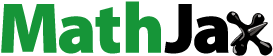
Abstract
Mushroom waste compost is the main byproduct when cultivating mushrooms. Containing many mycelia, it has a positive effect on animal health but its high fibre content may be detrimental to growth. In order to reduce the environmental problems caused by mushroom waste compost and possibly increase the use of agriculture byproducts, this study aimed to investigate the effects of replacing broiler feed with Pennisetum purpureum schum compost (PWMC) and its effect on meat quality and fat metabolism. Totally 240 broilers were used and allocated to 4 different treatments, including control group, 5% PWMC, Saccharomyces cerevisiae fermented PWMC (FPW) and Pennisetum purpureum schum (PP). Each treatment had 3 replicates and 20 broilers in a pen. The results show that replacing 5% broiler feed with PWMC, FPW and PP decreased the depth of subcutaneous fat in broilers from 3.6 to 2.6, 2.7 and 2.8 mm, respectively (p = .0132). Furthermore, adipolysis mRNA expression increased in all treatment groups, slightly increasing breast and thigh meat accumulation. Overall, mushroom waste compost at 5% in broiler diets could improve the body composition of broilers and adipolysis-related mRNA expression.
High-fibre agricultural by-products can replace part of the broiler diet without affecting its growth performance.
High-fibre agricultural by-products can decrease broiler subcutaneous fat accumulation.
High-fibre agricultural by-products can enhance fat metabolism-related mRNA expression.
Highlights
Introduction
Currently, the main sources of animal feed ingredients are crops, such as corn, wheat and soybeans (Eshel et al. Citation2014). For every 1000 kcals of animal protein produced, about 2.4 m3 of water, 34 m2/year of land and at least 4 kg feed must be consumed (Eshel et al. Citation2014). However, the inevitable by-products of crop production are often not effectively used. Common agricultural by-products include wheat bran, bean hull, rice bran, straw, and corn stalks, olive cake and prosopis pod (El-Ghamry et al. Citation2005; Eshel et al. Citation2014; Jones et al. Citation2019; Al-Harthi et al. Citation2019a, Citation2020; Hassan et al. Citation2020). At present, many agricultural by-products have been incinerated, buried or used as adjusting materials for poultry and livestock compost. However, in response to the shortage of food crops, people have become increasingly interested in high-fibre agricultural by-products. Because agricultural by-products usually have characteristics of more crude fibres, some people also use agricultural by-products as medium for the cultivation of fungi (such as mushrooms) (Jones et al. Citation2019).
Furthermore, Pennisetum is one of the common forage species, which can also be used as a medium for mushroom cultivation. However, many mushroom wastes compost will still be produced after the cultivation of mushrooms and cause new environmental problems (Wang et al. Citation2017). In addition, high-fibre agricultural by-products may promote the growth of moulds under the improper storage environment and bring the risk of mycotoxins. On the other hand, in the past, in order to pursue the growth efficiency of livestock animals, the diet of livestock animals usually contains lower fibre concentration (Buxton and Redfearn Citation1997). However, recent studies have repeatedly shown that appropriate amount of fibre supplementation has considerable benefits for animal health (Desai et al. Citation2016) and does not necessarily reduce animal growth performance (Teng et al. Citation2017; Nassar et al. Citation2019). In addition, it seems that adding fibre to animal diets can also improve animal body composition (Nassar et al. Citation2019), may be due to change in lipid metabolism (Al-Harthi et al. Citation2019a, Citation2019b).
In order to address the possible harm caused by high levels of indigestible fibre or improper storage of fibres, probiotics can be used to digest the fibres in the in vitro and increase the content of digestible fibres and reduce the possibility of toxin harm (Aguilar-Toalá et al. Citation2018). In order to contribute to the environment while effectively using resources, and to mitigate the harm caused by over-growing the feed ingredients required for livestock animals, our team has been working for many years to find new possibilities for agricultural by-products. In the past few years, we have pointed out the potential use of waste mushroom space bags (Wang et al. Citation2017), and also used probiotic fermentation to find new ways for the use of wheat bran (Teng et al. Citation2017; Lin et al. Citation2018; Chuang et al. Citation2019) and rice straw (Pan et al. Citation2018). However, few researches reported the effects and principles of high-fibre agricultural by-product on animal body composition change. It is suggested that the increase fibre content in broilers feed could enhance the adipose metabolism and increase some endocrines, such as oxytocin, secreted to improve muscle growth in broilers. This report therefore aims to investigate the effect of high-fibre feeds replacement in poultry feeds on the poultry body composition and find a new way of high fibre agriculture by-product utilisation.
Materials and methods
The collection and fermentation of pennisetum purpureum schum no.2 (PP) and PP waste mushroom compost (PWMC)
PWMC contained at least 70% PP and was the leftover medium from Pleurotus eryngii cultivation. All mature Pleurotus eryngii mushrooms were removed. PWMC was provided by the Taiwan Agricultural Research Institute Council of Agriculture, and the dried PP was provided by the Livestock Research Institute in Taiwan. Both dried PP and PWMC were stored at 4 °C until use. Saccharomyces cerevisiae fermented PWMC (FPW) was created by using S. cerevisiae to ferment PWMC. Briefly, 100 mL 108 CFU/mL S. cerevisiae broth was added to 1 kg PWMC, moisture was adjusted to 60%. Samples were fermented at 30 °C for 3 days and then dried at 50 °C for 1 day. FPW was collected and refrigerated at −20 °C until use.
Animal experiment design
PWMC, FPW and PP were used to identify the effect of PWMC on broilers growth performance, fat metabolism, nutrient absorption and carcase characteristics. This experiment was carried out at National Chung Hsing University (NCHU) in Taiwan and all protocols were as per the Animal Care and Use Committee at NCHU (IACUC: 108-049). The animal design and tests for serum characteristics and mRNA expression were slightly modified, as per Chuang et al. (Citation2019). Briefly, 240 1-day-old male Ross 308 broilers were divided into 4 treatment groups, basal diet (control), 5% PWMC, 5% FPW and 5% PP, for a 35-day experiment. Each group had 60 broilers with 3 replicates (20 broilers for each pen, and each pen is about 3.24 m2). The initial body weights of the chicks were similar (48.0 0.7 g/bird) among all groups. Temperature was kept at 33 °C for 1-day-old chicks then slowly decreased to 22 °C after 30 days. To achieve or exceed the NRC 1994 nutrient requirement for broilers, all experimental diets were calculated and the proximate composition was analysed with six replicates according to the AOAC (Citation2012) to determine the crude protein, crude fibre and crude fat levels (Table ). The starter diet was given to chickens before 21 days, and the finisher diet given from days 22 to 35. Water and feed were ad libitum during the whole rearing period. Body weight and feed intake were measured on days 21 and 35, and the feed conversion rate and body weight gain were calculated according to the data of body weight and feed intake. The ileum, abdominal fat and liver were collected from the 35-day-old broilers for qPCR analysis.
Table 1. Composition and calculated analysis (g/kg as fed) of the basal and experimental diet for broilers, starter diet (1–21 days) and finisher diet (22–35 days), respectivelyTable Footnote1.
Meat quality
Carcase handling
Thirty-six 35-day-old broilers (3 of each pen, 9 per treatment) were weighed (live weight, LW) and exsanguinated after stunning. The chicken bodies were soaked in 62 °C water for 1 min before the feathers were removed by depilatory machinery for 1 min. The organs and abdominal fat were removed before the hot carcase (HC) and abdominal fat (AF) were individually weighed. The subcutaneous fat (ScF) was determined by the skin thickness on the caudal vertebra. The hung carcases were refrigerated at 4 °C for 1 day and the colour and pH values were recorded by colour metre and pH metre. The dressing rate was calculated using the ratio of HC to LW. The carcase was cut into several parts, inner and outer breast, drumstick, thigh, wing, drumette, and wingette, and the weight of every part was recorded. The carcase percentage was also calculated based on the ratio of cut parts to carcase.
Water holding capacity
The methods of determining water holding capacity (WHC) were described by Bowker and Zhuang (Citation2015). The WHC can be separated into three parts, thawing loss (TL), drip loss (DL) and cooking loss (CL). Briefly, water on the breast meat (one of each pen and three for each treatment in TL and DL, two of each pen and six for each treatment for CL) was wiped off and the weight was recorded before treatment. To measure TL, the samples were frozen at −20 °C for 1 day then thawed at 4 °C before their weight was recorded. To measure DL, the samples were hung in a 4 °C refrigerator for another day before their weight was recorded. For CL, the samples were cooked for 40 min in 95 °C water before weights were recorded. All WHC measurements were calculated by the following formula:
Shearing force
The Dynamic Mechanical Analyser (Compact-100, Sun Rheo Metre, Japan) was used to measure shearing force. Briefly, six replicates of post-CL breast meat were used. Each portion of meat was cut into 1 cm 1 cm
1 cm cubes and put on the Dynamic Mechanical Analyser, according to the manufacturer’s protocol. After measurement, the shearing force data were recorded.
Organoleptic evaluation
The methods for the sensory tests were according to Toomer et al. (Citation2019). Briefly, breast meat (two of each pen, six for each treatment) was cooked at 95 °C for 40 min and then divided into four replicates. To carry out the sensory test, seven professionally trained subjects evaluated the samples on the following criteria: colour, odour, flavour, texture and total acceptance. The score for each criterion was from 1 to 7, from the least favourite to the most favourite.
Serum characteristics
At the end of the animal experiment (35-day-old), the 5 mL blood of all the broilers was collected in an air-conditioned laboratory (25 °C) and left to stand at 4 °C for 4 to 5 h. The samples were centrifuged at 3000 xg for 10 min to separate the blood cells and serum. The serum was then frozen at −20 °C until use. The concentrations of oxytocin (OXT) (Wuhan Fine Biotech Co., Ltd.), adiponectin (CUSA Bio., Wuhan, Hubei, China), corticosterone (Cayman Chemical Co., Ltd. Ann Arbour, MI, USA), and malondialdehyde (MDA) (Cayman Chemical Co., Ltd. Ann Arbour, MI, USA) in the broiler serum were measured. All analysis methods followed the manufacturer’s protocol. Briefly, the protein analytes bind to the antibody and the enzyme-linked immunosorbent assay reader is used to measure absorbance at key wavelengths.
Total RNA isolation and qPCR
The total RNA isolation and qPCR were measured to determine mRNA expression in liver, ileum and abdominal fat. mRNA was collected from 35-day-old broilers in each treatment group. The methods of total mRNA isolation followed the manufacturer’s protocol (SuperScript™ FirstStrand Synthesis System reagent, Invitrogen, Waltham, MA, USA). mRNA purity was determined by the absorbance ratio of 260/280 nm, and the methods of cDNA synthesis and qPCR analysis followed Chuang et al. (Citation2019). Briefly, cDNA was mixed with 2× SYBR GREEN PCR Master Mix-ROX (Gunster Biotech, Co., Ltd), deionised water, and each primer at a ratio of 5:1.2:1.8:1. The StepOnePlus™ Real-Time PCR System (Thermo Fisher, Waltham, MA, USA) was used to detect qRT-PCR performance, the 2−△△Ct method was used to calculate the relative mRNA expression level, and ß-actin was used as the housekeeping gene for normalisation. All primer sequences matched the genes of Gallus gallus (chicken) from Genbank.
Statistical analysis
The data collected were statistically analysed using general linear models procedure of SAS software (SAS® 9.4, 2018) following a Completely Randomised Design (CRD). Data on the dietary treatments were subjected to analysis of variance using Statistical Analysis System Institute Package (SAS) and the mean values were compared using the Tukey test with the significant level at p < .05.
The mathematic model was:
where Yij is the measurement on average of birds in pen j, dietary treatment i, µ is the overall mean, Di is the fixed effect of dietary treatment i, εij is the residual term that εij ∩ N (0, σ2ε).
Results
Broilers growth performance and carcass and characteristics
The initial body weight of broilers used in every treatment was around 48 g. The growth performance of each treatment show no significantly different both in the starter stage (1–21 days old) and the finisher stage (22–35 days old) (Table ).
Table 2. Broiler growth performances on different periods and different treatmentsTable Footnote1.
As the data shows in Table , the LW of 35-day-old broilers was significantly increased in the PWMC group (p = .0346). Although there was no significant difference in dressing rate or abdominal fat, ScF was decreased in all treatment groups, no matter the fibre type (Table ). There were no significant differences in carcase site percentage for all treatments (Table ). After 1 day of storage at 4 °C, there were no significant differences in the pH values for breast or thigh meat (Table ). When analysing meat colour, only the thigh meat in the PWMC group was lighter than the control (p = .015) (Table ). As summarised in Table , there were no significant differences among the treatment groups for WHC, CL, TL, or DL. However, the shearing force increased about 1.5 and 2 times in the PWMC and FPW groups, respectively (p < .0001). There was no significant difference in the MDA or corticosterone content in the broilers serum, but corticosterone levels were slightly decreased in the FPW and PP groups (Table ). OXT and adiponectin increased significantly in the high fibre groups, from 2 to 5 times higher for OXT (p = .0005) and 1.3 to 2 times higher (p = .0011) for adiponectin (Table ).
Table 3. Effect of PWMC, FWP and PP replacement in diet on Carcase characteristic of 35-day-old broilersTable Footnote1.
Table 4. Effect of PWMC, FWP and PP replacement in diet on pH value and meat colour on broiler breast or thigh at 1-day post-slaughterTable Footnote1.
Table 5. Effect of PWMC, FWP and PP replacement in diet on water holding capacities and shearing force of 35-day-old broiler breastTable Footnote1.
Table 6. Effect of PWMC, FWP and PP replacement in diet on serum characteristic of 35-day-old broilerTable Footnote1.
Organoleptic evaluation
The organoleptic evaluation indicators included colour, odour, flavour, texture and total acceptance. There were no significant differences in the scores of all measured indicators, for which the respective p values were .8694, .6444, .5856, .3725 and .5697. However, the total acceptance was slightly increased in all of the high fibre groups, from 6.59 for the control to 6.71, 6.75 and 6.77 for the PWMC, FPW and PP groups, respectively (Table ).
Table 7. Effect of PWMC, FWP and PP replacement in diet on organoleptic evaluation of 35-day-old broilers breastTable Footnote1.
Adipolysis-related and nutrient absorption-related mRNA expression
As the results show in Figure , potassium channel tetramerisation domain-containing 15 (KCTD15) and adiponectin mRNA expression, both of which are negatively correlated to the degree of animal obesity, significantly increased in liver and abdominal adipose tissue (p < .0001). In the liver, the type of fibre used for supplementation did not have a significant impact on the increase in KCTD15 and adiponectin. In the abdominal adipose tissue however, the PP group had about five times higher KCTA15 and four times higher adiponectin mRNA expression than the control group. In the adipolysis-related mRNA, the adipose triglyceride lipase (ATGL), ATP citrate synthase, and carnitine palmitoyltransferase I (CPT1) levels increased significantly in both the liver cells and adipocytes (p = .0057 and <.0001, respectively) of the high fibre groups (Figure ). There was a compensatory increase in adipogenesis-related mRNA, specifically the fatty acid synthase (FAS), fatty acid binding protein 4 (FABP4), 5′-AMP-activated protein kinase catalytic subunit alpha-2 (AMPK-α2) mRNA expression in liver cell and FAS, peroxisome proliferator-activated receptor alpha (PPAR-α), and peroxisome proliferator-activated receptor gamma (PPAR-γ) mRNA expression. Both AMPK-α2 and CCAAT-enhancer-binding protein (CEBP) mRNA expression decreased in the high fibre treatment groups (p < .0001). Interleukin-6 (IL-6) mRNA expression, which is positively correlated to adipocyte inflammation and can promote the rapid accumulation of adipose, was significantly decreased in the adipocytes of the PWMC and PP treatment groups (p < .0001) (Figure ).
Figure 1. Adipolysis and adipogenesis-related mRNA expression in liver (L) and abdominal fat (F) on 35-day-old broilers. (A, B) Potassium channel tetramerisation domain-containing 15 (KCTD15) and adiponectin express in liver and abdominal fat, respectively. (C, D) Adipose triglyceride lipase (ATGL), ATP citrate synthase (ATPCL) and carnitine palmitoyltransferase I (CPT1) express in liver and abdominal fat, respectively. (E, F) Fatty acid synthase (FAS), fatty acid binding protein 4 (FABP4), CCAAT-enhancer-binding proteins (CEBP) and 5′-AMP-activated protein kinase catalytic subunit alpha-2 (AMPK-α2) express in liver and abdominal fat, respectively, and peroxisome proliferator-activated receptor alpha (PPAR-α), peroxisome proliferator-activated receptor gamma (PPAR-γ) and interleukin-6 (IL-6) express in abdominal fat. a,b,c,dMeans within the same rows without the same superscript letter are significantly different (p < .05).

Conversely, all nutrient absorption-related mRNA, including excitatory amino acid transporter 3 (EAAT 3), free fatty acid receptor 2 (FFAR 2), glucose transporter 2 (GLUT 2), sodium-dependent glucose cotransporters 1 (SGLT 1) and peptide transporter 1 (PEPT 1), showed a 5–15 times increase in expression in the high fibre treatment groups. This was especially evident in EAAT3 and FFAR2 mRNA expression in the FPW group (p < .0001) (Figure ).
Figure 2. Nutrient absorption-related mRNA expression in ileum (ILE) on 35-day-old broilers. Excitatory amino acid transporter 3 (EAAT 3), free fatty acid receptor 2 (FFAR 2), glucose transporter 2 (GLUT 2), sodium-dependent glucose cotransporters 1 (SGLT 1) and Peptide transporter 1 (PEPT 1). a,b,cMeans within the same rows without the same superscript letter are significantly different (p < .05).

Discussion
It is estimated that there are at least 200 edible fungi worldwide, including mushroom, and a lot of kind of white rot fungi, such as Pleurotus eryngii (Wang et al. Citation2017). However, because of its high fibre content, mushroom wastes compost was not considered to be used as animal feed in the past, so it is usually treated by incineration or composting. However, this may cause harm to the environment and cause the wastes of resources (Buxton and Redfearn Citation1997). In addition, because of the traditional recognition of low-energy, high-fibre feed ingredients in animal feed, animal breeder are usually rarely willing to increase the fibre content of animal diets (Buxton and Redfearn Citation1997). However, previous studies have repeatedly shown that the addition of high fibre is beneficial to animal health (Desai et al. Citation2016; Teng et al. Citation2017; Hassan et al. Citation2020). In addition to increasing the strength of the animal’s intestinal barrier, high fibre also has a relief effect on the animal’s inflammatory response (Zou et al. Citation2018).
Although the effect of fibre addition on animal gut barrier has been reported for several times, there are few researches about how the fibre addition could alter the nutrient absorption-related mRNA expression. There are several types of transporters on the intestinal epithelial cells and can transfer specific substrate as well as the nutrient. In animals intestine, EAAT3 and PEPT-1 are the major peptide-related transporters . The increase of EAAT3 and PEPT1 can make the host have higher protein absorption efficiency (Madsen and Wong Citation2011; Bjørn-Yoshimoto and Underhill Citation2016). Among them, the expression of EAAT3 is more related to increasing the antioxidant capacity of animals and the health of nerve cells (Bjørn-Yoshimoto and Underhill Citation2016). Madsen and Wong (Citation2011) also pointed out that the expression of PEPT1 in the intestine is positively correlated with PPARα and will increase the adipolysis while fasting. FFAR2 is one of the receptors for short chain fatty acids (SCFAs) transport (Bartoszek et al. Citation2020). PWMC is the waste compose of mushroom, which may content lots of SCFAs when mushroom planted (Sande et al. Citation2019). It is also well known that fibre fermented by Saccharomyces cerevisiae will produce high content of SCFAs. However, the SCFAs content in PP is much lower than PWMC and FPW. Therefore, the FFAR2 mRNA expression in PWMC and FPW is much higher than control and PP groups, which means the intestinal epithelial cells can absorb more SCFAs in PWMC and FPW groups than in control and PP groups. GLUT 2 and SGLT1 are two different type of glucose transporter (Kalantar et al. Citation2019). The main glucose transporter in mammal is glucose transporter type 4 (GLUT 4), however, because the absent of GLUT 4 in poultry, GLUT 2 become one of the main glucose transporters in poultry, and is insulin sensitive (Braun and Sweazea Citation2008). SGLT1 is a transporter which can transport glucose by secondary active transport (Kalantar et al. Citation2019). The increase of nutrient absorption-related mRNA expression especially on PWMC and FPW groups may because of the enzyme produce during the P. eryngii or S. cerevisiae fermentation and both increase expression of GLUT2 and SGLT1 will improve glucose absorption efficiency. Kalantar et al. (Citation2019) also indicated that increase complex enzyme (1000 U/g phytase and 480 U/g multi-glycanase) could positively increase the PEPT1, GLUT 2 and SGLT1 mRNA expression in corn, wheat and barley-based diet. According to these, we can know that high fibre replacement in poultry diet will improve not only the gut barrier function but also the nutrient absorption rate. By the enhanced expression of nutrient absorption-related mRNA, animal could have a more efficiency on utilisation of nutrient and further decrease the feed demand when produce the same weight of meat. However, according to the growth performance data shown in Table , we could find out that although the nutrient transporter expression increases significantly, the growth performance only had slightly increase. However, these results also breaking the myth that adding high fibre will reduce animal performance (Buxton and Redfearn Citation1997).
Nassar et al. (Citation2019) reported that the addition of fibre to animal feed would improve body composition. The WHC data suggests that fibre replacement will not affect DL, TL, or CL in breast meat. However, increasing fibre content can increase the shearing force, especially in the PWMC group. Okrathok and Khempaka (Citation2020) reported that a 0.5%, 1%, or 2% supplementation of modified-dietary fibre from cassava pulp will decrease cholesterol content in both breast and thigh meat. This decrease in cholesterol may be the result of increasing shearing force. Shearing force is related to the texture of muscle fibres and it also affects the taste of meat when chewing, which in turn affects consumer preferences (Devatkal et al. Citation2019). As shown in Table , although there was no significant difference (p = .5856 and .3725), high fibre supplementation improved flavour and texture, which slightly increased total acceptance.
The results showed that treatment with high fibre had no significant effect on dressing rate or abdominal fat, but it could reduce the thickness of ScF. This affects the heat dissipation of the animal, thereby resulting in a poor response to heat stress and possibly leading to death. The accumulation of ScF affects the taste of meat and reduces consumer preference (Devatkal et al. Citation2019). The carcase is defined as what’s left of the animal body after removing blood, feathers, organs, the intestinal tract and abdominal fat. The dressing rate is the ratio of the animal’s live weight to its carcase weight. Based on the abdominal fat, ScF and carcase site percentage data, we can infer that releasing the energy stored in ScF will increase the carcase percentage for the inner breast and thigh. Similar results were shown in adipolysis and adipogenesis-related mRNA, as seen in Figure . Adipolysis could increase the amount of the energy source, such as SCFAs, acetyl-CoA or ATP, released from long chain fatty acids (LCFAs) and decrease the accumulation of adipose in adipocytes (Lee et al. Citation2012). AMPK-α2 is one of the main upstream genes which can enhance the activation of CPT-1 and accelerate the transfer of LCFAs into mitochondria and further increase the beta-oxidation of LCFAs (Habets et al. Citation2009). There are many types of fat that could be degraded by specific enzymes. ATGL is one of the lipases which can specifically hydrolyse TG and decrease TG levels in animal serum (Andler et al. Citation2016). ATPCL is a catalyst that can convert the energy in acetyl-CoA and oxaloacetate into ADP and phosphate, and thereby increase the production of ATP and citrate (Nuskova et al. Citation2020). However, when acetyl-CoA levels decrease in animals, beta-oxidation and glucose intake are enhanced to produce more acetyl-CoA and maintain homeostasis (Habets et al. Citation2009). In poultry, KCTD15 can downregulate adipose content in adipocytes (Liang et al. Citation2015). Adiponectin content is positively related to leptin, which can regulate animal feed intake, improve adipolysis and increase muscle growth (Tahmoorespur et al. Citation2010). Furthermore, the adipolysis-related mRNA detected in this report (ATGL, ATPCL and CPT-1) increased significantly, which accelerated adipolysis and increased the production of muscle tissue. Similar results were seen in the serum triglyceride content, which was 54.8, 40.5, 38.2 and 40.2 mg/dL in the control, PWMC, FPW and PP groups, respectively (p = .0008, data not shown). Although rapid adipolysis will decrease adipose concentration in the animal and stimulate a compensatory increase in adipogenesis-related gene expression, such as FAS, CEBP and PPAR-γ, the total amount of fat in the broilers still decreased, especially in the PWMC and PP treatment groups.
In order to maintain survival and growth needs, animals will obtain energy through food intake and the resulting breakdown of carbohydrates, fats and proteins. Fat is a means for animals to store excess energy and can release fatty acids from adipocytes when energy is shortage, carrying them to the organs in need via FABP4 and low-density lipoproteins (Wang et al. Citation2009). In poultry, adipose tissue tends to appear under the skin and abdomen. FAS is an upstream gene which can synthesise fully saturated long chain fatty acids in the liver and adipocytes by a series of condensation reactions by acetyl-CoA and malonyl-CoA (De Silva et al. Citation2019). In addition to the direct synthesis of fatty acids, adipose can also accumulate through the proliferation of adipocytes. CEBP is one of the main regulation genes involved in the late stages of adipocyte differentiation and can enhance adipose accumulation in animal adipocytes with the activation of PPAR-γ (Barbosa-da-Silva et al. Citation2015). PPAR-γ also enhances adipose synthesis, as demonstrated by Barbosa-da-Silva et al. (Citation2015). when mice failed to synthesise fat normally when their PPAR-γ levels were low. PPAR-α, which is in the same family as PPAR-γ, has the opposite effect and can promote beta-oxidation (Barbosa-da-Silva et al. Citation2015), and will lead to metabolic disorders if PPAR-α has an abnormal presentation. When the serum levels of TG or low-density lipoprotein increase, adipocytes will secrete IL-6, produce low-grade inflammation and prompt adipocytes to begin accumulating fat (Aloui et al. Citation2016). However, treatment with PWMC or PP effectively reduced the production of IL-6, lowering the degree of subcutaneous fat accumulation. Although the FPW group had similar results, its IL-6 mRNA expression was not significantly different from the control, and the data in Table shows that its abdominal fat was higher. Therefore, when using FPW as a replacement, the more likely outcome is to transfer subcutaneous fat accumulation to abdominal fat accumulation. This may be due to the increase of FABP4 mRNA. FABP4 is a kind of fatty acid binding protein, which is the main free fatty acid transporter in animal blood. Excessive FABP4 expression can lead to fat infiltration in muscle or liver cells, thereby increasing oxidative stress and causing fat accumulation (Wang et al. Citation2009). FPW seems to upregulate these results and may alter the location of adipose accumulation. In addition, OXT has traditionally been thought to stimulate smooth muscle contraction and regulate animal mood. However, recent studies have shown that OXT can also promote adipolysis and regulate fat distribution (Iwasa et al. Citation2019). Overall, PWMC, FPW and PP can all promote adipolysis and alter the body composition of broilers, however after fermentation, FPW supplementation seems more likely to transfer the site of fat accumulation.
Conclusions
In summary, this report confirms that the inclusion of Mushroom waste compost at 5% in broiler diets can increase nutrient absorption in the animals by stimulating nutrient receptor mRNA expression in intestinal epithelial cells and will not downregulate body weight. Mushroom waste compost at 5% in broiler diets can also activate OXT, adiponectin and adipolysis-related mRNA expression to enhance fat metabolism, slightly increase the percentage of muscle tissue (especially the inner breast and thigh), and reduce the accumulation of subcutaneous fat. Saccharomyces cerevisiae, however, may affect animal body composition through other metabolic patterns. In addition, mushroom waste compost at 5% in broiler diets replacement in the broilers can effectively improve body composition and increase consumer preference for its meat products. Based on these results, indicated that a new use for mushroom waste compost and recommend replacing poultry feed with 5% PWMC.
Ethical approval
This experiment was carried out at National Chung Hsing University (NCHU) in Taiwan and all protocols were as per the Animal Care and Use Committee at NCHU (IACUC: 108-049).
Disclosure statement
The authors certify that there is no conflict of interest with any financial organisation regarding the material discussed in the manuscript.
Additional information
Funding
References
- [AOAC] Association of Agricultural Chemists. 2012. Official methods of analysis of AOAC International. Gaithersburg (MD): AOAC International.
- Aguilar-Toalá JE, Garcia-Varela R, Garcia HS, Mata-Haro V, González-Córdova AF, Vallejo-Cordoba B, Hernández-Mendoz A. 2018. Postbiotics: an evolving term within the functional foods field. Trends Food Sci Tech. 75:105–114.
- Al-Harthi MA, Attia YA, Al-Sagan A, Elgandy MF. 2019a. Nutrients profile, protein quality and energy value of whole prosopis pods meal as a feedstuff for poultry feeding. Ital J Anim Sci. 18:30–38.
- Al-Harthi MA, Attia YA, Elgandy MF. 2019b. The effect of pelleting and enzyme supplementation on performance, carcass and blood parameters of broilers fed on different concentrations of olive cake. Rev Bras Cienc Avic. 19:83–90.
- Al-Harthi MA, Attia YA, El-Shafey AS, Elgandy MF. 2020. Impact of phytase on improving the utilisation of pelleted broiler diets containing olive by-products. Ital J Anim Sci. 19:310–318.
- Aloui F, Charradi K, Hichami A, Subramaniam S, Khan NA, Limam F, Aouani E. 2016. Grape seed and skin extract reduces pancreas lipotoxicity, oxidative stress and inflammation in high fat diet fed rats. Biomed Pharmacother. 84:2020–2028.
- Andler SM, Wang LS, Goddard JM, Rotello VM. 2016. Chapter one – preparation of biocatalytic microparticles by interfacial self-assembly of enzyme–nanoparticle conjugates around a cross-linkable core. Methods Enzymol. 516:1–17.
- Barbosa-da-Silva S, Souza-Mello V, Magliano DC, Marinho TS, Aguila MB, Mandarim-de-Lacerda CA. 2015. Singular effects of PPAR agonists on nonalcoholic fatty liver disease of diet-induced obese mice. Life Sci. 127:73–81.
- Bartoszek A, Moob EV, Biniend A, Fabisiaka A, Krajewsk JB, Mosińska P, Niewinna K, Tarasiuka A, Martemyanov K, Salaga M, et al. 2020. Free fatty acid receptors as new potential therapeutic target in inflammatory bowel diseases. Pharmacol. Res. 152:04604.
- Bjørn-Yoshimoto WE, Underhill SM. 2016. The importance of the excitatory amino acid transporter 3 (EAAT3). Neurochem Int. 98:4–18.
- Bowker B, Zhuang H. 2015. Relationship between water-holding capacity and protein denaturation in broiler breast meat. Poult Sci. 94:1657–1664.
- Braun EJ, Sweazea KL. 2008. Glucose regulation in birds. Comp Biochem Physiol B Biochem Mol Biol. 151:1–9.
- Buxton DR, Redfearn DD. 1997. Plant limitations to fiber digestion and utilization. J Nutr. 127:8145–8185.
- Chuang WY, Lin WC, Hsieh YC, Huang CM, Chang SC, Lee TT. 2019. Evaluation of the combined use of Saccharomyces cerevisiae and Aspergillus oryzae with phytase fermentation products on growth, inflammatory, and intestinal morphology in broilers. Animals. 9:1051.
- De Silva GS, Desai K, Darwech M, Naim U, Jin X, Adak S, Harroun N, Sanchez LA, Semenkovich CF, Zayed MA. 2019. Circulating serum fatty acid synthase is elevated in patients with diabetes and carotid artery stenosis and is LDL-associated. Atherosclerosis. 287:38–45.
- Desai MS, Seekatz AM, Koropatkin NM, Kamada N, Hickey CA, Wolter M, Pudlo NA, Kitamoto S, Terrapon N, Muller A, et al. 2016. A dietary fiber-deprived gut microbiota degrades the colonic mucus barrier and enhances pathogen susceptibility. Cell. 167:1339–1353.
- Devatkal SK, Naveena BM, Kotaiah T. 2019. Quality, composition, and consumer evaluation of meat from slow-growing broilers relative to commercial broilers. Poult Sci. 98:6177–6186.
- El-Ghamry AA, Al-Harthi MA, Attia YA. 2005. Possibility to improve rice polishing utilisation in broiler diets by enzymes or dietary formulation based on digestible amino acids. Eur Poult Sci. 69:49–56.
- Eshel G, Shepon A, Makov T, Milo R. 2014. Land, irrigation water, greenhouse gas, and reactive nitrogen burdens of meat, eggs, and dairy production in the United States. Proc Natl Acad Sci U S A. 111:11996–12001.
- Habets DDJ, Coumans WA, Hasnaoui ME, Zarrinpashneh E, Bertrand L, Viollet B, Kiens B, Jensen TE, Richter EA, Bonen AJ, et al. 2009. Crucial role for LKB1 to AMPKα2 axis in the regulation of CD36-mediated long-chain fatty acid uptake into cardiomyocytes. Biochim Biophys Acta. 1791:212–219.
- Hassan RA, Shafi ME, Attia KM, Assar MH. 2020. Influence of oyster mushroom waste on growth performance, immunity and intestinal morphology compared with antibiotics in broiler chickens. Front Vet Sci. 7:333.
- Iwasa T, Matsuzaki T, Mayila Y, Yanagihara R, Yamamoto Y, Kawakita T, Kuwahara A, Irahara M. 2019. Oxytocin treatment reduced food intake and body fat and ameliorated obesity in ovariectomized female rats. Neuropeptides. 75:49–57.
- Jones MP, Lawrie AC, Huynh TT, Morrison PD, Mautner A, Bismarck A, John S. 2019. Agricultural by-product suitability for the production of chitinous composites and nanofibers utilising Trametes versicolor and Polyporus brumalis mycelial growth. Proc Biochem. 80:95–102.
- Kalantar M, Schreurs NM, Raza SHA, Khan R, Ahmed JZ, Yaghobfar A, Shah MA, Kalantar MH, Hosseini SM, Rahman SU. 2019. Effect of different cereal-based diets supplemented with multi-enzyme blend on growth performance villus structure and gene expression (SGLT1, GLUT2, PepT1 and MUC2) in the small intestine of broiler chickens. Gene Rep. 15:00367.
- Lee JH, Moon MH, Jeong JK, Park YG, Lee YJ, Seol JW, Park SY. 2012. Sulforaphane induced adipolysis via hormone sensitive lipase activation, regulated by AMPK signaling pathway. Biochem Biophys Res Commun. 426:492–497.
- Liang SS, Ouyang HJ, Liu J, Chen B, Nie QH, Zhang XQ. 2015. Expression of variant transcripts of the potassium channel tetramerization domain-containing 15 (KCTD15) gene and their association with fatness traits in chickens. Domest Anim. 50:65–71.
- Lin WC, Lee MT, Lo CT, Chang SC, Lee TT. 2018. Effects of dietary supplementation of Trichoderma pseudokoningii fermented enzyme powder on growth performance, intestinal morphology, microflora and serum antioxidantive status in broiler chickens. Ital J Anim Sci. 17:153–164.
- Madsen SL, Wong EA. 2011. Expression of the chicken peptide transporter 1 and the peroxisome proliferator-activated receptor α following feed restriction and subsequent refeeding. Poult Sci. 90:2295–2300.
- Nassar MK, Lyu S, Zentek J, Brockmann GA. 2019. Dietary fiber content affects growth, body composition, and feed intake and their associations with a major growth locus in growing male chickens of an advanced intercross population. Livest Sci. 227:135–142.
- Nuskova H, Mikesova J, Efimova I, Pecinova A, Pecina P, Drahota Z, Houstek J, Mracek T. 2020. Biochemical thresholds for pathological presentation of ATP synthase deficiencies. Biochem Biophys Res Commun. 521:1036–1041.
- Okrathok S, Khempaka S. 2020. Modified-dietary fiber from cassava pulp reduces abdominal fat and meat cholesterol contents without affecting growth performance of broiler chickens. J Appl Poult Res. 29:229–239.
- Pan YG, Lin WC, Lo CT, Chang SC, Yu B, Lee TT. 2018. Effects of substitution of Bermuda grass hay with Trichoderma fermented rice straw on growth, blood, and rumen fluid parameters in Barbados sheep. J Appl Anim Res. 46:1162–1168.
- Sande D, Oliveira GP, Moura MAF, Martins BA, Lima MT, Takahashi JA. 2019. Edible mushrooms as a ubiquitous source of essential fatty acids. Food Res Int. 125:108524.
- Tahmoorespur M, Ghazanfari S, Nobari K. 2010. Evaluation of adiponectin gene expression in the abdominal adipose tissue of broiler chickens: feed restriction, dietary energy, and protein influences adiponectin messenger ribonucleic acid expression. Poult Sci. 89:2092–2100.
- Teng PY, Chang CL, Huang CM, Chang SC, Lee TT. 2017. Effects of solid-state fermented wheat bran by Bacillus amyloliquefaciens and Saccharomyces cerevisiae on growth performance and intestinal microbiota in broiler chickens. Jpn Poult Sci. 16:552–562.
- Toomer OT, Livingston ML, Wall B, Sanders E, Vu TC, Malheiros RD, Livingston KA, Carvalho LV, Ferket PR. 2019. Meat quality and sensory attributes of meat produced from broiler chickens fed a high oleic peanut diet. Poult Sci. 98:5188–5197.
- Wang Q, Guan T, Li H, Bernlohr DA. 2009. A novel polymorphism in the chicken adipocyte fatty acid-binding protein gene (FABP4) that alters ligand-binding and correlates with fatness. Comp Biochem Physiol B Biochem Mol Biol. 154:298–302.
- Wang CC, Lin LJ, Chao YP, Chiang CJ, Lee MT, Chang SC, Yu B, Lee TT. 2017. Antioxidant molecular targets of wheat bran fermented by white rot fungi and its potential modulation of antioxidative status in broiler chickens. Br Poult Sci. 58:262–271.
- Zou J, Chassaing B, Singh V, Pellizzon M, Ricci M, Fythe MD, Kumar MV, Gewirtz AT. 2018. Fiber-mediated nourishment of gut microbiota protects against diet-induced obesity by restoring IL-22-mediated colonic health. Cell Host Microbe. 23:41–53.