Abstract
The present study was carried out to investigate the effect of different levels of guanidine acetic acid (GAA) on the performance, carcase yield and energetic molecular metabolites of breast muscle in broiler chickens fed with wheat-soy diet containing different levels of nutrient densities. 450- day-old male broiler chicks (Ross 308), assigned in a completely randomised design experiment with factorial arrangement 3 × 3, 9 treatments and 5 replicates of 10 birds each. The diets included three levels of GAA supplementation (0, 0.6, 1.2 g/kg of diet) and three dietary nutrient density (ND) levels (low; 2800 kcal ME/kg, medium; 2950 kcal ME/kg and high; 3100 kcal ME/kg with constant rates of ME to other nutrients). The experimental diets were fed ad libitum from 1–42 days of age. One bird per pen was euthanized on day 10 and 42 to determine the energetic molecular metabolites concentrations of adenosine phosphate (ATP, ADP and AMP), phosphocreatine (PCr) and creatinine (CRN) in breast muscles. The inclusion of 0.6 g/kg GAA in wheat–soy diet, improved production performance, the relative weight of breast yield and increased the concentration of high phosphate energy metabolites (PCr, ATP/ADT and PCr/ATP) in breast muscle. The supplementation of high-density diet with GAA compared with the low-density diet improved broiler chicken’s performance. It is concluded that the use of GAA in diet may facilitate the conversion of high-energy phosphates to form ATP in muscles and thus improve broiler chicken’s performance.
Introduction
Guanidine acetic acid (GAA); also known as glycocyamin or guanidine acetate; is a natural precursor for creatine in the animal body. Creatine is a nitrogenous organic acid that involves in the energy metabolism through the creatine and phosphocreatine (PCr) system to all cells in the body mainly muscle. The creatine and phosphocreatine system functions as a backup to the ADP/ATP-cycle to store and mobilise energy when required on short notice by increasing the formation of ATP (Wyss & Kaddurah-Daouk Citation2000).
It is likely that the requirement for creatine is proportionally greater in growing animals because of their high need to supply creatine for muscles in the regeneration of ATP from creatine and phosphocreatine system, in addition to replacing creatine losses in the form of creatinine, there may be a need to provide creatine to the growing tissues (Brosnan et al. Citation2009). Therefore, the capacity for de novo synthesis may be limiting in high-yielding farm animals, especially in those fed all-vegetable diets (Wallimann et al. Citation2007). The use of creatine as a feed additive in animal and poultry diets shows some drawbacks such as instability and high cost compared with GAA, which is more stable and less expensive (Baker Citation2009).
Hence, in view of eradicating animal-origin feedstuffs from poultry diet, broiler chickens generally fed diets containing vegetable source, based on maize/wheat-soy meal, at this situation might be deficient in creatine and supplementation of broiler chickens diets with GAA (as creatine precursor) might restore the creatine load in tissues (Ringel et al. Citation2008). Broiler chickens diet supplemented with GAA might be favourable in broiler chickens with fast growing ability because of their high need to supply creatine for muscles in the regeneration of ATP from creatine and PCr system that have paramount importance in the muscle energy management of fast-growing broiler chickens (Nain et al. Citation2008; Michiels et al. Citation2012).
Wheat is the preferred grain for cultivation in many areas in Iran; therefore, it is often replaced for maize in poultry diets. The objective of the current study was to evaluate the response of broiler chickens to GAA added to all-vegetable diets on performance and muscle energy metabolites of broiler chickens fed with wheat–soy diet containing different nutrient densities.
Materials and methods
Feed stuffs composition
A wheat sample (Falat variety) was obtained from Khorasan Razavi province agricultural research centre (Northeast of Iran). The chemical compositions of wheat and soy meal were determined by NIR through Evonik–Degussa agent in Tehran, Iran and data were used to formulate the experimental diets.
Birds, housing and care
The Animal Care Committee of the Ferdowsi University of Mashhad approved experimental procedures. A total of 450-day-old male broiler chicks of commercial strain (Ross 308) were obtained from a commercial hatchery. The birds randomly were assigned to nine dietary treatments with five replicates/treatment of 10 birds/replicate. Each pen was one square metre space and, floors were covered with wood shaving. The house temperature at initially was maintained at 32–34 °C, and was decreased to 3.5 °C per week to reach a constant temperature of 20–22 °C at 24 days of age and was kept constant thereafter. The relative humidity was 50–60% and the lighting programme was 23L: 1D cycle throughout the experimental period.
Experimental design and diets
A completely randomised design (CRD) experiment with a factorial arrangement 3 × 3 of three levels of GAA1 (0.0, 0.6 and 1.2 g GAA/kg of diet) and three levels of dietary nutrient densities (low, medium and high) was used. Diets were formulated, based on the nutrient requirements of Ross-308 rearing guideline (Aviagen Citation2015), and the nutrient densities were adjusted with the three following metabolisable energy levels 2800, 2950 and 3100 kcal/kg to have the constant energy to nutrient ratios for each feeding period (Table ). All birds had free access to mash feed and water throughout the experiment.
Table 1. Ingredients and composition of experimental diets with/without supplemental GAATable Footnotea.
Measurements
Performance traits
Pen body weights (BWs) were recorded at 0, 10, 24 and 42 days of ages and feed intake during 1–10, 11–24 and 25–42 days of ages, by weighing the offered and residual feed at the beginning and end of each period. The growth performance as mean live body weight (LBW), daily weight gain (ADG) and daily feed intake (ADFI) were calculated during each experimental period. Daily died chick was weighed and recorded for correct the growth performance. The FCR was calculated as the amount of feed consumed divided by pen weight gain including the weight gain of the mortalities.
Slaughter and meat sampling procedures
One bird from each pen close to the average pen weight was randomly selected, weighed, and then was compassionately slaughtered at 10 and 42 days of ages, after 8h feed withdrawal. Carcass was obtained by removing head, feathers, feet and gastro-intestinal tract. After chilling for 24 h at 2 °C, carcases were cut according to a standardised procedure (Uijttenboogaart & Gerrits Citation1982) to determine skinless breast weight. In order to measure molecular concentrations of energy metabolites in muscle, about 1 g of muscle from about 1.5 cm deep in the right major pectoral tissue was collected, wrapped in an aluminium foil and kept at −80 °C freezer for later analysis.
Measurement of ATP, ADP, AMP, PCr and creatinine in muscles
The concentrations of adenosine phosphate (ATP, ADP and AMP), PCr and creatinine (CRN) in breast muscles were evaluated by reverse-phase-HPLC. The Liu et al. (Citation2006) method was used with some modification as follows.
Preparation of standard stock solutions
The sodium salt standard samples of 5-ATP, 5-ADP and 5-AMP were purchased from Sigma-Aldrich Inc. The ATP, ADP and AMP standard stock solutions of 1000 μg/mL were prepared by dissolving 50 mg of ATP, ADP and AMP standards in 50 mL of per-chloric acid solution (0.4 M HClO4) and stored at 4 °C.
Sample preparation
A frozen muscle sample (200 mg) was cut and homogenised for 1 min in 1.0 mL of ice-cold 0.4 M HClO4 and kept in an ice bath for 10 min, then homogenate was centrifuged for 10 min at 3600 × g, in refrigerated centrifuge (4 °C). Supernatant (850 μL) was collected and adjusted to a pH of 6.5 with 850 μL of 0.6 M K2HPO4, and mixture was mixed (by vortex) for 20 s and centrifugation was repeated for 5 min. The supernatant (1.5 mL) was collected, stored at −20 °C and analysed within 24 h.
HPLC analysis
The separation of muscle ATP, ADP, AMP, PCr and CRN were performed using an Alliance HPLC system Waters-2695 (Waters Corporation, Milford, MA), equipped with an integrated auto sampler. Chromatographs were recorded with a Waters-2996 PDA UV detector. The HPLC conditions were set as follows:
Purospher STAR RP-18e column (250 × 4.6 mm i.d., 5 μm) with a column temperature of 30 °C
Mobile phase, acetonitrile: 50 mM KH2PO4 buffer solution (vol/vol = 10:90, pH adjusted to 6.5 for ATP, ADP, AMP and 6.8 for PCr and CRN using 1 M KOH) at a flow rate of 0.8 mL/min
Detection wavelength was 254 nm and injection volume was 20 μL
Sample and mobile phase water was prepared using a Milli-Q water purification system (Millipore Corp., Bedford, MA) and
Both the sample and mobile phase solution were filtered with 0.45-μm Durapore membrane polyvinylidene fluoride filters (Millipore Corp)
Standard curves preparation
The standard stock solutions (1000 μg/mL) were diluted to 5, 10, 20, 40, 80, 160 and 320 μg/mL in deionised water. A volume of 20 μL of each standard sample was used for HPLC analysis. The standard curve and regression equation were established between chromatographic peak area (y-axis, mV min) and standard sample concentration (x-axis, μg/mL). The retention times for ATP, ADP, AMP, PCr and CRN were 3.4, 3.7, 4.4, 3.5 and 4.2 min, respectively.
Statistical analysis
The data were tested for main effects of diet nutrient density (ND) levels and GAA supplementation and for their interaction. All data were analysed by ANOVA using the GLM procedure of SAS 9.1 software (SAS Citation2003). Means were compared for significant differences using Tukey–Kramer’s multiple range test (p < .05). Linear and quadratic models were fitted to data to describe the relationships between depended variables with dietary GAA levels. Pen was considered the experimental unit for performance analysis, and individual breast samples were used as experimental unit for energy metabolites analysis.
Results and discussion
Performance
Effect of dietary ND and GAA supplementation on BW, average daily gain (ADG), average daily feed intake (ADFI) and feed conversion ratio (FCR) in male broiler chickens are shown in the Table .
Table 2. Effect of dietary ND and GAA supplementation on average live body weight (LBW), ADG, ADFI and FCR in male broiler chickensTable Footnote1.
A significant interaction between ND and GAA in diet was observed on FI during 1–10 days period (p < .05). The FI numerically increased as GAA levels increased in high ND diet fed birds, but in low and medium nutrient density (MND) diets fed birds, the highest FI was in those fed diet contained 0.6g/kg GAA (Figure ). A greater FI response to GAA levels were observed at higher ND diet, The FI increased linearly with increasing GAA level in high nutrient dense diet (p < .06). This can be associated with the effect of diet supplementation with GAA on the energy metabolism process (Ringel et al. Citation2008). This response could be due to the better energy utilisation in chicks receiving the high ND diet supplemented with GAA. A similar effect was reported by Mousavi et al. (Citation2013), even though the differences between GAA-treated and control groups were not significant (p = .09). It is shown that supplementation of diet with GAA reduced feed intake in turkeys (Lemme et al. Citation2010). In contrast, Michiels et al. (Citation2012) found slightly higher feed intakes in chickens receiving diet supplemented with 0.6 or 1.2 g/kg GAA compared to those fed control diet. No effect of GAA on feed intake of broilers was observed by Ringel et al. (Citation2007).
Figure 1. Effect of diet GAA supplementation on ADFI (g/b/d) of broiler chicks fed three dietary nutrient densities during 1–10 days of age: low nutrient density (LND), Y = 28.433 − 11.833X, R2 = .13; MND, Y = 27.38 − 7.267X, R2 = .055; and HND, Y = 27.291 + 16.933X, R2 = .416.
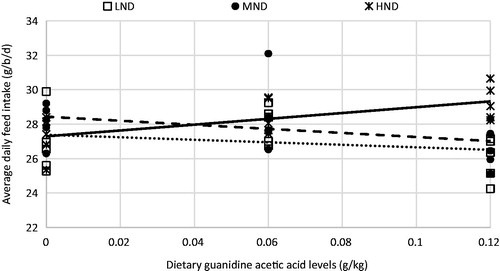
The interaction between diet ND and addition GAA on the LBW at 24 day, WG at 11–24 days and FCR at 1–42 days were close to significant level (p < .10). Live body weight at 24 days and ADG during 11–24 days were significantly increased and FCR during 1–42 days was significantly decreased (p < .05) in birds fed diet with high nutrient density (HND) and 1.2 g GAA/kg compared with those fed other dietary treatments. Feeding high nutrient density (HND) diet increased growth rate (Saleh et al. Citation2004) and muscle creatine store under this condition may lead to improve bird’s production performance (Mousavi et al. Citation2013).
Our results showed an improvement in FCR of birds fed diet supplemented with GAA (Table ). Similarly, several other studies have shown that dietary GAA supplementation improved FCR in broiler chickens (Ringel et al. Citation2007; Michiels et al. Citation2012; Dilger et al. Citation2013; Mousavi et al. Citation2013). This positive effect of GAA might be related to its ability to increase muscle creatine levels (Wyss & Kaddurah-Daouk Citation2000). Michiels et al. (Citation2012) suggested that supplementation of GAA in all-vegetable diets improves performance and carcase characteristics in terms of the FCR ratio and breast meat yield. Other studies indicated that GAA supplementation of up to 0.12% to all-vegetable diets improved performance in male and female broiler chickens (Lemme et al. Citation2007a, Lemme et al. Citation2007b). Furthermore, supplementation of GAA to turkey’s diets also improved growth performance (Lemme et al. Citation2010). This positive effect of GAA may be associated with its ability to increase the cells creatine level. The higher muscle creatine demands higher nutrients, which can be provided with higher ND diets, this can lead to a more efficient utilisation of dietary nutrients, improved growth performance and FCR. It is reported that creatine is a semi-essential compound for animal growth (Lemme et al. Citation2007c). As creatine deficiency may act as a limiting factor for growth, especially in birds that fed solely with plant ingredients, because plant feedstuff are absolutely deficient in creatine (Michiels et al. Citation2012).
Breast muscles energetic molecular metabolites concentrations
The effects of diet ND and GAA supplementation and their interaction on concentrations of ATP, ADP, AMP, the ATP/ADP ratio, PCr, CRN, PCr/ATP ratio and PCr/CRN ratio in breast muscles at 10 and 42 days of age are shown in Table .
Table 3. Effect of diet ND and GAA supplementation on energy metabolites in breast muscle (mg/kg of fresh matter) of male broiler chickensTable Footnote1.
The effect of dietary ND was significant on ATP, ADP and AMP concentration of broiler chickens breast muscle at 10 days of age (p < .05). With increased dietary ND, the concentration of high-energy phosphates numerically increased in the breast muscles. The average concentration of ATP, PCr, CRN, ATP/ADP ratio and PCr/CRN ratio in breast muscle were significantly increased by GAA supplementation of diet at 10 days of age (p < .05). Comparing to birds fed control diet, the addition of 0.6g GAA/kg to diet significantly increased chicken’s breast muscle ATP concentration (150 ppm vs 200 ppm), PCr concentration (3945 ppm vs 6608 ppm), CRN concentration (33 ppm vs 62 ppm) and ATP/ADP ratio (2.7 vs 6.1) at 10 days of age. The ATP, CRN and PCr concentrations increased quadratically (p < .01) with increase in GAA level, which peaked around 0.06 g GAA/kg diet (Figure ). The interaction between the ND and GAA was not significant.
Figure 2. Effect of dietary GAA supplementation on energetic molecular metabolite concentrations in breast muscles of broiler chicks at 10 days of age: (a) ATP, Y = 150.03 + 1664.20X – 13818X2, R2 = .20; (b) creatinine (CRN), Y = 33.44 + 817.76X– 5818.40X2, R2 = .27 (CRN) and (c) phosphocreatine (PCr), Y = 3954 + 87133x − 715010x2, R2 = .44.
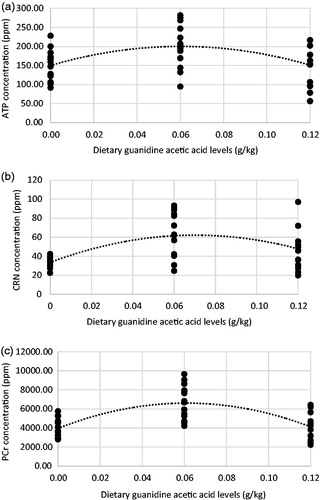
The effect of dietary ND on concentration of PCr, ATP/ADP ratio and PCr/ATP ratio in breast muscle was significant at 42 days of age (p < .05). With increased dietary ND, the ATP/ADP and PCr/ATP ratio were increased in the breast muscles. The concentration of PCr, PCr/ATP and PCr/CRN ratio in breast muscle was significantly affected by dietary GAA concentration at 42 days of age (p < .05). Diet supplemented with GAA caused an increased PCr, PCr/ATP and PCr/CRN ratio in breast muscle so that birds fed 0.6 g GAA/kg supplemented diet had significantly higher PCr concentration (9062 vs 7218 ppm), PCr/ATP ratio (28.4 vs 20.3) and PCr/CRN ratio (288.6 vs 189.6) in their breast muscles. The interaction between the ND and GAA was not significant.
It is shown that the addition of GAA to the broiler chickens diet, especially at the rate of 0.6g/kg increased the ability of breast muscle cells to store more energy as high-energy phosphate molecules at 10 and 42 days of age. Breast muscle of birds fed diet containing 0.6g GAA/kg contained higher concentrations of CRN, PCr, ATP, ATP/ADP ratio and PCr/ATP ratio compared with those fed control diet. Other investigators (Haussinger Citation1996; Hultman et al. Citation1996; Young et al. Citation2007; Michiels et al. Citation2012) were reported similar results. The increase in ATP/ADP and PCr/CRN in the muscle tissues, via the addition of GAA to diet shown to have a major role in energy metabolism (Lemme et al. Citation2007b; Lemme et al. Citation2010). GAA is the single immediate precursor of creatine in animal’s tissue (Bloch & Schoenheimer Citation1941). Creatine is a key energy reserve in animal tissues and prevents cells devoid from ATP, it helps to avoid the cellular ATP depletion through the immediate provision of high energy phosphates to regenerate the ATP molecule from ADP (Wyss & Kaddurah-Daouk Citation2000).
Tissues with high-energy demand, such as the skeletal muscle, take up creatine. The concentration of ATP in skeletal muscle would result in a muscle contraction of only a few seconds (Wallimann et al. Citation1992). Fortunately, during times of increased energy demands, the phosphagen (ATP/PCr) system rapidly re-synthesizes ATP from ADP with the use of PCr through a reversible reaction creatine kinase (CK). Additionally, in most muscles, the ATP regeneration capacity of CK is very high and is therefore not a limiting factor. Although the cellular concentrations of ATP are small, changes are difficult to detect because ATP is continuously and efficiently replenished from the large pools of PCr and CK. Creatine has the ability to the reserve of PCr in muscle, potentially increasing the muscle’s ability to resynthesize ATP from ADP to meet the increased energy demands (Spillane et al. Citation2009).
While the animal by-products contain creatine, vegetable ingredients are not a source of this compound. In contrast to animal sources, purely vegetable-based diets are free of creatine, and creatine must be synthesised de novo only from GAA. Brosnan et al. (Citation2009) reported that the addition of GAA to the diet of fast-growing broiler chickens diet could be useful to meet the needs of birds to creatine (Brosnan et al. Citation2009). Approximately 66–75% of creatine requirements are met endogenously, and the remainder must be supplied by diet (Ringel et al. Citation2007). Therefore, vegetable diets fail to supply creatine to poultry and may increase the requirements of arginine and glycine necessary for endogenous creatine synthesis (Wyss & Kaddurah-Daouk Citation2000). In this experiment, our results indicated that the relative weight of breast meat has increased with an increase in the level of GAA, birds fed diet supplemented with 0.6 g GAA/kg diet compared to those fed control and/or 1.2 g GAA/kg diet had have higher relative weight of breast muscle (p < .16). This can be under the effects of creatine phosphate/ATP rate in the muscle. Thus, the creatine and creatine phosphate concentrations will be increased in all muscles by the addition of GAA to vegetable-based diet (Ringel et al. Citation2008; Baker Citation2009). Loading creatine in the body such as breast muscles can be useful to increase muscle growth or its work strength (Michiels et al. Citation2012). Potential increase in water uptake by muscle cells and increased muscle cell volume can be affected by the addition of GAA to the diet and thus increasing the relative weight of breast muscle in broiler chickens (Williams & Branch Citation1998).
Conclusions
GAA supplementation in diet at the rate of 0.6 and 1.2 g/kg markedly improved the production performance, the relative weight of pectoral muscle and elevated the concentration of high phosphate energy metabolites in breast muscle cells. The addition of GAA to high ND diet was more effective than low and MND on broiler chicken’s performance. The PCr/CRN and ATP/ADP ratios in the breast muscles of birds fed diet supplemented with GAA were markedly increased compared to those fed control diet. This indicates that PCr increased buffering capacity for ATP hydrolysis and supports the idea that creatine-loaded muscles have the capacity of increased muscle growth or work, which might be beneficial for skeletal muscle growth. The GAA has the potential to rapidly mobilised reserve of high-energy phosphate for ATP formation.
Disclosure statement
The authors report no conflicts of interest. The authors alone are responsible for the content and writing of this article.
Note
Additional information
Funding
Notes
1 Evonik Degussa GmbH, Hanau-Wolfgang, Germany.
Reference
- Aviagen. 2015. Ross 308: broiler nutrition specification USA.
- Baker DH. 2009. Advances in protein-amino acid nutrition of poultry. Amino Acids. 37:29–41.
- Bloch K, Schoenheimer R. 1941. The biological precursors of creatine. J Biol Chem. 1138:167–194.
- Brosnan JT, Wijekoon EP, Warford-Woolgar L, Trottier NL, Brosnan ME, Brunton JA, Bertolo RF. 2009. Creatine synthesis is a major metabolic process in neonatal piglets and has important implications for amino acid metabolism and methyl balance. J Nutr. 139:1292–1297.
- Dilger RN, Bryant-Angeloni K, Payne RL, Lemme A, Parsons CM. 2013. Dietary guanidino acetic acid is an efficacious replacement for arginine for young chicks. Poult Sci. 92:171–177.
- Haussinger D. 1996. The role of cellular hydration in the regulation of cell function. Biochem J. 313:697–710.
- Hultman E, Soderlund K, Timmons JA, Cederblad G, Greenhaff PL. 1996. Muscle creatine loading in men. J Appl Physiol. 81:232–237.
- Lemme A, Gobbi R, Helmbrecht A, Van Der Klis JD, Firman J, Jankowski J, Kozlowsk K. 2010. Use of guanidine acetic acid in all-vegetable diets for turkeys. 4th Turkey Sci Prod Conf.
- Lemme A, Ringel J, Rostagno HS, Redshaw MS. 2007a. Supplemental guanidine acetic acid improved feed conversion, weight gain, and breast meat yield in male and female broilers. Proc. 16th Eur Symp Nutr, Strasbourg, France.
- Lemme A, Ringel J, Sterk A, Young JF. 2007b. Supplemental guanidine acetic acid affects energy metabolism of broilers. Proc. 16th Eur Symp Poult Nutr, Strasbourg, France.
- Lemme A, Tossenberger J, Ringel J. 2007c. Digestibility and availability of the creatine source guanidino acetic acid in broilers. J Anim Sci. 85(Suppl. 1):153.
- Liu H, Jiang YM, Luo YB, Jiang WB. 2006. A simple and rapid determination of ATP, ADP and AMP concentrations in pericarp tissue of litchi fruit by high performance liquid chromatography. Food Technol Biotech. 44:531–534.
- Michiels J, Maertens L, Buyse J, Lemme A, Rademacher M, Dierick NA, De Smet S. 2012. Supplementation of guanidinoacetic acid to broiler diets: effects on performance, carcass characteristics, meat quality, and energy metabolism. Poult Sci. 91:402–412.
- Mousavi SN, Afsar A, Lotfollahian H. 2013. Effects of guanidinoacetic acid supplementation to broiler diets with varying energy contents. J Appl Poult Res. 22:47–54.
- Nain S, Ling B, Alcorn J, Wojnarowicz CM, Laarveld B, Olkowski AA. 2008. Biochemical factors limiting myocardial energy in a chicken genotype selected for rapid growth. Comp Biochem Physiol A Mol Integr Physiol. 149:36–43.
- Ringel J, Lemme A, Knox A, McNab J, Redshaw MS. 2007. Effects of graded levels of creatine and guanidino acetic acid in vegetable-based diets on performance and biochemical parameters in muscle tissue. Proc 16th Eur Symp Poult Nutr, Strasbourg, France.
- Ringel J, Lemme A, Redshaw MS, Damme K. 2008. The effects of supplemental guanidino acetic acid as a precursor of creatine in vegetable broiler diets on performance and carcass parameters. Poult Sci. 87 (Suppl. 1):72.
- Saleh EA, Watkins SE, Waldroup AL, Waldroup PW. 2004. Effects of dietary nutrient density on performance and carcass quality of male broilers grown for further processing. Int J Poult Sci. 3:1–10.
- SAS. 2003. User's guide: Statistics, Version 9.1. vol. 2. Cary, NC: S.A.S Institute.
- Spillane M, Schoch R, Cooke M, Harvey T, Greenwood M, Kreider R, Willoughby DS. 2009. The effects of creatine ethyl ester supplementation combined with heavy resistance training on body composition, muscle performance, and serum and muscle creatine levels. J Int Soc Sports Nutr. 6:1–14.
- Uijttenboogaart G, Gerrits AR. 1982. Methods of dissection of broiler carcasses and description of parts. Spelderholt Report 370. Beekbergen, The Netherlands.
- Wallimann T, Tokarska-Schlattner M, Neumann D, Epand RM, Epand RF, Andres RH, Widmer HR, Hornemann T, Saks VA, Agarkova I, et al. 2007. The phosphocreatine circuit: molecular and cellular physiology of creatine kinases, sensitivity to free radicals, and enhancement by creatine supplementation. In: Molecular system bioenergetics: energy for Life Weinheim, Germany: Wiley-VCH; p. 195–264.
- Wallimann T, Wyss M, Brdiczka D, Nicolay K, Eppenberger HM. 1992. Intracellular compartmentation, structure and function of creatine kinase isoenzymes in tissues with high and fluctuating energy demands: the 'phosphocreatine circuit' for cellular energy homeostasis. Biochem J. 281:21–40.
- Williams MH, Branch JD. 1998. Creatine supplementation and exercise performance: an update. J Am Coll Nutr. 17:216–234.
- Wyss M, Kaddurah-Daouk R. 2000. Creatine and creatinine metabolism. Physiol Rev. 80:1107–1213.
- Young JF, Bertram HC, Theil PK, Petersen AG, Poulsen KA, Rasmussen M, Malmendal A, Nielsen NC, Vestergaard M, Oksbjerg N. 2007. In vitro and in vivo studies of creatine monohydrate supplementation to Duroc and Landrace pigs. Meat Sci. 76: 342–351.