Abstract
A feeding experiment was carried out to examine the effect of dietary sodium selenite (SS) supplementation on pigeon squabs. A total of 864 paired pigeons were randomly and equally distributed into four groups with three replicates. Each group was given a basal diet either unsupplemented (T1) or supplemented with 0.5, 1.0, or 1.5 mg/kg of SS in feed (T2–T4). Diet had no significant effect on the final body weight of squabs (P > 0.05). However, during days 8–14, body weight gain (BWG) in SS supplemented groups was lower than the control group (P < 0.05), while BWG was higher than controls from days 15–21 (P < 0.05). Squabs fed the basal diet without SS supplementation displayed lower selenium content in chest and leg muscle (P < 0.05). Glutathione peroxidase 4 (GPx4) mRNA levels were lower in liver, ovary and testis tissue in controls (P < 0.05), while growth hormone (GH) mRNA levels in liver were higher in controls (P < 0.05). These results suggest dietary SS supplementation had potential effect on growth performance during the later growth period (days 15–21). Furthermore, dietary SS supplementation increased the selenium content in muscle and affected the expression of GPx4 and GH.
The dietary selenium supplementation affected the body weight gain of the squabs.
Increasing selenium supplementation in diet improved the selenium content in muscle.
Increasing selenium supplementation in diet affected the expression of GPx4 and GH.
Highlights
Introduction
Selenium (Se) is an essential element required for normal growth and maintenance in human and other animals (Rayman, Citation2000; Choct et al. Citation2004). The NRC (1994) recommended minimum level of 0.15 mg/kg for selenium supplementation of broilers, however, the selenium deficiency syndromes were found in poultry industries. Walter and Jensen (Citation1963) indicated that the Se deficiency can be rapidly reversed and prevent by Se supplementation. Furthermore, Se is required for the active site of the Se-dependent enzyme GPx (Kohrle et al. Citation2005), and the synthesis of selenoproteins is affected by levels of Se supplementation (Zhang et al. Citation2013). GPx activity can serve as a biomarker of Se status in dose response studies, and can be used to indicate dietary Se requirements in aquatic animals (Gatlin and Wilson, Citation1984; Hilton et al. Citation1980; Lin and Shiau, Citation2005). Zoidis et al. (Citation2010) found that GPx4 mRNA levels in chicken are down-regulated by excess Se, but Weiss and Sunde (Citation2001) reported that transcript abundance of GPx4 in rat liver was not affected by dietary Se, Zhou et al. (Citation2009) also got similar conclusion in pigs.
Growth hormone (GH) is essential for postnatal growth. The release of GH from the anterior pituitary is believed to be under the reciprocal regulation of GH-releasing hormone and somatostatin (Frohman and Jansson, Citation1986; Strobl and Thoma, 1994). GH is regulated by the hypothalamic GH releasing factor, glucocorticoid, and thyroid hormones (Barinaga et al. Citation1983). Zhou and Wang (Citation2011) reported that Se supplementation affects the growth performance of chickens, while Yoon et al. (Citation2007) found that Se supplementation did not influence the growth performance of broilers.
Unlike chickens, pigeons pair for life, and newly hatched squabs are fed with crop milk from their parents via a system of milk feeding that is unique among birds (Silver, Citation1984). However, little is known about the effects of dietary Se supplementation on the growth performance of squabs, or on the expression of growth related-genes such as GH and GPx4. This study focused on the effects of dietary SS supplementation on the growth performance of squabs. The Se concentration in chest and leg muscle was measured, and GPx4 and GH expression levels were determined to explore the molecular mechanism of dietary Se.
Materials and methods
Ethics approval
This study was reviewed and approved by the Institutional Animal Care and Use Committee of the Department of Animal Science and Technology, Yangzhou University (SYXK(Su) IACUC 2012-0029). All experiments were performed in accordance with the Regulations for the Administration of Affairs Concerning Experimental Animals (China, 1988). All pigeon procedures were performed according to the Standards for the Administration of Experimental Practices (Jiangsu, China, 2008).
Animals and management
A total of 864 paired adult White King pigeons were obtained from the Tangshan Cuigu pigeon industry (Nanjing, China). They were randomly divided into four groups, each group contained three replicates (72 birds per replicate). Dietary treatment 1 (T1) did not include sodium selenite (SS) supplementation, and treatments T2–T4 were supplemented with 0.5 mg, 1.0 mg, and 1.5 mg/kg of SS in dry matter (DM), respectively (Table ). The basal diet contained 0.047mg/kg Se. The pigeons were fed and watered ad libitum. The experiment lasted for 60 days.
Table 1. Composition of basal diet.
Table 2. Composition and nutrient levels of the supplemental sand.
Body weight
The body weight (BW) of 30 same squabs from each replicate were recorded weekly (from day 1 to day 28). BW gain (BWG) was calculated by subtracting the BW recorded at the end of the previous week from the current weight.
Tissue collection
24 paired pigeons (24 female pigeons and 24 male pigeons) were selected randomly from the four treatments (2 paired pigeons from each replicate), anesthetized with sodium pentobarbital at a dosage of 2.5 mg/100 g BW, sacrificed, and the hypothalamic–pituitary–gonadal axis (HPG axis), liver, chest muscle and leg muscle were isolated. Tissues samples were collected at the same time of day (between 09:00 and 10:00 h) for all groups, and all efforts were made to minimize suffering. The samples of 10 g fresh chest muscle and leg muscle of squabs for Se content assay were stored at −20 °C until analysis. RNase-free tubes were used to collect 1 g liver, HPG axis and chest muscle of squabs for RT-PCR were immediately frozen in liquid nitrogen and stored at −80 °C until analysis.
Muscle selenium assay
The frozen chest muscle and leg muscle were thawed, about 1 g were weighed, digested, and analyzed for selenium content. The samples were digested with 10 mL acid mixture (4HClO4: 1HNO3) overnight. The mixed solutions were heated using an electric hot plate until the samples were completely digested. The solutions were heated until they were clear and colorless, and then the remaining solutions were diluted to 2 mL. After adding 5mL HCl (6 mol/L), the mixed solutions were further heated until HClO4 was smoking. The solutions were transferred to a 50 mL volumetic flask and fixed (Hao et al. 2004). The hydride generation atomic fluorescence spectrometry (AA6501, Shimadzu Ltd., Japan) was used to analyze the Se concentration in the samples (Wang and Li, Citation2011).
RT-PCR analysis
Total RNA was isolated using TRIzol, 1% agarose gelelectrophoresis and Thermo NanoDrop 2000 (Thermo, USA) were used to detect the integrity and quality of RNA. 1 μL total RNA was reverse-transcribed using the Fast Quant RT Kit (catalog numbers DP405 and KR106, respectively; TIANGEN Biotech Co., LTD, Beijing, China).
Expression of GPx4, GH and GAPDH were measured by real-time PCR with primers designed to amplify the coding region of the target gene (Table ) and the SuperReal PreMix reagent (SYBR Green; catalog number FP204; TIANGEN Biotech Co., Ltd.). The 20 µL reactions contained 10 μL of 2× SuperReal Premix, 0.4 μL of 50× ROX Reference Dye, 0.6 μL of each primer, 1 μL of cDNA, and 7.4 μL of ddH2O. PCR involved cDNA denaturation at 95 °C for 15 min, followed by 40 cycles of 95 °C for 10 s and 60 °C for 32 s. Assays were repeated independently three times.
Table 3. Primer sequences of target genes and GAPDH gene.
Statistical analysis
Data were expressed as means ± standard deviation (SD) and analyzed by one-way ANOVA using SPSS 13.0 (SPSS Inc., Chicago, IL). The significance of differences among the different groups was evaluated by the least significant difference post hoc multiple comparisons test. The linear and quadratic effects of SS concentration were assessed using orthogonal polynomials. The significance level was set at P < 0.05.
Results
Growth performance and selenium concentration analysis
The effect of dietary SS supplementation on the growth performance of squabs was investigated (Table ). The final BW of squabs supplemented with varying quantities of dietary SS showed no significant differences among the treatments (P > 0.05). The BW of 14-day-old squabs was higher in the control group (P < 0.05), but there were no significantly difference among supplemented groups (P > 0.05). However, squabs supplemented with SS had a higher BW at day 21 (P < 0.05). Furthermore, BW of 14-day-old squabs were linearly (P < 0.01) decreased as the level of SS increased, while the BW of 21-day-old squabs were linearly (P < 0.01) increased as the level of SS increased. The result showed that different SS supplementation dosages had no effect on the BW of squabs (P > 0.05) .
Table 4. Effect of dietary SS supplementation on the growth performance of squabs.
Between days 1 and 7, BWG was not significantly different among groups (P > 0.05; Table ), but BWG began to differ during the period of rapid growth, and peaked in groups without dietary SS supplementation during days 8–14 (P < 0.05). During days 15–21d, BWG was higher in groups supplemented with dietary SS (P < 0.05), SS supplementation linearly and quadratically (P < 0.01) increased. However, during days 22–28, BWG was not significantly different between the four groups (P > 0.05). It was obvious that the supplemental dosage had minor effect on the BWG of squabs.
Determination of the selenium concentration in chest and leg muscle (Table ) showed that the Se concentration increased with increasing SS dietary supplementation dosage, and the pattern was similar in both tissues. The Se concentration in both chest and leg muscle was significantly higher in supplemented groups than in the control group (P < 0.05), suggesting dietary SS supplementation increased the Se content in both tissues.
Table 5. Effects of dietary SS supplementation on muscle Se concentration of squabs (Means ± SD) (mg/kg).
Analysis of GPx4 and GH expression
RT-PCR was used to measure GPx4 and GH expression in various tissues following dietary SS supplementation. The results (Fig. ) showed that SS supplementation affected GPx4 mRNA levels in gonad and liver. In testis and liver, expression of GPx4 was higher in all SS supplemented groups than in T1 (P < 0.05), however, GPx4 mRNA levels in liver were higher in T4 than T2 (P < 0.05). In ovary, GPx4 expression was higher in T2 and T4 than in T1 (P < 0.05), but there were no significant difference among SS supplemented groups (P > 0.05). In hypothalamus, pituitary and chest muscle, GPx4 expression was similar among all treatments (P > 0.05).
Figure 1. Relative (2-ΔΔCT) of expression levels of GPx4 in tissues of squabs supplemented with dietary SS. Values marked with different letters on the bars are significantly different (P < 0.05).
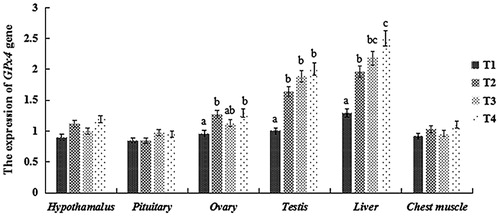
Figure 2. Relative (2-ΔΔCT) of expression levels of GH in tissues of squabs supplemented with dietary SS.
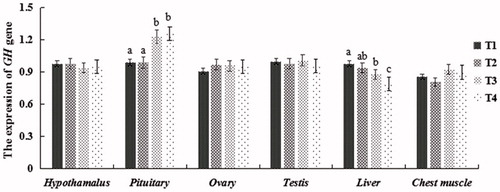
In pituitary, expression of GH was higher in T3 and T4 than in T1 and T2 (P < 0.05). However, in liver, the opposite was true; GH mRNA levels in T4 were significantly lower than in T1 and T2 (P < 0.05), while there were no significant difference in T2 and T3 (P > 0.05). Despite some minor fluctuation, there was no difference in GH expression among the four groups in hypothalamus, ovary, testis and chest muscle (P > 0.05).
Discussion
In nature, animals receive Se mainly from forages and grains, and the supplemental Se is mixed with basal diet in poultry industry (Surai, Citation2002; Ryu et al. Citation2005). The digestive system of animal, including chickens, has adapted to Se during evolution. The peroxidant actions of selenite inclusion in food animal diet can induce physiological activities (Surai, Citation2002), dietary Se supplementation has been widely used to improve the physiological status of fowls. The effects of dietary Se supplementation on growth performance and meat quality of various fowls, including chicken, duck, and quail have been reported (Choct et al. Citation2004, Wang et al. Citation2011). The results of this study revealed that dietary supplementation of SS did not have any impact on the final BW of squabs, in agreement with the findings of Yoon et al. (Citation2007) and Wang and Xu (Citation2008). Previous studies showed that supplemental Se did not affect BW, regardless of the feeding duration, that Edens et al. (Citation2000) fed broilers with basal diets containing 0.10 or 0.30 ppm SS for 42 days, Choct et al. (Citation2004) fed broilers with basal diets supplemented with 0.10 or 0.25 mg/kg SS for 38 days, and Dlouhá et al. (Citation2008) fed broilers with basal diets supplemented with 0.3 mg/kg SS for 42 days. However, Dlouhá et al. (Citation2008) also concluded that supplemented SS improved the BW of broilers at the age of 21 day, which suggests the effect of Se supplementation may be associated with the feeding duration. Furthermore, Surai (Citation2002) and Zuberbuehler et al. (Citation2006) concluded that the symptoms of Se deficiency observed in their studies were associated with the duration of experiments in rats and hens. Moreover, Wang et al. (Citation2011) concluded the effect of Se is dependent on species and dosage. Ort and Latshaw (Citation1978) suggested that a basal diet supplemented with 5 ppm of SS was not toxic to the laying chicken, Moksnes (Citation1983) found that the White Leghorn chickens were fed a basal diet containing 0.30 mg mg/kg of SS supplemented with 6.0 mg/kg of SS for 18 weeks, no toxic effect were observed. However, Spallholz and Hoffman (Citation2002) found that SS supplementation above 4–5 μg/g in the diet resulted in toxicity in aquatic birds, suggesting the dosage of dietary SS used in the present study maybe was within the permissible level.
Milk, eggs and meat are staple foods for humans, and all are a potential source of dietary Se intake (Gibson, Citation1990; Givens et al. Citation2004). Zhou and Wang (Citation2011) demonstrated that the Se content in muscle was significantly increased by dietary supplementation of Se, in accordance with our current results. Pan et al. (Citation2007) and Payne and Southern (Citation2005) also reported an increase in breast Se content in chicken fed with selenomethionine or Se-yeast. Sevcikova et al. (2006) and Wang et al. (Citation2011) suggested that the supplement of Se in the diet for broiler increased the microelement concentration in muscle. Pigeon meat has grown popularity in China and it is therefore a potential source of Se, Gibson (Citation1990) also recommended muscle meat as a a dependable dietary source of Se.
Se is required for sperm motility, and GPx4 is believed to shield developing sperm cells from oxidative damage, since it is polymerized into a structural protein in mature spermatozoa (Scott et al. Citation1998; Iwanier and Zachara, Citation1995). Previous studies reported that dietary Se has no effect on GPx4 mRNA levels in the liver and testis of pigs and rats (Zhou et al. Citation2009; Sachdev and Sunde, Citation2001). However, our results showed that expression of GPx4 in liver and testis increased with increasing dietary SS dosage. Zoidis et al. (Citation2010) found that liver GPx4 mRNA levels in chickens were highest following supplementation with 0.15 ppm Se. Zhang et al. (Citation2013) also pointed out that GPx4 mRNA levels were higher following 0.1 and 0.4 mg/kg Se supplementation of basal diet and lower following treatment with excess Se. Gan et al (Citation2002) also reported higher GPx4 mRNA levels following consumption of a diet supplemented with 20 μg Se/kg of diet, and similar expression levels in control animals and those fed with 40 μg Se/kg of diet. These results indicated that GPx4 mRNA levels in tissues maybe were related to the dosage of supplemental Se.
GH is synthesized in the somatotroph cells of the anterior pituitary and released under the control of two hormones produced in the hypothalamus (Dierickx and Vandesande, Citation1979; Jacobowitz et al. Citation1983). Growth hormone secretagogues (GHS) are a class of drugs that are believed to regulate GH (Howard et al. Citation1996), and Guan et al. (Citation1997) reported that the hypothalamus, hippocampal formation and pituitary are the regions with the highest expression of GHS-R mRNA. In pituitary, GH mRNA levels were higher in the 1.0 mg and 1.5 mg SS supplementation groups, suggesting dietary SS supplementation affected GH expression, but there was no significant difference in body weight. Adams et al. (Citation1990) identified at least one liver-specific GH receptor transcript in sheep, and Gluckman et al. (Citation1983) found that GH-dependent somatic growth was closely correlated with the appearance in the liver of an increasing number of high-affinity receptors for GH (Freemark et al. Citation1986). Our current results showed that expression of GH mRNA was low in the liver of squabs fed a diet supplemented with SS, as indicated by the slow growth of birds at day 28. Furthermore, higher GH mRNA levels in the pituitary were accompanied by lower GH mRNA levels in the liver of squabs supplemented with SS. This interesting observation is clearly worthy of further investigation.
Conclusion
In conclusion, dietary SS supplementation promoted BWG during the later growth period (days 15–21), but had no effect on the final weight of squabs at 28 days of age. The Se content in chest muscle and leg muscle tissue was higher with increasing dosage of dietary SS, which is important because pigeon meat is a potential source of dietary Se for humans. GPx4 mRNA levels in liver and testis also appeared to be correlated with the dosage of Se supplementation, whereas GH mRNA levels were high in the pituitary but low in the liver of squabs supplemented with SS. Further studies are needed to explain these observations.
Disclosure statement
The authors report no conflicts of interest. The authors alone are responsible for the content and writing of this article.
Additional information
Funding
References
- Adams TE, Baker L, Fiddes RJ, Brandon MR. 1990. The sheep growth hormone receptor: molecular cloning and ontogeny of mRNA expression in the liver. Mol Cell Endocrinol. 73:135–145.
- Akulov AV, Minina LA, Andreev MN, Tomskikh YUI, Bronnikova KA, Korenkov, I. 1972. Experimental selenium poisoning in hens. Sel kkokhozyaistvennaya Biologiya. 7:430–436.
- Barinaga M, Yamonoto G, Rivier C, Vale W, Evans R, Rosenfeld MG. 1983. Transcriptional regulation of growth hormone gene expression by growth hormone-releasing factor. Nature. 306:84–85.
- Bates JM, Spate VL, Morris JS, St Germain DL, Galton VA. 2000. Effects of Selenium Deficiency on Tissue Selenium Content, Deiodinase Activity, and Thyroid Hormone Economy in the Rat during Development 1. Endocrinology. 141:2490–2500.
- Choct M, Naylor AJ, Reinke N. 2004. Selenium supplementation affects broiler growth performance, meat yield and feather coverage. Brit. Poult. Sci. 45:677–683.
- Davis RH, Fear J, Winton AC. 1996. Interactions between dietary selenium, copper and sodium nitroprusside, a source of cyanide in growing chicks and laying hens. Brit. Poult. Sci. 37:87–94.
- Dierickx K, Vandesande F. 1979. Immunocytochemical localization of somatostatin -containing neurons in the rat hypothalamus. Cell Tissue Res. 201:349–359.
- Dlouhá G, Sevcikova S, Dokoupilová A, Zita L, Heindl J, Skrivan M. 2008. Effect of dietary selenium sources on growth performance, breast muscle selenium, glutathione peroxidase activity and oxidative stability in broilers. Czech J. Anim. Sci. 53:265–269.
- Edens FW, Carter TA, Parkhurst CR, Sefton AE. 2000. Effect of selenium source and litter type on broiler feathering. J. Appl. Poult. Res. 9:407–413.
- Freemark M, Comer M, Handwerger S. 1986. Placental lactogen and GH receptors in sheep liver: striking differences in ontogeny and function. Am J Physiol-Endoc M. 251:328–333.
- Frohman LA, Jansson JO. 1986. Growth hormone-releasing factor. Endocr Rev. 7:223–253.
- Gan L, Liu Q, Xu HB, Zhu YS, Yang XL. 2002. Effects of selenium overexposure on glutathione peroxidase and thioredoxin reductase gene expressions and activities. Biol Trace Elem Res. 89:165–175.
- Gatlin DM, Wilson RP. 1984. Dietary selenium requirement of fingerling channel catfish. J Nutr. 114:627–633.
- Gibson RS. 1990. Principles of Nutritional Assessment. Oxford University Press, New York, NY.
- Givens DI, Allison R, Cottrill B, Blake JS. 2004. Enhancing the selenium content of bovine milk through alteration of the form and concentration of selenium in the diet of the dairy cow. J Sci Food Agr. 84:811–817.
- Gluckman PD, Butler JH, Elliot TB. 1983. The ontogeny of somatotropic binding sites in ovine hepatic membranes. Endocrinology. 112:1607–1612.
- Guan XM, Yu H, Palyha OC, McKee KK, Feighner SD, Sirinathsinghji DJ, Howard AD. 1997. Distribution of mRNA encoding the growth hormone secretagogue receptor in brain and peripheral tissues. Mol Brain Res. 48:23–29.
- Hao X, Ling Q, Hong F. 2014. Effects of dietary selenium on the pathological changes and oxidative stress in loach (Paramisgurnus dabryanus). Fish Physiol. Biochem. 40:1313–1323.
- Hill KE, Zhou J, McMahan WJ, Motley AK, Atkins JF, Gesteland RF, Burk RF. 2003. Deletion of selenoprotein P alters distribution of selenium in the mouse. J Biol Chem. 278:13640–13646.
- Hilton JW, Hodson PV, Slinger SJ. 1980. The requirement and toxicity of selenium in rainbow trout (Salmo gairdneri). J Nutr. 110:2527–2535.
- Howard AD, Feighner SD, Cully DF, Arena JP. 1996. A receptor in pituitary and hypothalamus that functions in growth hormone release. Science. 273:974.
- Iwanier K, Zachara BA. 1995. Selenium supplementation enhances the element concentration in blood and seminal fluid but does not change the spermatozoal quality characteristics in subfertile men. J Androl. 16:441–447.
- Jacobowitz DM, Schulte H, Chrousos GP, Loriaux DL. 1983. Localization of GRF-like immunoreactive neurons in the rat brain. Peptides. 4:521–524.
- Juniper DT, Phipps RH, Jones AK, Bertin G. 2006. Selenium supplementation of lactating dairy cows: effect on selenium concentration in blood, milk, urine, and feces. J Dairy Sci. 89:3544–3551.
- Kohrle J, Jakob F, Contempre B, Dumont JE. 2005. Selenium, the thyroid, and the endocrine system. Endocr Rev. 26:944–984.
- Lin YH, Shiau SY. 2005. Dietary selenium requirements of juvenile grouper, Epinephelus malabaricus. Aquaculture. 250:356–363.
- Moksnes K, Norheim G. 1982. Selenium concentrations in tissues and eggs of growing and laying chickens fed sodium selenite at different levels. Acta Vet. Scand. 23:368–379.
- Moksnes K. 1983. Selenium deposition in tissues and eggs of laying hens given surplus of selenium as lenomethionine. Acta Vet. Scand. 24:34–44.
- Ort JF, Latshaw JD. 1978. The toxic level of sodium selenite in the diet of laying chickens. J Nutr. 108:1114–1120.
- Pan C, Huang K, Zhao Y, Qin S, Chen F, Hu Q. 2007. Effect of selenium source and level in hen's diet on tissue selenium deposition and egg selenium concentrations. J Agr Food Chem. 55:1027–1032.
- Payne RL, Lavergne TK, Southern LL. 2005. Effect of inorganic versus organic selenium on hen production and egg selenium concentration. Poult. Sci. 84:232–237.
- Payne RL, Southern LL. 2005. Comparison of inorganic and organic selenium sources for broilers. Poult. Sci. 84:898–902.
- Rayman MP. 2000. The importance of selenium to human health. The lancet. 356:233–241.
- Roveri A, Casasco A, Maiorino M, Dalan P, Calligaro A, Ursini F. 1992. Phospholipid hydroperoxide glutathione peroxidase of rat testis. Gonadotropin dependence and immunocytochemical identification. The Journal of Biological Chemistry. 267:6142–6146.
- Ryu YC, Rhee MS, Lee KM, Kim BC. 2005. Effects of different levels of dietary supplemental selenium on performance, lipid oxidation, and color stability of broiler chicks. Poult. Sci. 84:809–815.
- Sachdev SW, Sunde RA. 2001. Selenium regulation of transcript abundance and translational efficiency of glutathione peroxidase-1 and -4 in rat liver. Biocheml J. 357:851–858.
- Scott R, MacPherson A, Yates RWS, Hussain B, Dixon J. 1998. The eVect of oral selenium supplementation on human sperm motility. Br J Urol. 82:76–80.
- Ševčíková S, Skřivan M, Dlouhá G, Koucký M. 2006. The effect of selenium source on the performance and meat quality of broiler chickens. Czech J Anim Sci. 51:449–457.
- Silver R. 1984. Prolactin and parenting in the pigeon family. J Exp Zool. 232:617–625.
- Spallholz JE, Hoffman DJ. 2002. Selenium toxicity: cause and effects in aquatic birds. Aquat Toxicol. 57:27–37.
- Strobl JS, Thomas MJ. 1994. Human growth hormone. Pharmacol Rev. 46:1–34.
- Surai PF. 2002. Natural antioxidants in avian nutrition and reproduction. Nottingham University Press, Nottingham.
- Walter ED, Jensen LS. 1963. Effectiveness of selenium and noneffectiveness of sulfur amino acids in preventing muscular dystrophy in the turkey poult. J Nutr. 80:327–331.
- Wang BW, Guoqing H, Qiaoli W, Bin Y. 2011. Effects of yeast selenium supplementation on the growth performance, meat quality, immunity, and antioxidant capacity of goose. J Anim Physiol Anim Nutr. 95:440–448.
- Wang Y, Li J. 2011. Effects of chitosan nanoparticles on survival, growth and meat quality of tilapia, Oreochromis nilotica. Nanotoxicology. 5:425–431.
- Wang Y, Zhan X, Zhang X, Wu R, Yuan D. 2011. Comparison of different forms of dietary selenium supplementation on growth performance, meat quality, selenium deposition, and antioxidant property in broilers. Biol Trace Elem Res. 143:261–273.
- Wang YB, Xu BH. 2008. Effect of different selenium source (sodium selenite and selenium yeast) on broiler chickens. Anim Feed Sci Tech. 144:306–314.
- Weiss SS, Sunde A. 2001. Selenium regulation of transcript abundance and translational efficiency of glutathione peroxidase-1 and -4 in rat liver. Biochem. J. 357:851–858.
- Yoon I, Werner TM, Butler JM. 2007. Effect of source and concentration of selenium on growth performance and selenium retention in broiler chickens. Poult. Sci. 86:727–730.
- Zhang Q, Chen L, Guo K, Zheng L, Liu B, Yu W, Tang Z. 2013. Effects of different selenium levels on gene expression of a subset of selenoproteins and antioxidative capacity in mice. Biol Trace Elem Res. 154:255–261.
- Zhou JC, Zhao H, Li JG, Xia XJ, Wang KN, Zhang YJ, Lei XG. 2009. Selenoprotein gene expression in thyroid and pituitary of young pigs is not affected by dietary selenium deficiency or excess. J Nutr. 139:1061–1066.
- Zhou X, Wang Y. 2011. Influence of dietary nano elemental selenium on growth performance, tissue selenium distribution, meat quality, and glutathione peroxidase activity in Guangxi Yellow chicken. Poult. Sci. 90:680–686.
- Zoidis E, Pappas AC, Georgiou CA, Komaitis Ε, Feggeros K. 2010. Selenium affects the expression of GPx4 and catalase in the liver of chicken. Comp Biochem Phys B. 155:294–300.
- Zuberbuehler CA, Messikommer RE, Arnold MM, Forrer RS, Wenk C. 2006. Effects of selenium depletion and selenium repletion by choice feeding on selenium status of young and old laying hens. Physiol Behav. 87:430–440.