Abstract
Iron is an important mineral for animal development and function. The present study was carried out to evaluate the effect of FeSO4 replacement with cysteine-coated Fe3O4 nanoparticles in quail. We hypothesised that the iron nanoparticles could be used as a dietary supplement. The quails were randomly allotted to five dietary treatments with four replicate pens and 10 birds per pen. The treatments consisted of: negative control without any iron supplementation, positive control with 120 mg/kg of FeSO4 and three experimental groups that contained 1.2, 12 and 120 mg/kg of l-cysteine coated iron oxide nanoparticles, organic iron, in their diet, respectively. The least weight gain was recorded in the negative control group. Similar weight gain, FCR, haematological and biochemical parameter results between the experimental and positive control groups show that organic iron successfully performed the biological role of iron in the quail. We concluded that 1.2 mg/kg cysteine-coated Fe3O4 nanoparticles are required and sufficient for quails’ optimal maintenance and growth. The results confirm that cysteine-coated Fe3O4 nanoparticles can be used as an iron source in the quail diet.
Keywords:
Introduction
Iron is an essential mineral in animal body. Iron deficiency was one of the most important nutrition deficiencies that causes anaemia, created by low bioavailability of iron in plant-based diet (Layrisse et al. Citation1975). The insoluble or very poorly soluble iron compounds in diets reduced the absorbability of the element (Zimermann and Hilty Citation2001). In this situation, addition of iron supplement to the diet is a necessity. A size-based strategy that promised to increase intake of micronutrients is a suitable choice to increase the absorption of poorly soluble Fe compounds (Zimermann and Hilty Citation2001). It was shown that reduction of the iron particle size by 50–60% increased Fe absorption by 50% in rats (Motzok et al. Citation1975). It was demonstrated that nutritional application of metal nanoparticles depends on their physical and chemical characteristics, such as the size and specific surface area (Bogunia-Kubik and Sugisaka Citation2002). The nanoparticles may injure cells by increasing oxidative stress (Nel et al. Citation2006) and could be the main concern in live cells nanomaterials exposure (Bogunia-Kubik and Sugisaka Citation2002). l-Cysteine as a powerful antioxidant could bind to dangerous and reactive free molecules and protect cells from the toxic effects of radiation (Lafleur et al. Citation1980). The aim of this research was to study the effects of cysteine-coated Fe3O4 nanoparticles (Fe3O4-Cys NPs) as an additive in comparison with FeSO4, on the weight gain and some blood parameters in quails.
Materials and methods
Two-hundred day-old quails that were hatched in the Faculty of Agriculture, University of Kurdistan were used in this experiment. The quails were weighed and randomly placed to five groups of four replicate cages (90 × 32 × 27 cm), containing 10 birds (mix gender) using a completely randomised design (CRD). The ingredients and composition of diet are shown in Table . The corn–soybean meal basal diet was formulated to exceed the nutrients requirement of quail recommended by National Research Council (NRC 1994). Diet and water were provided on ad libitum basis. The five dietary treatments were fed (Table ): negative control without any iron supplementation, positive control contained 120 mg/kg of common form of iron (300 mg of FeSO4, 40%) and experimental diets that contained 1.2, 12 and 120 mg/kg of organic iron (126, 12.6 and 1.26 mg of l-cysteine coated iron oxide nanoparticles, Fe3O4-Cys NPs 95%, were added to 1 kg of the basal diet, respectively). Feed intake per cage and individual body weight (BW) were determined in 10, 17, 26, 35 and 42 days and used to calculate feed conversion ratio (FCR). The experiment ended within 42 days. Blood samples were collected from wing vein of two birds per cage (one male and one female) using a needle (13 × 0.45 mm) and syringe (2.0 mL) was suitable for quails. The fresh blood samples were divided into two tubes: one EDTA-coated tube for haematological parameters assay, and the second tube without anticoagulant for collecting the serum after centrifugation. Biochemical analysis: serum (biochemical kits, Pars Azmoon Inc., Tehran, Iran) and reading in an autoanalyzer (Abbott Alcyon 300, Chicago, IL) to determine the following parameters: iron, total protein, albumin alanine transaminase (ALT), aspartate aminotransferase (AST) and alkaline phosphatase (ALP). The amount of serum thyroid stimulating hormone (TSH) and ferittin were determined (ELISA Kits, PishtazTeb, Inc., Tehran, Iran) by ELISA Plate Reader. Haematological parameters: determination of haemoglobin concentration (Cyanomethemoglobin method, BaharAfshan Kit, Tehran, Iran) was performed. The haematocrit (HCT) was done by centrifuging the blood sample for five minute at 11,000 (rpm). Red blood cell (RBC) indices, mean corpuscular volume (MCV); mean corpuscular haemoglobin (MCH) and mean corpuscular haemoglobin concentration (MCHC) were calculated.
Table 1. Ingredient composition of the diets fed (1–42 days).
Preparation of Fe3O4 nanoparticles
Different methods have been developed for production of iron oxide nanoparticles (Dang et al. Citation2007; Gawande et al. Citation2012). We used a protocol as described by Gawande et al. (Citation2012). The iron (III) chloride hexahydrate (5.4 g) and urea (3.6 g) dissolved in 200 cm3 distilled water were vigorous stirred at 85–90 °C for two hours, resulting in the solution colour gradually turning from red to brown. After cooling the solution to room temperature (30–35 °C), iron (II) sulphate heptahydrate (2.8 g) was added to the solution over a period of 30 min under vigorous stirring. Adjust the pH of solution from about <1 to 10 by adding 1 M NaOH. By increasing the pH to 10, the formation of Fe3O4 nanoparticles makes the solution colour change to black. The produced black materials were treated by ultrasound in a sealed flask for 30 min in order to disperse the particles. The flask of black solution was placed in the magnetic field for sedimenting the iron oxide nanoparticles. After washing the nanoparticles with ethanol (three times), it was washed again with distilled water (three times). Finally, a black powder was collected in a drying chamber after 4 h at 60 °C.
Synthesis of Fe3O4 nanoparticles coated by l-cysteine (Fe3O4-Cys NPs)
Fe3O4-Cys NPs were synthesised according to Gawande et al. (Citation2012). In brief, Fe3O4 NPs powder (1 g) was dispersed into distilled water (20 mL) with ultrasound at 25 C. l-Cysteine solution (1 g of l-cysteine in 40 mL of methanol/water (1:1)) and Fe3O4 MNPs aqueous solution were added to a flat bottomed beaker with a 100 mL capacity. The reaction mixture was stirred at room temperature (24 h) using a magnetic stirrer. Finally, the product was washed with absolute methanol and water. Fe3O4-Cys NPs was dried in a chamber after 4 h at 60 °C.
Fourier transform infrared (FTIR) spectrometer
To identify the presence of cysteine on the Fe3O4 nanoparticles’ surface, FTIR spectra were measured using a BRUKER VECTOR 22 FTIR spectrometer (Billerica, MA).
Magnetic properties
The magnetic properties of Fe3O4-Cys NPs have been measured by an alternating gradient force magnetometer (AGFM, model MDKG, Kashan, Iran).
Scanning electron microscopy (SEM)
The samples of produced nanoparticles have been sent to Razi Metallurgical Research Center (RMRC), Tehran, Iran to analyse the nanoparticles. The morphology of the cysteine-coated Fe3O4 nanoparticles was assessed by a scanning electron microscopy (VEGA\\TES-LMU, Brno, Czech Republic).
Determination of food iron concentration
The iron concentration in the food samples was determined by atomic absorption spectroscopy. An amount of 10 g of each diets was dried in an air oven at 100 °C for 2–3 hours. The dried sample was next charred until it coked to smoke. The coked sample was then ashed in the oven at 450 °C until a greyish ash was produced. The ash was treated with 2 mL concentrated nitric acid, heated to dryness 45 min at 100 °C and ash again for two hours at 450 °C. The whitish ash was dissolved in dilute hydrochloric acid 2%, transferred to a volumetric flask (50 mL) and made up to 25 mL. For each treatment, at least three readings were recorded and then calculated the average. The amount of iron in prepared solution samples was determined by a Varian Atomic Absorption Spectrophotometer model 220 with an air-acetylene flame. A duplicate analysis was performed for each analytical sample. Based on analytical results, the iron concentration for treatment groups was 71 ± 12.3, 197 ± 9.5, 177.7 ± 6.2, 185.33 ± 27.7 and 209.3 ± 14.6 mg/kg of diets for negative control, positive control, 1.2, 12 and 120 mg/kg Fe3O4-Cys NPs, respectively.
Statistical analysis
Data were analysed using general linear model procedure of SAS software (Cary, NC) and significant differences among treatments were separated using Dunn’s multiple range test.
Results
The FTIR results of produced samples demonstrated that Fe3O4 NPs were coated by l-cysteine. The curve of the AGFM confirmed that, the saturation magnetisation value for Fe3O4 is 66 emu/g, while the MS for cysteine-coated Fe3O4 decreased to 44 emu/g. To estimate an average size of nanoparticles, the SEM image was analysed. The mean particle size of Fe3O4-Cys NPs was determined as 46.68 nm (Figure ).
The haematological and biochemical parameters are displayed in Table . There were no differences between treatment groups on haematological data in male quails. There were statistical differences in HCT, RBC and MCV in treatment groups of female quails (p < .05). The negative control of female quails showed significant decreasing Htc, RBC count and MCV index, while all these parameters were within the normal ranges and showed no differences between the groups received Fe3O4-Cys NPs and positive control. Serum iron of both male and female quails in negative control was significantly lower than other treatment groups that received FeSO4 or Fe3O4-Cys NPs (p < .05).
Table 2. Effect of dietary Fe on some haematological and biochemical parameters of male and femaleTable Footnote* quails (mean ± SD).
Although serum ferritin concentration of all the female groups was no significant different from the control, there was a dramatically reduced serum ferritin in negative control as compared with other groups of male quails which received iron supplementation (p < .05). The serum concentration of ferritin was definitely higher in group which consumed 120 mg/kg of Fe3O4-Cys NPs than other groups (p < .05). The serum total proteins were significantly lower in birds that received 120 mg/kg of Fe3O4-Cys NPs and negative control while the total protein concentration was higher in quails that consumed the diet supplemented with 12 mg/kg of Fe3O4-Cys NPs in both male and female quails (p < .05).
Serum AST levels decreased significantly in group that received 120 mg/kg of Fe3O4-Cys NPs as compared with all other groups of male birds (p < .05). Differences were not observed between the female groups of quails for AST. There were similar results for ALP in male and female quails. ALP levels significantly increased in negative controls compared with other (p < .05).
All day-old quails in the present study had a similar BW. The results of mean daily BW, weight gain and FCR during experiment are presented in Table . The significant statistical difference in means of BW, weight gain and food conversion ratio between treatment groups was one of the most important findings in this study. According to statistical analysis, the lowest mean of BW related to negative control, while the groups that received Fe3O4-Cys NPs had a highest BW during days 10, 17, 26, 35 and 42 of experiment (p < .05). The lowest and highest levels of weight gain from 1 to 42 days were related to negative control and one of the groups received iron nanoparticles (Figure ). Although FCR of the negative control increased in days 10 and 42, the groups that consumed Fe3O4-Cys NPs had a maximum level of FCR in days 26 and 35. In accordance with all these words and the analysis of means of FCR in total period of study (Figure ), the highest FCR was related to the negative control that had no iron supplementation in diet whereas 120 Fe3O4-Cys NPs group had lower FCR than other groups (p < .05). There was no significant difference in the mortality between treatment groups.
Figure 2. Effect of dietary Fe on weight gain (g), 1–42 days. *mg/kg cysteine-coated Fe3O4 nanoparticles. **mg/kg Fe3O4 (positive control). 0 (negative control). (a–d) Within the same row with different superscripts are significantly different (p < .05).
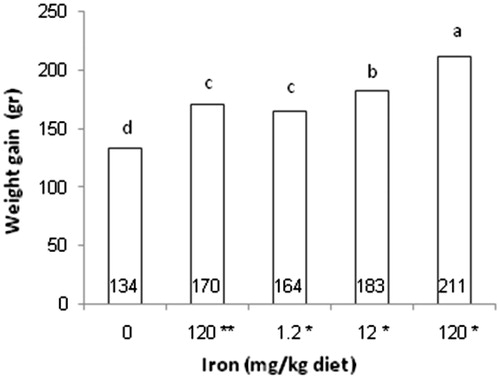
Figure 3. Effect of dietary Fe on feed conversion ratio (FCR), 1–42 days. *mg/kg cysteine-coated Fe3O4 nanoparticles. **mg/kg Fe3O4 (positive control). 0 (negative control). (a–c) Within the same row with different superscripts are significantly different (p < .05).
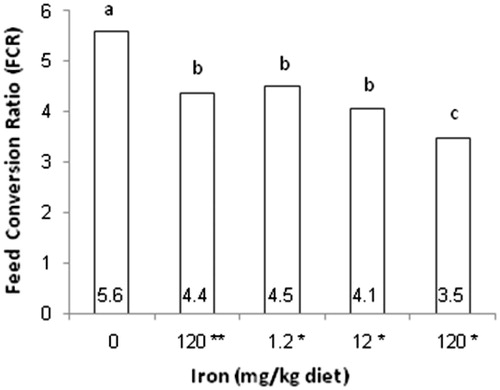
Table 3. Effect of dietary Fe on weight gain (g) and feed conversion ratio (FCR) in 10, 17, 26, 35 and 42 days in quails (mean ± SD).
Discussion
The results of FTIR, AGFM and SEM confirmed that iron nanoparticles have been coated with l-cysteine. According to the results of determination of iron in diets (Table ) and blood parameters, the negative control diet could be considered as a Fe-deficient diet (71 ± 12.3 mg/kg) that induced a typical nutritional iron-deficiency anaemia (IDA) in female quails. The iron of negative control diet could not provide iron requirement for quails. In this IDA, the blood exhibits a reduction in RBC, Htc, concentration of iron, ferritin and total protein in serum. In affected quails, the iron anaemia deficiency led to a decrease in weight gain and increase in FCR from the first day to the last day of experiment. Most cereal grains contain 30–60 mg, Fe kg–1 dry matter (DM). Bioavailability of minerals in diet was not 100% and it showed that the total amount of a mineral in a diet was not a reliable index of its nutritional value (Harland Citation1989). Most of the iron content in diets was present as organic complexes, with some bound to phytate (Morris and Elis Citation1976). Presence of anti-nutrients such as oxalates, tannins and phytates decreased mineral bioavailability (Harland Citation1989). It has been described a semilogarithmic relationship between the amount of phytates and the inhibition of absorbability of iron in diet (Hallberga Citation1987). On the basis of this fact, The National Research Council (Citation1994) recommended 120 mg/kg of iron to be supplemented in quail diet (National Research Council – NRC Citation1994). The results showed that iron-supplemented diet could improve the final weight and prevent IDA in positive control. Increased bioavailability of trace minerals improved animal health and weight performance.
As nanoparticles become smaller, its surface increased, therefore, they would be more reactive (Borm et al. Citation2006). Almost all nanoparticles stimulated toxicity effects on molecular level and caused oxidative stress that led to the damage to cellular membrane lipids, DNA and proteins. The previous reports suggested that some nanoparticles have high toxic effect such as Ag and zinc oxide while MoO3 had moderately toxic but Fe3O4, Al and MnO2 had less or no toxicity (Hussain et al. Citation2005). But some investigations showed the cytotoxic effects of iron oxide nanoparticles (Kouchesfehami et al. Citation2013; Di Bona et al. Citation2015). In order to decrease toxicity effects of nanoparticles in biological systems, coating of nanoparticles surface with albumin, dextran (Berry et al. Citation2003), polyethylene glycol (Gupta and Curtis Citation2004), methionine hydroxy (Saki et al. Citation2014) and cysteine (Mohammadi et al. Citation2017) was used as a way to increase the effectiveness of these particles. The intestinal absorption of the organic minerals was more than inorganic oxide and sulphate (Aoyagi and Baker Citation1994). The metal-amino acid chelates or complexes provided readily bioavailable amino acids and metal. These forms of metal could be utilised better than inorganic sources (Kim et al. Citation2011). Some of the amino acids such as cysteine, methionine and taurine had a protective effect against oxidative stress by participating in radical scavenging (Atmaca Citation2004). l-Cysteine was one of the most important sulphur-containing amino acids that had an antioxidant effect and protected the cell from dangerous of reactive free molecules (Pekas Citation1979; Lafleur et al. Citation1980; Mukawevho et al. Citation2014). Superoxide dismutase (SOD) molecule that converted superoxide to H2O2, had cysteine residues at its active site (Atmaca Citation2004). Cysteine was a sulphur-containing amino acid and had a main role in extracellular protection, because of its redox inconsistency. Most of the extracellular cysteine oxidised to inhibit oxidation of cellular compounds (Atmaca Citation2004). Several investigators have reported iron nanoparticles as a food additive in poultry (Nikonov et al. Citation2011; Saki et al. Citation2014), but there was a little information about application of cysteine coated iron oxide nanoparticles as a dietary additive. The results demonstrated that cysteine-coated Fe3O4 nanoparticles (Fe3O4-Cys NPs) were effective in improving the performance of quails at all supplementary levels compared to negative control. A surprising finding in this study was that the lowest supplementary level of Fe3O4-Cys NPs (1.2 mg per kg diet) had similar results as compared with positive control. One of the main purposes of the current study was to evaluate the nutritional potential of Fe3O4-Cys NPs in quails with sufficient data regarding nutritional effects of these nanoparticles that were coated with l-cysteine. Actual amount of inorganic iron FeSO4 40% was 300 mg per kg of diet while 1.26 mg of Fe3O4-Cys NPs was needed to add the diet to provide iron requirements of the quail. Therefore, it would be a great advantage that we could decrease the iron concentration of diet 238 folds using Fe3O4-Cys NPs with purity ≥95%. Finally, under conditions of the present study, bioavailability of Fe3O4-Cys NPs was similar to that from sulphate for growing quails.
Conclusions
The results confirmed that the corn-soybean meal basal diet, contained 71 ± 12.3 mg Fe per kg, was not sufficient to provide the birds’ iron requirements. Therefore, supplementation of an iron source in the diet is mandatory. Currently, iron sulphate is a common form of iron added to the birds’ diet. The quails performance, haematological and biochemical parameters analyses did not reveal any significant differences between the FeSO4 and cysteine-coated Fe3O4 nanoparticles received groups. So, we conclude that replacing 120 mg/kg iron in form of FeSO4 with 1.2 mg/kg organic iron in the diet was appropriate for high performance with beneficial effects on the quail.
Disclosure statement
The authors report no conflicts of interest. The authors alone are responsible for the content and writing of this article.
Additional information
Funding
References
- Aoyagi S, Baker DH. 1994. Copper Layrisse–amino acid complexes are partially protected against inhibitory effects of l-cysteine and l-ascorbic acid on copper absorption in chicks. J Nutr. 124:388–395.
- Atmaca G. 2004. Antioxidant effects of sulfur-containing amino acids. Yonsei Med J. 45:776–788.
- Berry CC, Wells S, Charles S, Curits AS. 2003. Dextran and albumin derivatised iron oxide nanoparticles: influence on fibroblasts in vitro. Biomaterials. 24:4551–4557.
- Bogunia-Kubik K, Sugisaka M. 2002. From molecular biology to nanotechnology and nanomedicine. Biosystems. 65:123–138.
- Borm PJA, Robbins D, Haubold S, Kuhlbusch T, Fissan H, Donaldson K, Schins R, Stone V, Kreyling W, Lademann J, et al. 2006. The potential risks of nanomaterials: a review carried out for ECETOC. Particle Fibre Toxicol. 14:1–35.
- Dang F, Kamada K, Enomoto N, Hojo J, Enpuku K. 2007. Sonochemical synthesis of the magnetite nanoparticles in aqueous solution. J Ceram Soc Japan. 115:867–872.
- Di Bona KR, Xu Y, Gray M, Fair D, Hayles H, Milad L, Montes A, Sherwood J, Bao Y, Rasco JF. 2015. Short- and long term effects of prenatal exposure to iron oxide nanoparticles: influence of surface charge and dose on developmental and reproductive toxicity. Int J Mol Sci. 16:30251–30268.
- Gawande MB, Velhinho A, Nogueira AD, Ghumman CAA, Teodoro OMND, Branco PS. 2012. A facile synthesis of cysteine ferrit magnetic nanoparticles for application in multicomponent reactions – a sustainable protocol. RSC Adv. 2:6144–6169.
- Gupta AK, Curtis AS. 2004. Surface modified superparamagnetic nanoparticles for drug delivery: interaction studies with human fibroblasts in culture. J Mater Sci Mater Med. 15:493–496.
- Hallberga L. 1987. Wheat fiber, phytates and iron absorption. Scand J Gastroenterol Suppl. 129:73–79.
- Harland BF. 1989. Dietary fibre and mineral bioavailability. Nutr Res Rev. 2:133–147.
- Hussain SM, Hess KL, Gearhart JM, Geiss KT, Schlager JJ. 2005. In vitro toxicity of nanoparticles in BRL 3A rat liver cells. Toxicol In Vitro. 19:975–983.
- Kim GB, Seo YM, ShinK S, Rhee AR, Han J, Paik IK. 2011. Effects of supplemental copper-methionine chelate and copper-soy proteinate on the performance, blood parameters, liver mineral content, and intestinal microflora of broiler chickens. J Appl Poul Res. 20:21–32.
- Kouchesfehami HM, Kiani S, Rostami AA, Fakheri R. 2013. Cytotoxic effect of iron oxide nanoparticles on mouse embryonic stem cells by MTT assay. Iran J Toxicol. 7:849–853.
- Lafleur MV, Woldhuis J, Loman H. 1980. Effects of sulphydryl compounds on the radiation damage in biologically active DNA. Int J Radiat Biol Relat Stud Phys Chem Med. 37:493–498.
- Layrisse M, Martinez-Torres C, Renzy M, Leets I. 1975. Ferritin iron absorption in man. J Blood. 45:689–698.
- Mohammadi H, Farzinpour A, Vaziry A. 2017. Reproductive performance of breeder quails fed diets supplemented with l-cysteine-coated iron oxide nanoparticles. Reprod Domest Anim. 52:298–304.
- Morris ER, Ellis R. 1976. Isolation of monoferric phytate from wheat bran and its biological value as an iron source to the rat. J Nutr. 106:753–760.
- Motzok I, Pennell MD, Davies MI, Ross HU. 1975. Effect of particle size on the biological availability of reduced iron. J Assoc Off Anal Chem. 58:99–103.
- Mukawevho E, Ferreria Z, Ayeleso A. 2014. Potential role of sulfur-containing antioxidant systems in highly oxidative environments. Molecules. 19:19376–19389.
- National Research Council – NRC. 1994. Nutrient requirements of poultry. Washington (DC): National Academy Press; p. 45.
- Nel A, Xia T, Madler L, Li N. 2006. Toxic potential of materials at the nanolevel. Science. 311:622–627.
- Nikonov IN, Folmanis YG, Folmanis GE, Kovalenko LV, Laptev GYu, Egorov IA, Fisinin VI, Tananaev IG. 2011. Iron nanoparticles as a food additive for poultry. Doklady Biol Sci. 440:328–331.
- Pekas LA. 1979. Propachlor detoxication in the small intestine: cysteine conjugation. J Toxicol Environ Health. 5:653–662.
- Saki AA, Abbasinezhad M, Rafati AA. 2014. Iron nanoparticles and methionine hydroxy analogue chelate in ovofeeding of broiler chickens. Int J Nanosci Nanotechnol. 10:187–196.
- Zimermann MB, Hilty FM. 2001. Nanocompounds of iron and zinc: their potential in nutrition. Nanoscale. 3:2390–2398.