigate sources of variation for MY, quality traits, MCP and FA composition:
where yijklm is the dependent variable, μ is the overall intercept of the model, MSi is the fixed effect of the ith milking system (i = CMP, AMS), PARj is the fixed effect of the jth parity of the cow (j = first, second, third, fourth and fifth and later parities), DIMk is the fixed effect of the kth class of stage of lactation of the cow (k = 1–12, the first being a class from 5 to 35 d, followed by 9 classes of 30 d each, and the last two being classes of 45 and 130 d, respectively), (MS × PAR)ij is the fixed interaction effect between milking system and parity, (MS × DIM)ik is the fixed interaction effect between milking system and DIM, (PAR × DIM)jk is the fixed interaction effect between parity and DIM, HTDl(MSi) is the random effect of the lth herd-test-date (l = 1–197) nested within the ith milking system ∼N(0,σ2HTD(MS)), cowm is the random effect of the mth cow (m = 1–918) ∼N(0,σ2cow), and eijklm is the random residual ∼N(0,σ2e). Significance of milking system effect was tested on the herd-test-date within milking system variance. A multiple comparison of means was performed for the main effect of milking system, and for interactions between milking system and days in milk, and milking system and parity using Bonferroni’s correction (p < .05).
Results
Descriptive statistics
Milk yield, FP, PP, CP and SCS averaged 30.2 kg/d, 3.78%, 3.38%, 2.64% and 2.84, respectively. Means of RCT, k20 and a30 were 22.9 min, 6.80 min, and 23.1 mm, and exhibited coefficients of variation of 26, 32 and 42%, respectively (data not shown). Saturated FA, UFA, MUFA and PUFA averaged 2.71, 1.18, 0.93 and 0.06 g/100 g milk, respectively. Among individual FA, C16:0 was the most important (1.17 g/100 g milk), followed by the sum of C18:1 (0.73 g/100 g milk), C14:0 (0.41 g/100 g milk) and C18:0 (0.34 g/100 g milk). Free FA averaged 0.57 mmol/100 g milk fat (data not shown), which is very close to the value (0.50 mmol/100 g milk fat) considered normal for fresh milk.
Significance of fixed effects
The significance of fixed effects included in the statistical analysis is reported in Table . Stage of lactation was the most important factor (p < .001) in explaining part of the variation of all studied traits, followed by parity, which was relevant for most variables, except for FP, SFA, UFA, C16:0, C18:0 and FFA. Milking system significantly affected (p < .05) MY, pH, RCT, C16:0 and FFA. Among interaction effects, parity × stage of lactation was the most important to explain the variability of analysed milk characteristics, except for fat, SFA, C14:0, C16:0 and FFA. Milking system × stage of lactation effect had a strong influence (p < .05) on MY, PP, CP, pH, a30 and FFA. Finally, milking system × parity effect was important (p < .05) for MY, PP, CP and SCS (Table ).
Table 1. p-ValueTable Footnotea of fixed effectsTable Footnoteb included in the statistical analysis of milk yield, quality traits, coagulation properties and fatty acid composition, and least squares means (standard errors within parentheses) of the studied traits for milking system effectTable Footnotec.
Cows milked by AMS produced more milk (+1 kg/d; p < .05) compared with those milked by CMP (Table ). Milk from cows milked by AMS had greater but not significant SCS (3.17 vs. 3.03), slightly higher pH (6.64 vs. 6.63; p < .05) and slightly longer RCT (23.9 vs. 22.7 min; p < .05) than milk from CMP. Regarding FA composition, only C16:0 and FFA were significantly (p < .05) different between AMS and CMP (Table ). In particular, milk FFA content was greater for AMS than CMP (0.64 vs. 0.48 mmol/100 g milk fat; p < .05). Milk yield across parities was greater for cows from AMS than CMP (Table ) even if significance was obtained only for animals in third (32.4 vs. 31.3 kg/d; p < .05) and fifth, and later lactations (31.7 vs. 29.1 kg/d; p < .05).
Table 2. Least squares means (standard errors within brackets) of milk yield, kg/d, for the interaction effect between milking systemTable Footnotea and parityTable Footnoteb.
The trend of MY, protein and a30 across lactation and milking system is depicted in Figures , respectively. Significant differences between AMS and CMP were detected until 170 days in milk, where cows milked by AMS yielded more milk than those milked with CMP. The most pronounced differences between the two milking systems were around the peak of lactation (Figure ). Concerning protein percentage, no statistical differences between AMS and CMP were detected across days in milk, with the exception of the beginning and end of lactation where milk of cows milked with AMS had greater and lower protein percentage than milk of cows milked with CMP, respectively (Figure ). Overall, cows milked by CMP produced milk with better a30 compared with AMS but significance was found only at the end of lactation (Figure ). The difference between AMS and CMP for FFA across days in milk is presented in Figure . Cows milked with AMS produced milk with greater FFA content across lactation. The greatest difference between AMS and CMP was detected within the first 80 days after calving, whereas the smallest differences were observed after 260 days in milk.
Figure 1. Least squares means of milk yield for the interaction effect between milking system (AMS: automatic milking system; CMP: conventional milking parlour) and days in milk (DIM). Significance of the differences between least squares means of AMS and CMP within each class of DIM is reported above the solid line. ns, not significant; *p < .05; **p < .01; ***p < .001.
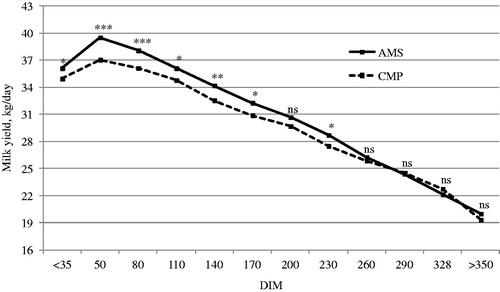
Figure 2. Least squares means of protein percentage for the interaction effect between milking system (AMS: automatic milking system; CMP: conventional milking parlour) and days in milk (DIM). Significance of the differences between least squares means of AMS and CMP within each class of DIM is reported above the solid line. ns, not significant; *p < .05.
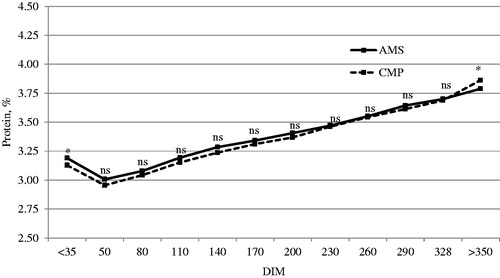
Figure 3. Least squares means of curd firmness (a30) for the interaction effect between milking system (AMS: automatic milking system; CMP: conventional milking parlour) and days in milk (DIM). Significance of the differences between least squares means of AMS and CMP within each class of DIM is reported above the dashed line. ns, not significant; **p < .01.
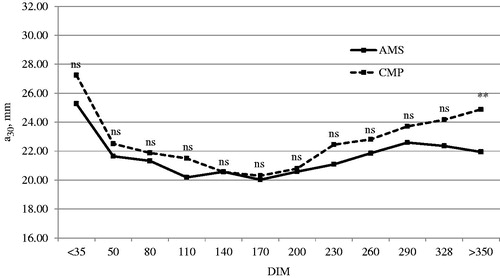
Figure 4. Least squares means of free fatty acids (FFA) for the interaction effect between milking system (AMS: automatic milking system; CMP: conventional milking parlour) and days in milk (DIM). Significance of the differences between least squares means of AMS and CMP within each class of DIM is reported above the solid line. ns, not significant; *p < .05; ***p < .001.
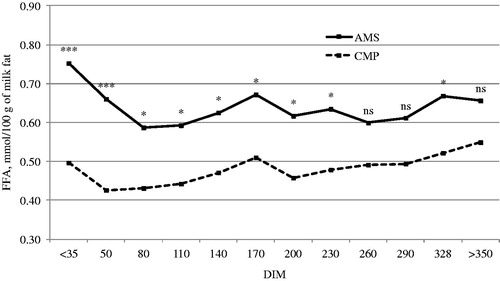
Discussion
In North Italy about half of cows are reared in small-to-medium size herds (<50 cows). The potential economic benefit of the introduction of AMS in small-to-medium herds is an attractive perspective for farmers as discussed by Rotz et al. (Citation2003) in a study which aimed at evaluating the interaction of AMS with farm size and animal production level. As previously mentioned, the main advantages of AMS compared with CMP are the reduction of labour for milking and the increase of milk production.
Effects of AMS on milk yield and quality traits
Cows from AMS produced 3.4% more milk per day than cows from CMP; this result may be the consequence of the direct relationship between milking frequency and milk secretion. Several authors demonstrated an increase of milk production (6 to 28%) with milking frequency (Hillerton et al. Citation1990; Knight et al. Citation1992; Klei et al. Citation1997; Stelwagen Citation2001; Løvendahl & Chagunda Citation2011). Traditional milk quality traits were not affected by milking system, in agreement with the literature (Amos et al. Citation1985; Svennersten-Sjaunja et al. Citation2000; Abeni et al. Citation2005; Innocente & Biasutti Citation2013). Also, Klei et al. (Citation1997) did not report any positive effect between two and three milkings per day for protein and fat percentages. Compared to CMP, the use of AMS increased MY in early lactation. Abeni et al. (Citation2005) and Svennersten-Sjaunja and Pettersson (Citation2008) reported significant interaction effects between milking system and stage of lactation from 91 to 180 days in milk, supporting findings from the present research. Concerning PP and CP, no information about the effect of AMS on these traits across lactation are available in the literature for comparison. Concerning milk SCC, the effect of introducing AMS in the dairy herd is quite contradictory among studies; our results indicated no difference in SCS for cows milked with AMS and CMP, although they were numerically higher in AMS milk. An increase of milk SCC after the introduction of AMS was observed by several authors in bulk milk (Klungel et al. Citation2000; Rasmussen et al. Citation2001; Kruip et al. Citation2002; de Koning et al. Citation2003).
To our knowledge, no information is available in the scientific literature to explain the interaction between milking system and parity. Our results demonstrated that cows of parity 5 or greater produced 2.6 kg/d more milk in AMS than CMP, and it can be hypothesised that cows from AMS and so milked more than two times per day maintain a better productive and health status respect to cows milked in CMP. The possibility for the cows to choose the time of milking could preserve the health of the udder, especially in high-productive animals.
Effects of AMS on milk pH and coagulation properties
Milk from herds equipped with AMS had slightly worse technological features compared with milk from herds equipped with CMP; indeed, pH (6.64 vs. 6.63; p < .05) and RCT (23.9 vs. 22.7 min; p < .05) were greater in AMS than CMP. Milk acidity, and in particular pH, affects the rate of hydrolysis of k-casein by chymosin; this relationship confirms our results and was reported also by Bastian et al. (Citation1991) who studied the effect of plasmin activity on clotting time and pH, and by several phenotypic and genetic studies carried out in Italian HF farms (De Marchi et al. Citation2013; Tiezzi et al. Citation2013; Penasa et al. Citation2014). Innocente and Biasutti (Citation2013) did not detect significant differences between AMS and CMP for MCP determined by Formagraph in bulk milk samples collected in 14 farms and O’Brien et al. (Citation2002) did not report significant effects on MCP by increasing milking frequency. The statistical analysis revealed that a30 was significantly affected by the interaction effect between milking system and stage of lactation.
Effects of AMS on milk fatty acid composition
Overall, no significant differences between milk from AMS and CMP existed for FA composition, except for C16:0 and FFA contents, which were greater in AMS than CMP. These results agree with findings of Svennersten-Sjaunja et al. (Citation2002) who investigated the effect of increasing milking frequency on milk FA profile and of Knight et al. (Citation1992) who explored the variation of acetyl-CoA carboxylase and FA synthetase in udder half milked 4 times daily compared with the other udder half milked twice daily. In contrast, Wiking et al. (Citation2006) reported a significant reduction of PUFA by increasing milking frequency. Greater content of FFA in milk from AMS confirms previous studies conducted at farm and udder level (Wiking et al. Citation2006). The difference between AMS and CMP for FFA across lactation seems to be related to MY; in fact, greater contents in FFA for cows milked by AMS with respect to CMP were depicted in the first three months of lactation where cows produced more milk per day. Rasmussen et al. (Citation2006) demonstrated that air intake in AMS did not have the largest influence on FFA at the cow or herd level and confirmed that MY at individual milking influences the FFA concentration. Abeni et al. (Citation2005), analysing individual samples of HF cows, reported that milk fat lipolysis was affected by milking system and showed that FFA content was greater in milk fat from AMS than CMP. Free FA derive from the degradation of milk fat into glycerol and FFA through lipolysis reaction. Cooling and mechanical treatments of milk can break the membrane of the fat globule, resulting in an increase of FFA. The increasing of FFA is firstly related to increasing milking frequency or short milking intervals (Klei et al. Citation1997; Justesen & Rasmussen Citation2000; Klungel et al. Citation2000; Wiktorsson et al. Citation2000; de Koning & Rodenburg Citation2004; Slaghuis et al. Citation2004; Wiking et al. Citation2006). Another explanation for the greater FFA content of milk from cows milked more than 2 times daily is the fat globule size; shorter milking intervals are associated with larger fat globules which are more susceptible to lipolysis than smaller ones (Wiking et al. Citation2003; Abeni et al. Citation2005).
Conclusions
The present study investigated the effect of milking system on milk coagulation traits and fatty acid composition of HF cows. Milking system had an effect on MY and FFA content. On average, cows milked by AMS produced more milk and with greater FFA content than cows milked by CMP. Traditional milk quality traits were not affected by milking system and MCP differed marginally between AMS and CMP. Results from the present study suggest that AMS can be adopted without detrimental effects on milk features, especially on milk coagulation characteristics. An economic analysis is required before recommending this system to producers in specialised cheese production areas.
Acknowledgements
The authors thank the Breeders Association of Veneto Region (ARAV, Padova, Italy) for providing data used in this study.
Disclosure statement
The authors declare that there is no conflict of interest associated with this paper. The authors alone are responsible for the content and writing of this article.
References
- Abeni F, Degano L, Calza F, Giangiacomo R, Pirlo G. 2005. Milk quality and automatic milking: fat globule size, natural creaming, and lipolysis. J Dairy Sci. 88:3519–3529.
- Abeni F, Degano L, Giangiacomo R, Speroni M, Pirlo G. 2003. Robotic milking and milk quality: effects on the cheese making properties of milk. Ital J Anim Sci. 2:301–312.
- Amos HE, Kiser T, Loewenstein M. 1985. Influence of milking frequency on productive and reproductive efficiencies of dairy cows. J Dairy Sci. 68:732–739.
- Bastian ED, Brown RJ, Ernstrom CA. 1991. Plasmin activity and milk coagulation. J Dairy Sci. 74:3677–3685.
- Berglund I, Pettersson K, Svennersten-Sjaunja K. 2002. Automatic milking: effects on somatic cell count and teat end-quality. Livest Prod Sci. 78:115–124.
- de Koning K, Rodenburg J. 2004. Automatic milking: State of the art in Europe and North America. In Proc. Automatic Milking – A Better Understanding. Lelystad, The Netherlands. Wageningen, The Netherlands: Wageningen Acad. Publ.; p. 27–37.
- de Koning K, Slaghuis B, van der Vorst Y. 2003. Robotic milking and milk quality: effects on bacterial counts, somatic cell counts, freezing points and free fatty acids. Ital J Anim Sci. 2:291–299.
- De Marchi M, Toffanin V, Cassandro M, Penasa M. 2013. Prediction of coagulating and noncoagulating milk samples using mid-infrared spectroscopy. J Dairy Sci. 96:4707–4715.
- De Marchi M, Toffanin V, Cassandro M, Penasa M. 2014. Invited review: mid-infrared spectroscopy as phenotyping tool for milk traits. J Dairy Sci. 97:1171–1186.
- Dijkhuizen AA, Huirne RBM, Harsh SB, Gardner RW. 1997. Economics of robot application. Comput Electron Agric. 17:111–121.
- Hillerton JE, Knight CH, Turvey A, Wheatley SD, Wilde CJ. 1990. Milk yield and mammary function in dairy cows milked four times daily. J Dairy Res. 57:285–294.
- Innocente N, Biasutti M. 2013. Automatic milking systems in the Protected Designation of Origin Montasio cheese production chain: effects on milk and cheese quality. J Dairy Sci. 96:740–751.
- International Committee for Animal Recording (ICAR). 2014. International Agreement of Recordings Practices—Guidelines Approved by the General Assembly held in Berlin, Germany, on May 2014. ICAR. Roma, Italy.
- Justesen P, Rasmussen MD. 2000. Improvement of milk quality by the Danish AMS self-monitoring programme. In: Hogeveen H, Meijering A, editors. Proc. Int. Symp. on Robotic Milking, Lelystad, The Netherlands. Wageningen Pers, Wageningen, The Netherlands; p. 83–88.
- Kaartinen L, Ali-Vehmas T, Sandholm M. 1990. Effect of frequent milkings on milk NAGase, plasmin, trypsin inhibitory capacity and the quality of whey as the growth medium for mastitis pathogens. Zentralbl Veterinarmed B. 37:337–344.
- Klei LR, Lynch JM, Barbano DM, Oltenacu PA, Lednor AJ, Bandler DK. 1997. Influence of milking three times a day on milk quality. J Dairy Sci. 80:427–436.
- Klungel GH, Slaghuis BA, Hogeveen H. 2000. The effect of the introduction of automatic milking systems on milk quality. J Dairy Sci. 83:1998–2003.
- Knight CH, Hillerton JE, Kerr MA, Teverson RM, Turvey A, Wilde CJ. 1992. Separate and additive stimulation of bovine milk yield by the local and systemic galactopoietic stimuli of frequent milking and growth hormone. J Dairy Res. 59:243–252.
- Kruip TA, Morice H, Robert M, Ouweltjes W. 2002. Robotic milking and its effect on fertility and cell counts. J Dairy Sci. 85:2576–2581.
- Løvendahl P, Chagunda MG. 2011. Covariance among milking frequency, milk yield, and milk composition from automatically milked cows. J Dairy Sci. 94:5381–5392.
- Mollenhorst H, Hidayat MM, van den Broek J, Neijenhuis F, Hogeveen H. 2011. The relationship between milking interval and somatic cell count in automatic milking systems. J Dairy Sci. 94:4531–4537.
- O’Brien B, Ryan G, Meaney WJ, McDonagh D, Kelly A. 2002. Effect of frequency of milking on yield, composition and processing quality of milk. J Dairy Res. 69:367–374.
- Penasa M, Tiezzi F, Gottardo P, Cassandro M, De Marchi M. 2015. Genetics of milk fatty acid groups predicted during routine data recording in Holstein dairy cattle. Livest Sci. 173:9–13.
- Penasa M, Tiezzi F, Sturaro A, Cassandro M, De Marchi M. 2014. A comparison of the predicted coagulation characteristics and composition of milk from multi-breed herds of Holstein-Friesian, Brown Swiss and Simmental cows. Int Dairy J. 35:6–10.
- Pretto D, De Marchi M, Penasa M, Cassandro M. 2013. Effect of milk composition and coagulation traits on Grana Padano cheese yield under field conditions. J Dairy Res. 80:1–5.
- Rasmussen MD, Bjerring M, Justesen P, Jepsen L. 2002. Milk quality on Danish farms with automatic milking systems. J Dairy Sci. 85:2869–2878.
- Rasmussen MD, Blom JY, Nielsen LAH, Justesen P. 2001. Udder health of cows milked automatically. Livest Prod Sci. 72:147–156.
- Rasmussen MD, Wiking L, Bjerring M, Larsen HC. 2006. Influence of air intake on the concentration of free fatty acids and vacuum fluctuations during automatic milking. J Dairy Sci. 89:4596–4605.
- Rotz CA, Coiner CU, Soder KJ. 2003. Automatic milking systems, farm size, and milk production. J Dairy Sci. 86:4167–4177.
- Sapru A, Barbano DM, Yun JJ, Klei LR, Oltenacu PA, Bandler DK. 1997. Cheddar cheese: influence of milking frequency and stage of lactation on composition and yield. J Dairy Sci. 80:437–446.
- Shipe WF, Senyk GF, Fountain KB. 1980. Modified copper soap solvent extraction method for measuring free fatty acids in milk. J Dairy Sci. 63:193–198.
- Slaghuis BA, Bos K, de Jong O, Tudos AJ, te Giffel MC, de Koning K. 2004. Robotic milking and free fatty acids. In Proc. Automatic Milking – A Better Understanding. Lelystad, The Netherlands. Wageningen, The Netherlands: Wageningen Acad. Publ; p. 341–347.
- Stelwagen K. 2001. Effect of milking frequency on mammary functioning and shape of the lactation curve. J Dairy Sci. 84(E Suppl):E204–E211.
- Svennersten-Sjaunja K, Berglund I, Pettersson G. 2000. The milking process in an automated milking system, evaluation of milk yield, teat condition and udder health. In Proc. Int. Symp. on Robotic Milking, Lelystad, The Netherlands. Wageningen, The Netherlands: Wageningen Pers; p. 277–287.
- Svennersten-Sjaunja K, Persson S, Wiktorsson H. 2002. The effect of milking interval on milk yield, milk composition and raw milk quality. In Proc. Int. Symp. The First North American Conference on Robotic Milking, Toronto, ON, Canada; p. V-43–V-48.
- Svennersten-Sjaunja KM, Pettersson G. 2008. Pros and cons of automatic milking in Europe. J Anim Sci. 86:37–46.
- Tiezzi F, Pretto D, De Marchi M, Penasa M, Cassandro M. 2013. Heritability and repeatability of milk coagulation properties predicted by mid-infrared spectroscopy during routine data recording, and their relationships with milk yield and quality traits. Animal. 7:1592–1599.
- Wiking L, Björck L, Nielsen JH. 2003. Influence of feed composition on stability of fat globules during pumping of raw milk. Int Dairy J. 13:797–803.
- Wiking L, Nielsen JH, Båvius AK, Edvardsson A, Svennersten-Sjaunja K. 2006. Impact of milking frequencies on the level of free fatty acids in milk, fat globule size, and fatty acid composition. J Dairy Sci. 89:1004–1009.
- Wiktorsson H, Svennersten-Sjaunja K, Salomonsson M. 2000. Short or irregular milking intervals in dairy cows - Effects on milk quality, milk composition and cow performance. In Proc. Int. Symp. on Robotic Milking, Lelystad, The Netherlands. Wageningen, The Netherlands:Wageningen Pers; p. 128–129.