Abstract
Current study explored the acute toxicity and oxidative stress of ammonia-N on Macrobrachium rosenbergii. Prawns were exposed to ammonia-N at 3.01, 3.55, 4.18, 4.92, 5.80, 6.82, and 8.04 mg·L−1 for a 96 h acute toxicity test. In the subsequent sublethal toxicity text, they were exposed to ammonia-N at 0.5 or 1.88 mg·L−1 (1/2 96 h LC50) for 3, 8, 12, 24, 48, and 72 h. The total superoxide dismutase (T-SOD) activity, catalase (CAT) activity and malondialdehyde (MDA) content were detected in the muscle, haemolymph, and gill. The gene expression of antioxidases (Cu/ZnSOD, CAT, GPX), heat shock proteins (HSP60, HSP70, HSP90), and apoptosis-related genes (Caspase 3, Bok) in the muscle and hepatopancreas also was evaluated. The LC50 values of non-ionic ammonia at 24, 48, 72, and 96 h were 7.72, 5.44, 4.28, and 3.78 mg·L−1, respectively. Ammonia-N significantly affected the T-SOD and CAT activities in the haemolymph and gill but not in the muscle. After 72 h of ammonia-N exposure, MDA content in the muscle, haemolymph, and gill in 1.88 mg·L−1 group significantly increased. The relative mRNA expression of antioxidant enzymes, heat shock proteins and Caspase 3 were upregulated in the hepatopancreas and muscle after ammonia exposure, especially in 1.88 mg·L−1. This result suggested that 1/2 96 h LC50 ammonia exposure induced severe oxidative stress, eventually even apoptosis of M. rosenbergii through the caspase pathway.
Half of 96 h LC50 ammonia-N caused severe oxidative stress on M. rosenbergii.
Ammonia-N induced the antioxidase and HSP to protect cells from oxidative stress.
Ammonia-N induced mRNA expression level of Caspase 3 of M. rosenbergii.
Highlights
Introduction
Ammonia-N is one of the most common pollutants in aquatic environment, and it significantly affects growth, gas exchange, acid/base balance, osmoregulation, metabolic profiles, and the immune system of crustaceans (Chen and Kou Citation1992; Romano and Zeng Citation2007; Lu et al. Citation2016). For giant freshwater prawn, the Macrobrachium rosenbergii, ammonia exposure reduced survival rate, growth rate and feed in take (Naqvi et al. Citation2007; Dong et al. Citation2020). Ammonia-N exposure also increased M. rosenbergii susceptibility to pathogens (Cheng and Chen Citation2002; Cheng et al. Citation2003). However, the molecular mechanism of ammonia stress on M. rosenbergii not well understood. Pervious study demonstrated that ammonia exposure could increase production of reactive oxygen species (ROS) and cause oxidative stress, which was one of the toxicity mechanisms of ammonia (Halliwell Citation1999; Cheng et al. Citation2015).
ROS overproduction damaged to the biomacromolecules, including DNA damage and lipid peroxidation, leading to the cell dysfunction. To prevent oxidative damage, cells have developed a complex antioxidant defense system that includes non-enzymatic antioxidants (e.g. glutathione, thioredoxine) as well as enzymatic activities (e.g. Cu–Zn SOD, CAT) (Sies Citation1991). The Cu–Zn SOD transformated the superoxide anion (O2−) into the superoxide anion (H2O2), which is subsequently converted into H2O by CAT and GPx (Yeh et al. Citation2009). Several studies reported that ammonia modulates the activity of antioxidative enzymes in crustaceans (Zhang et al. Citation2015; Li et al. Citation2018; Jiang et al. Citation2019). However, the results obtained sometimes were different. For example, Liang et al. (Citation2016) reported after 96 h a decrease of superoxide dismutase (SOD) activity in the hepatopancreas of Litopenaeus vannamei exposured to 20 mg·L−1 ammonia. In contrast, Jiang et al. (Citation2019) showed that ammonia increased the total antioxidant capacity (T-AOC) and activities of antioxidant enzymes in the tissues such as intestine and hepatopancreas of L. vannamei after 6–24 h of exposure to 15 mg·L−1 ammonia. Thus, the oxidative stress caused by ammonia may be related not only to ammonia tolerance among aquatic organisms but also to the concentration and duration of exposure.
Various environmental stressors have been shown to change the expression of heat shock proteins (HSPs). They are highly conserved and responsible for recognising unfolded or misfolded proteins and help their restructuring (Bernadett and Linda Citation2009). Based on their size, molecular weight and functions in eukaryotes, there can be divided into six major groups of HSPs: small HSPs, HSP60, HSP70, HSP90, HSP100 and HSP110 (Parsell and Lindquist Citation1993). The study of Chaurasia et al. (Citation2016) showed that HSPs such as HSP60, HSP70 and HSP90 can increase the resistance to pathogens and environmental stresses in the giant freshwater prawn (M. rosenbergii). However, previous studies of the ammonia effect on crustaceans have focussed on HSP70 or HSP90, and little is known about the effect on HSP60 (Cheng et al. Citation2015; Li et al. Citation2018; Jiang et al. Citation2019).
HSP60 is an important mitochondrial chaperone which can facilitates the folding of many proteins and help them to be transferred into the mitochondrial matrix. The mitochondrion is the main site of ROS production and the main target of ROS. Qian et al. (Citation2012) compared the expression patterns of HSP60, HSP70, and HSP90 of L. vannamei in response to different acute stresses and reported that HSP60 was most sensitive to pH, cadmium, and manganese, whereas HSP70 was more sensitive to iron and zinc treatments. Hence, studies of the role of different HSPs in the defense mechanism of crustaceans exposed to ammonia could lead to the development of better protection strategies in the aquaculture setting.
The main targets of ROS overproduction in mitochondria are the protein components of the membranes, polyunsaturated fatty acids and mtDNA. Orrenius (Citation2007) suggested that the invading pathogens can stimulate ROS overproduction and so cause the mutations in mtDNA and cell apoptosis. Yuan et al. (Citation2003) previously showed that prolonged oxidative stress can lead to cell death via activation of the apoptotic cell death pathway, and Liang et al. (Citation2016) reported that ammonia stress can induce apoptosis in the hepatopancreas of L. vannamei. Apoptosis can remove old, excess, damaged or potentially dangerous cells. Li et al. (Citation2018) found that ammonia stress caused significant changes to apoptosis-related gene expression in the black tiger shrimp (Penaeus monodon). However, the precise mechanism of ammonia-induced apoptosis in crustaceans remains unclear.
Macrobrachium rosenbergii is a fast-growing and nutrient-rich species, and it has become a commercially important shrimp species in Southern and Southeastern Asian countries (Tidwell et al. Citation2007). It also is considered to be less prone to certain viral diseases in aquaculture compared to penaeid shrimps (Bonami and Widada 2011). Although researchers have studied the ammonia tolerance and ammonia-induced oxidative stress of M. rosenbergii, the effect of ammonia on HSPs and apoptosis have not been investigated previously. In this study, we evaluated the acute toxicity effects of ammonia-N and its effects on antioxidant enzyme activities of total-SOD (T-SOD), CAT, glutathione peroxidase (GSH-Px)) and malondialdehyde (MDA) content in M. rosenbergii. We also quantified the expression levels of antioxidant genes (Cu/ZnSOD, CAT, GPX), HSP genes (HSP60, HSP70, HSP90), and apoptosis-related genes (Caspase3, Bok) in the hepatopancreas and muscle of M. rosenbergii after exposure to ammonia-N.
Materials and methods
Animal preparation
Healthy M. rosenbergii with body weight of 3.46 ± 0.66 g were obtained from a private farm (Yancheng City, Jiangsu Province, China). Prior to the experiment, the prawns were acclimated for 7 d in 80 L laboratory tanks filled with well-oxygenated water (temperature, 27 ± 1 °C; pH 8.0 ± 0.1). During acclimation, the prawns were fed three times each day with a commercial feed at 4% of their body weight, and water was exchanged by 50% daily. All mandatory animal welfare, laboratory health and safety procedures were complied with in the course of conducting any experimental work reported in this paper.
Acute ammonia-N exposure experiments
After acclimation, prawns were randomly selected for the acute ammonia-N exposure experiment. Freshwater was spiked with a stock solution of ammonia-N of 1000 mg L−1, which was prepared by dissolving 38.2 g of NH4Cl into 1 L of distilled water. Water was adjusted to 0 (control), 20, 23.53, 27.73, 32.67, 38.47, 45.27, and 53.33 mg·L−1 total ammonia-N, and the corresponding un-ionised ammonia (NH3–N) concentrations were 0, 3.01, 3.55, 4.18, 4.92, 5.80, 6.82, and 8.04 mg·L−1, respectively. Concentrations of NH3-N were calculated using the un-ionised ammonia calculator provided by Albert (Citation1973).
Each treatment was performed in three parallel tanks, with five individuals per tank. The experiment was conducted in 24 rectangular glass tanks (40 × 20 × 30 cm3). Each tank contained 12 L of well-oxygenated mixed water. Feeding was terminated during the test, and one half of the water was renewed on alternate days to keep ammonia-N concentrations constant. Water was maintained at a temperature of 27 ± 1 °C and a pH of 8.0 ± 0.1, with ≥6.0 mg L−1 dissolved oxygen. The survival of tested prawns in each container was determined at 24, 48, 72, and 96 h. The half lethal concentration (LC50) was estimated using probit analysis.
Test for sublethal toxicity of ammonia-N
The nominal concentrations of ammonia-N were 0.5 and 1.88 mg·L−1, which reflected about 1/10 and 1/2 of the 96 h LC50 based on the results of the acute experiment, and they represent the low level and high level ammonia-N, respectively. Each treatment was performed in three parallel tanks, with 15 individuals per tank. The experiment was conducted in nine rectangular glass tanks (40 × 20 × 30 cm3). Each tank contained 12 L of well-oxygenated mixed water. Feeding was terminated during the test, and one-half of the water was renewed on alternate days to keep ammonia-N concentrations constant. The experimental conditions were the same as those described in Section “Acute ammonia-N exposure experiments”.
Three prawns was randomly removed and anaesthetised on ice from each of the tanks in each treatment group at 3, 8, 12, 24, 48, and 72 h of ammonia-N exposure. A haemolymph sample was obtained from the heart of each prawn using a sterilised syringe with a 25-gauge needle containing 0.3 mL of an anti-coagulant (450 mmol L−1 NaCl, 10 mmol L−1 KCl, 10 mmol L−1 EDTA-Na2, and 10 mmol L−1 HEPES, pH 7.45) with an osmolarity of 780 mOsm kg−1, modified from the anticoagulant devised by Smith and Söderhäll (Citation1983). The haemolymph sample was collected to measure T-SOD and CAT activities and MDA content.
Hepatopancreas and muscle samples were also excised rapidly from each prawn, freeze-dried in liquid nitrogen, and stored at −80 °C for quantitative analysis of antioxidant gene (CAT, Cu/ZnSOD, GPX), HSP gene (HSP60, HSP70, HSP90), and apoptosis-related gene (Caspase3, Bok) expression. Additionally, muscle and gill tissues were sampled to measure MDA content and antioxidant enzyme activities (T-SOD, CAT). GSH-Px activity in muscle tissue also was measured.
Enzyme activity and MDA content assays
The enzyme activities (T-SOD, CAT, and GSH-Px) and the MDA content were measured using commercial assay kits (Jiancheng, Ltd., Nanjing, China). The protein concentrations were determined using a Coomassie Brilliant Blue protein assay kit (Jiancheng, Ltd.). The methods used followed the instructions provided by the manufacturer.
Gene expression assay
Total RNA from hepatopancreas and muscle samples was extracted using TRIzol reagent (TaKaRa, Dalian, China) according to the manufacturer's instructions. The RNA sample was reverse transcribed using a PrimeScript® RT reagent kit with DNA Eraser (TaKaRa). The quantitative polymerase chain reaction (qPCR) was performed in triplicate using the Bio-Rad CFX96 Real-Time PCR Detection System (Bio-Rad, Hercules, CA, USA). The specific primers of antioxidant genes (Cu/ZnSOD, CAT, GPX), HSP genes (HSP60, HSP70, HSP90), and apoptosis-related genes (Caspase3, Bok) were used in this study (Table ). The relative mRNA levels in all tissues were calculated using the 2−ΔΔCT method (Livak and Schmittgen Citation2001). The gene mRNA levels were normalised with β-actin as reference gene.
Table 1. Fluorescence quantitative PCR reaction primer.
Statistical analysis
The gene expression, antioxidant enzyme activity, and MDA content results were tested with one-way analysis of variance followed by least significant difference multiple comparisons using SPSS 16.0 software (SPSS Inc., Chicago, IL, USA). Results were considered to be significantly different at p<.05.
Results
Ammonia-N acute experiment
The LC50 values of ammonia-N were 7.72, 5.44, 4.28, and 3.78 mg·L−1 at 24, 48, 72, and 96 h, respectively, for M. rosenbergii, and the safe concentration was 0.378 mg·L−1 (Table ).
Table 2. The LC50 and Sc of ammonia-N stress on M. rosenbergii.
Effect of ammonia-N exposure on T-SOD and CAT activities of M. rosenbergii
Figure shows the T-SOD and CAT activities of prawns at different levels of ambient ammonia–N exposure. Ammonia-N significantly affected the T-SOD and CAT activities of the haemolymph and gill of M. rosenbergii (p<.05), but this effect was not observed in muscle (p>.05). At the high level of ammonia-N (1.88 mg·L−1), increased T-SOD activity of haemolymph occurred after 24 h of exposure compared with that of the 0.5 mg·L−1 and control groups (p<.05). However, activity decreased after 48 h of exposure and was significantly lower than that of the control (p<.05). The high dose also significantly increased T-SOD of the gill after 8 h of exposure compared with that of the low dose and control groups (p<.05).
Figure 1. Effect of ammonia-N on the T-SOD and CAT activities of haemolymph, muscle and gill of M. rosenbergii. Notes: The different lowercase mean significant difference at same time (p<.05), no lowercase mean no significant differences at same time (p>.05). T-SOD: Total Superoxide Dismutase; CAT: Catalase.
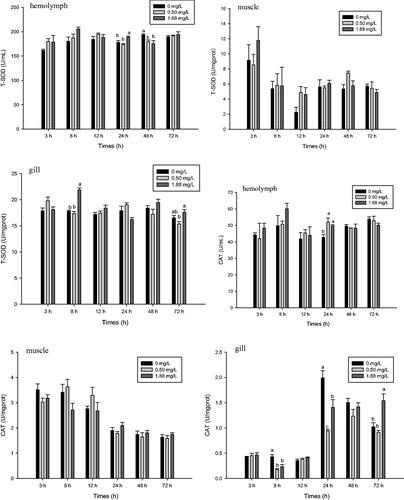
After 24 h of exposure to 0.5 and 1.88 mg·L−1 ammonia-N, CAT activity significantly increased in the haemolymph (p<.05). The high dose also increased the CAT activity of the gill after 72 h of exposure (p<.05). However, compared with the control, CAT activity of the gill was lower at 8 and 24 h post-exposure at the high and low doses, respectively (p<.05) (Figure ). After 72 h of exposure, 0.5 and 1.88 mg·L−1 ammonia-N significantly increased GSH-Px activity of muscle compared with the control (Figure ).
Effect of ammonia-N exposure on MDA content of M. rosenbergii
MDA content of M. rosenbergii was significantly affected by exposure to ammonia-N (p<.05) (Figure ). MDA content of the 0.5 mg·L−1 group peaked in the haemolymph at 3 h and in the gill at 8 h, with increases of 95.27 and 111.33%, respectively, compared with the control (p<.05). Afterwards the content significantly decreased and recovered to the control level by 72 h. After 12 h of exposure to the high dose, MDA content increased in the haemolymph but decreased in the muscle. MDA content decreased in the gill after 3 and 48 h of exposure to 1.88 mg·L−1 ammonia-N. Compared with the control, exposure to the high dose significantly increased MDA content in the haemolymph, muscle, and gill of M. rosenbergii after 72 h.
Effect of ammonia-N exposure on expression of antioxidant genes in M. rosenbergii
The relative mRNA expression levels of Cu/ZnSOD, CAT, and GPX in the hepatopancreas and muscle were affected by ammonia-N exposure (Figure ). After 8 h of exposure, expression Cu/ZnSOD in the hepatopancreas and muscle peaked in the 1.88 mg·L−1 group (Figure ), with values 6.53 and 7.98 times that of the control (p<.05), respectively. Compared with the 0.50 mg·L−1 and control groups, the 1.88 mg·L−1 dose also significantly increased the expression of Cu/ZnSOD in the hepatopancreas at 48 h and muscle at 24 h (p<.05). After 72 h of exposure, expression of Cu/ZnSOD in both treatment groups was not significantly different from that of the control.
Figure 4. Effect of ammonia-N on the relative expression Cu/ZnSOD, CAT, and GPX of muscle and hepatopancreas in M. rosenbergii. Notes: The different lowercase mean significant difference at same time (p<.05), no lowercase mean no significant differences at same time (p>.05). Cu/ZnSOD: Copper and zinc-containing superoxide dismutase; CAT: Catalase; GPX: Glutathione peroxidase.
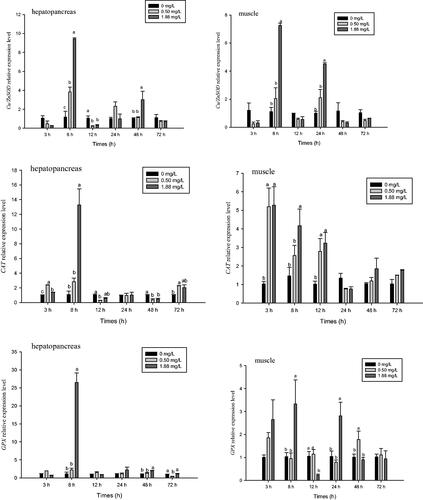
Compared with the control, ammonia-N doses of 0.5 and 1.88 mg·L−1 significantly increased the relative mRNA expression level of CAT in muscle at 3 and 12 h and hepatopancreas at 3 and 8 h, respectively (p<.05) (Figure ). Thereafter, expression of CAT in both hepatopancreas and muscle decreased significantly. After 48 h of ammonia-N exposure, expression of CAT in the hepatopancreas in the 0.5 mg·L−1 group was lower than that of the control group. However, it increased after 72 h of exposure and was significantly higher than that of the control. Expression of CAT in muscle did not differ significantly from that of the control at 24, 48, or 72 h (p>.05).
After 8 h of ammonia-N exposure, the relative mRNA expression levels of GPX in the hepatopancreas and muscle in the high dose group peaked, with values that were 23.86 times that of the control and 12.21 times that of the low dose group (p<.05) (Figure ). Thereafter, they significantly decreased, and expression of GPX in muscle in the high dose group was significantly decreased than those of the low dose and control groups at 12 h (p<.05). Expression levels of GPX at 1.88 mg·L−1 (muscle at 24 h, hepatopancreas at 48 h) and 0.5 mg·L−1 (muscle at 48 h) were higher than that of the control (p<.05). After 72 h of exposure, expression of GPX in muscle at both doses did not differ significantly compared with the control, but expression of GPX in the hepatopancreas in the 0.5 mg·L−1 group was significantly lower than that of the 1.88 mg·L−1 and control groups.
Effect of ammonia-N exposure on expression of HSP genes in M. rosenbergii
The relative mRNA expression levels of HSP60, HSP70, and HSP90 in the hepatopancreas and muscle were affected by ammonia–N (Figure ). After 3 h of ammonia-N exposure, expression of HSP60 in muscle in both treatment groups significantly increased (p<.05). However, no significant difference in HSP60 expression was observed in the 0.5 mg·L−1 group at any other time point. Compared with the control, the high dose significantly increased expression of HSP60 in the muscle at 8, 24, and 72 h and the hepatopancreas at 8, 24, 48, and 72 h (p<.05).
Figure 5. Effect of ammonia-N on the relative expression of HSP60, HSP70, and HSP90 of muscle and hepatopancreas in M. rosenbergii. Notes: The different lowercase mean significant difference at same time (p<.05), no lowercase mean no significant differences at same time (p>.05).
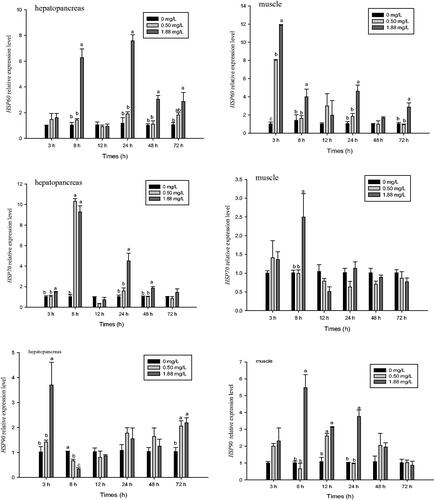
After 8 h of ammonia-N exposure, the expression level of HSP70 in the hepatopancreas and muscle peaked in the high dose group, with values that were 9.11 and 2.47 times that of the control, respectively (p<.05) (Figure ). Compared with the control and low dose group, the high dose group also showed increased expression of HSP70 in the hepatopancreas at 3, 24, and 48 h (p<.05). However, no significant difference in expression of HSP70 in muscle was observed between treatment and control groups at any other time point. The expression level of HSP70 did not differ between the 0.5 mg·L−1 and control groups, except for in the hepatopancreas at 8 h, where it was significantly higher than that of the control (p<.05).
Compared with the control and 0.5 mg·L−1 groups, 1.88 mg·L−1 ammonia-N exposure significantly increased the expression level of HSP90 in the hepatopancreas after 3 h (p<.05) (Figure ), but then it significantly decreased at 8 h and did not differ significantly from the control at 12, 24, and 48 h. However, after 72 h of exposure, both treatment groups exhibited significantly elevated expression of HSP90 in the hepatopancreas relative to the control (p<.05). The relative mRNA expression level of HSP90 in the muscle peaked at 8 h in the high dose group and at 12 h in the low dose group. Thereafter, the expression levels significantly decreased. Expression of HSP90 in the muscle in the 1.88 mg·L−1 group was still significantly higher than that of the control at 12 and 24 h (p<.05). Compared with the control, expression of HSP90 in the muscle of both treatment groups did not differ significantly after 48 and 72 h of ammonia-N exposure.
Effect of ammonia-N exposure on expression of apoptosis-related genes in M. rosenbergii
The relative mRNA expression levels of Caspase 3 in the hepatopancreas and muscle were affected by ammonia–N (p<.05) (Figure ). Compared with the control, the 1.88 mg·L−1 dose of ammonia-N significantly increased expression of Caspase 3 in the hepatopancreas at 8 and 24 h (p<.05) (Figure ). Expression of Caspase 3 in the high dose group at 24 h was also higher than that of the low dose group (p<.05). No significant difference in the expression of Caspase 3 in the hepatopancreas was observed between the ammonia groups and the control at any other time point (p>.05). After 24 and 48 h of exposure, 1.88 mg·L−1 of ammonia-N increased the expression of Caspase 3 in muscle compared with that of the control and 0.5 mg·L−1 groups.
Figure 6. Effect of ammonia-N on the relative expression of Caspase3 and Bok of muscle and hepatopancreas in M. rosenbergii. Notes: The different lowercase mean significant difference at same time (p<.05), no lowercase mean no significant differences at same time (p>.05).
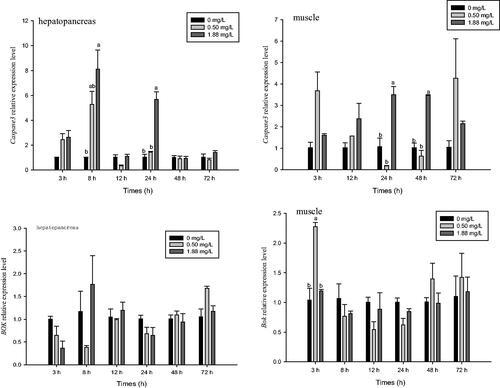
The relative mRNA expression level of Bok in muscle was affected by ammonia-N (p<.05), but its expression in the hepatopancreas was not (p>.05) (Figure ). After 3 h of exposure, expression of Bok in the muscle was significantly increased in the low dose group compared with that of the control and high dose groups, but no significant difference between the ammonia and control groups was detected at any other time point.
Discussion
The antioxidant capacity of M. rosenbergii exposed to ammonia-N
Ammonia has been reported to increase the concentration of ROS, causing oxidative stress in aquatic animals (Cheng et al. Citation2015; Zhang et al. Citation2015; Liang et al. Citation2016). Antioxidant enzymes such as SOD, CAT, and GPx are responsible for eliminating excess ROS. MDA content is most frequently used to monitor lipid peroxidation (Lushchak Citation2011). The effect of ammonia on antioxidant enzymes has been demonstrated previously in muscle, gill, haemolymph, and hepatopancreas of crustaceans (Zhang et al. Citation2015; Liang et al. Citation2016; Jiang et al. Citation2019). In this study, variation of antioxidant enzyme activity in different tissues of M. rosenbergii was found after ammonia exposure. The T-SOD activity of the gill peaked at 8 h after exposure to the high level of ammonia (1.88 mg·L−1), and it significantly increased in the haemolymph at 24 h and then decreased. However, the SOD and CAT activities of muscle were not affected significantly by ammonia. These results suggested that the T-SOD of the gill of M. rosenbergii was most sensitive to ammonia-N, followed by the haemolymph and then the muscle. The gill of crustaceans is not only responsible for gas exchange and osmoregulation, but it also is the main excretor of ammonia. NH3 in water can directly enter gill epithelial cells, affecting the excretion of ammonia-N from the body and causing damage to gill tissue (Romano and Zeng Citation2007). This likely explained the sensitivity of the gill to ammonia-N observed in this study.
The transcript level of Mn-SOD and CAT in the liver of the pufferfish (Takifugu obscurus) increased significantly after ammonia-N exposure (Cheng et al. Citation2015). Similar results were found in this study. The expression level of Cu/ZnSOD, CAT, and GPX in the hepatopancreas and muscle were affected significantly by exposure to ammonia-N, and they peaked at 8 h in the high dose group, except for CAT in the muscle. Expression changes of these genes in the hepatopancreas were greater than those in the muscle.
Liang et al. (Citation2016) found that the MDA content in the hepatopancreas of L. vannamei increased significantly after 96 h of ammonia exposure, and Jiang et al. (Citation2019) reported that MDA content of the hepatopancreas increased throughout their ammonia exposure experiment. In contrast, dynamic changes of MDA content were found in M. rosenbergii in the current study. However, MDA concentrations in the gill, muscle, and haemolymph of M. rosenbergii significantly increased at the end of the experiment in the group exposed to the high dose of ammonia-N, which suggests that ROS were overproduced, thereby inducing severe membranous lipid peroxidation.
HSP and apoptosis-related gene expression in M. rosenbergii in response to ammonia-N
In response to cellular stressors, such as hyperthermia, oxidative damage, physical injury, or chemicals, the expression of HSPs increases dramatically (Bernadett and Linda Citation2009). HSP70 is related to the modulation of cellular redox status and reduction of ROS level and to enhancing cell survival functions (Azad et al. Citation2011). Transcriptome analysis of the hepatopancreas in L. vannamei showed that ammonia upregulated the expression of HSP70 but downregulated the expression of HSP 90 (Lu et al. Citation2016). Similar results were reported for P. monodon (Li et al. Citation2018). Ammonia stress changed the HSP70 and HSP90 transcription of T. obscurus, and the HSP90 mRNA level was upregulated in a concentration-dependent manner (Cheng et al. Citation2015).
Results of the present study also showed that expression levels of HSP60, HSP70, and HSP90 in the muscle or hepatopancreas of M. rosenbergii increased in the high level ammonia-N group, but different expression profiles were obtained in hepatopancreas and muscle tissues. The maximal expression levels of HSP90, HSP70, and HSP60 were observed in the hepatopancreas at 3, 8, and 24 h after ammonia exposure (1.88 mg L−1), with values of 3.63, 6.52, and 9.11 times that of control, respectively. Moreover, low concentration of ammonia induced the expression of HSP70 and HSP90 at 8 and 72 h, respectively, but it had no effect on the expression of HSP60. These results indicated that the transcription level of HSP70 and HSP90 was more sensitive than that of HSP60 in the hepatopancreas. However, among the three HSPs, HSP60 played the dominant role in protecting cells against ammonia stress in muscle tissue. For example, the maximal expression of HSP60 in muscle in the 1.88 mg L−1 ammonia treatment group occurred at 3 h and was 12 times that of the control. In contrast, the maximal expression of HSP70 and HSP90 in the muscle in the high ammonia group was observed at 8 h, with values that were 2.47 and 5.40 times that of the control, respectively. Chaurasia et al. (Citation2016) previously reported that the expression levels of HSP60, HSP70, and HSP90 of M. rosenbergii were highly expressed in haemocytes, gill, and hepatopancreas, respectively, and they showed that HSP60 of M. rosenbergii had the most dominant role in protecting cells against infectious pathogens. Results of the present study showed that ammonia exposure changed HSP60, HSP70, and HSP90 transcription, which suggested that HSPs were beneficial for protein repair, protein synthesis and degradation, and maintenance of the normal function cell after ammonia-N exposure in M. rosenbergii. Moreover, the sensitivity of HSPs differed among different tissues.
The defense mechanism of an organism is usually activated under environmental stress or pathogen infection, and cell death or apoptosis is one form of defense (Cohen Citation1993). Overproduction of ROS induces opening of the mitochondrial permeability transition pore, leading to cell death via apoptosis (Ryter et al. Citation2007, Schieber and Chandel Citation2014). Ammonia exposure increased the apoptotic cell ratio in blood cells of T. obscurus (Cheng et al. Citation2015) and in the hepatopancreas of L. vannamei (Liang et al. Citation2016). In mammals, the cell apoptosis pathway includes the extrinsic pathway and the intrinsic or mitochondrial pathway, and both require caspase activation. Translocation of apoptotic factor cytochrome c from mitochondria to the cytosol is a crucial step in activation of the apoptosis machinery in the mitochondrial pathway. Once released, cytochrome c can bind apoptosis protease-activating factor 1 to form the apoptosome and activate caspase-9. Once active, caspase-9 can directly cleave and activate caspase-3, which is the primary executioner of apoptotic death (Li et al. Citation1997). At present, only a few studies have focussed on the apoptotic mechanism of crustaceans under ammonia-N stress. Liang et al. (Citation2016) suggested that ammonia-N can regulate hepatopancreas cell apoptosis of L. vannamei by initiating the unfolded protein response pathway of the endoplasmic reticulum. In the present study, the relative mRNA expression levels of Caspase 3 in the hepatopancreas and muscle were affected by the high dose ammonia-N treatment but not by the low dose treatment.This result suggested that high level ammonia may induce oxidative stress and eventually even apoptosis in the hepatopancreas and muscle cells of M. rosenbergii through the caspase pathway.
Cell lymphoma 2 (BCL-2) ovarian killer (Bok) is a BCL-2 family protein with high homology to the multidomain proapoptotic BAX and BAK proteins, but little was known about its roles in the process of apoptosis (Zhang et al. Citation2019). Bok was initially isolated and cloned from a rat ovary (Hsu et al. Citation1997). Similarly, Bok mRNA is expressed most abundantly (p<.05) in the ovary of M. rosenbergii, and the gene expression pattern after bacterial and viral induction showed that Bok is a proapoptotic protein that promotes cell death by interacting with selective anti-apoptotic proteins (Chaurasia et al. Citation2015). Zhang et al. (Citation2019) showed that overexpression of Bok promoted the repair of oxidative damage by hydrogen peroxide. In the current study, 0.5 mg·L−1 ammonia-N significantly increased expression of Bok in the muscle compared with that of the control and 1.88 mg·L−1 groups, which may be related to the repair of oxidative damage caused by ammonia. However, the precise apoptosis mechanism of action of Bok in M. rosenbergii requires further study.
Conclusions
In summary, we demonstrated the effects of ammonia-N exposure on antioxidant enzymes, heat shock proteins, and apoptosis-related genes of M. rosenbergii. Our results indicated that ammonia exposure could induce antioxidant activity in the haemolymph and gill. Compared with haemolymph and muscle, the gill of M. rosenbergii had the highest sensitivity to ammonia-N. The 1/2 of 96-LC50 (1.88 mg·L−1) of ammonia-N caused significant lipid peroxidation. Relative mRNA expression of antioxidant enzymes and heat shock proteins was induced in the hepatopancreas and muscle after ammonia exposure, especially at 1.88 mg·L−1 group, indicating that the antioxidant system and heat shock proteins tried to protect cells from oxidative stress. Moreover, expression of the apoptosis genes Caspase 3 also was induced. Overall, these results suggested that half of the 96 h LC50 ammonia exposure induced severe oxidative stress, eventually even apoptosis of M. rosenbergii through the caspase pathway.
Disclosure statement
No potential conflict of interest was reported by the author(s).
Additional information
Funding
References
- Albert A. 1973. Selective toxicity. 5th ed. London, England: Chapman and Hall.
- Azad P, Ryu J, Haddad GG. 2011. Distinct role of Hsp70 in Drosophila hemocytes during severe hypoxia. Free Radic Biol Med. 51(2):530–538.
- Bernadett K, Linda G. 2009. Induction of heat shock proteins for protection against oxidative stress. Adv Drug Deliver Rev. 61(4):310–318.
- Bonami JR, Widada JS. 2011. Viral diseases of the giant fresh water prawn Macrobrachium rosenbergii: a review. J Invertebr Pathol. 106(1):131–142.
- Chaurasia MK, Nizam F, Ravichandran G, Arasu MV, Al-Dhabi NA, Arshad A, Elumalai P, Arockiaraj J. 2016. Molecular importance of prawn large heat shock proteins 60, 70 and 90. Fish Shellfish Immun. 48:228–238.
- Chaurasia MK, Palanisamy R, Harikrishnan R, Arasu MV, Al-Dhabi NA, Arockiaraj J. 2015. Molecular profiles and pathogen-induced transcriptional responses of prawn B cell lymphoma-2 related ovarian killer protein (BOK). Fish Shellfish Immun. 45(2):598–607.
- Chen JC, Kou YZ. 1992. Effects of ammonia on growth and molting on Penaeus japonicus juveniles. Aquaculture. 104(3–4):249–260.
- Cheng CH, Yang FF, Ling RZ, Liao SA, Miao YT, Ye CX, Wang AL. 2015. Effects of ammonia exposure on apoptosis, oxidative stress and immune response in pufferfish (Takifugu obscurus). Aquat Toxicol. 164(1):61–71.
- Cheng W, Chen JC. 2002. The virulence of Enterococcus to freshwater prawn Macrobrachium rosenbergii and its immune resistance under ammonia stress. Fish Shellfish Immunol. 12(2):97–109.
- Cheng W, Chen SM, Wang FI, Hsu PI, Liu CH, Chen JC. 2003. Effects of temperature, pH, salinity and ammonia on the phagocytic activity and clearance efficiency of giant freshwater prawn Macrobrachium rosenbergii to Lactococcus garvieae. Aquaculture. 219(1–4):111–121.
- Cohen JJ. 1993. Apoptosis: The physiologic pathway of cell death. Hosp Pract. 28(12):35–43.
- Dong XX, Liu QG, Kan DQ, Zhao WH, Guo HS, Lv LL. 2020. Effects of ammonia-N exposure on the growth, metabolizing enzymes, and metabolome of Macrobrachium rosenbergii. Ecotoxicol Environ Saf. 189:110046.
- Halliwell B. 1999. Antioxidant defence mechanisms: from the beginning to the end (of the beginning). Free Radic Res. 31(4):261–272.
- Hsu SY, Kaipia A, McGee EM, Lomeli M, Hsueh AJW. 1997. Bok is a pro-apoptotic Bcl-2 protein with restricted expression in reproductive tissues and heterodimerizes with selective anti-apoptotic Bcl-2 family members . Proc Natl Acad Sci USA. 94(23):12401–12406.
- Jiang L, Feng JX, Ying R, Yin FM, Pei SR, Lu JG, Cao YT, Guo JL, Li ZF. 2019. Individual and combined effects of ammonia-N and sulfide on the immune function and intestinal microbiota of Pacific white shrimp Litopenaeus vannamei. Fish Shellfish Immunol. 92:230–240.
- Li P, Nijhawan D, Budihardjo I, Srinivasul SM, Ahmad M, Alnemri ES, Wang XD. 1997. Cytochrome c and dATP-dependent formation of Apaf-1/caspase-9 complex initiates an apoptotic protease cascade. Cell. 91(4):479–489.
- Li YD, Zhou FL, Huang JH, Yang LS, Jiang S, Yang QB, He JG, Jiang SG. 2018. Transcriptome reveals involvement of immune defense, oxidative imbalance, and apoptosis in ammonia-stress response of the black tiger shrimp (Penaeus monodon). Fish Shellfish Immunol. 83:162–170.
- Liang ZX, Liu R, Zhao DP, Wang LL, Sun MZ, Wang MQ, Song LS. 2016. Ammonia exposure induces oxidative stress, endoplasmic reticulum stress and apoptosis in hepatopancreas of pacific white shrimp (Litopenaeus vannamei). Fish Shellfish Immun. 54(1):523–528.
- Livak KJ, Schmittgen TD. 2001. Analysis of relative gene expression data using real-time quantitative PCR and the 2(-delta delta C(T)) method. Methods. 25(4):402–402e408.
- Lu X, Kong J, Luan S, Dai P, Meng XH, Cao BX, Luo K. 2016. Transcriptome analysis of the hepatopancreas in the Pacific White Shrimp (Litopenaeus vannamei) under acute ammonia stress. PLOS One. 11(10):e0164396.
- Lushchak VI. 2011. Environmentally induced oxidative stress in aquatic animals. Aquat Toxicol. 101(1):13–30.
- Naqvi AA, Adhikari S, Pillai BR, Sarangi N. 2007. Effect of ammonia‐N on growth and feeding of juvenile Macrobrachium rosenbergii. Aquaculture Res. 38(8):847–851.
- Orrenius S, Gogvadze V, Zhivotovsky B. 2007. Mitochondrial oxidative stress: implications for cell death. Annu Rev Pharmacol Toxicol. 47:143–183.
- Parsell DA, Lindquist S. 1993. The function of heat-shock proteins in stress tolerance: degradation and reactivation of damaged proteins. Annu Rev Genet. 27:437–e496.
- Qian ZY, Liu XL, Wang LJ, Wang XZ, Li Y, Xiang JH, Wang P. 2012. Gene expression profiles of four heat shock proteins in response to different acute stresses in shrimp, litopenaeus vannamei. Comp Biochem Physiol C Toxicol Pharmacol. 156(3–4):211–220.
- Romano N, Zeng CS. 2007. Ontogenetic changes in tolerance to acute ammonia exposure and associated gill histological alterations during early juvenile development of the blue swimmer crab, Portunus pelagicus. Aquaculture. 266(1–4):246–254.
- Ryter SW, Kim HP, Hoetzel A, Park JW, Nakahira K, Wang X, Choi AMK. 2007. Mechanisms of cell death in oxidative stress. Antioxid Redox Signal. 9(1):49–89.
- Schieber M, Chandel NS. 2014. ROS Function in redox signaling and oxidative stress. Curr Biol. 24(10):R453–R462.
- Sies H. 1991. Oxidative stress: From basic research to clinical application. Am J Med. 91(3C):31S–38S.
- Smith VJ, Söderhäll K. 1983. Induction of degranulation and lysis of haemocytes in the freshwater crayfish, Astacus astacus by components of the prophenoloxidase activating system in vitro. Cell Tissue Res. 233(2):295–303.
- Tidwell JH, Coyle S, Arnum A, Weibel C. 2007. Production response of freshwater prawns Macrobrachium rosenbergii to increasing amounts of artificial substrate in ponds. J World Aquacult Soc. 31(3):452–458.
- Yeh SP, Liu KF, Chiu ST, Jian SJ, Cheng W, Liu CH. 2009. Identification and cloning of a selenium dependent glutathione peroxidase from giant freshwater prawn, Macrobrachium rosenbergii. Fish Shellfish Immunol. 27(2):181–191.
- Yuan J, Murrell GAC, Trickett A, Wang MX. 2003. Involvement of cytochrome c release and caspase-3 activation in the oxidative stress-induced apoptosis in human tendon fibroblasts. BBA-Mol Cell Res. 1641(1):35–41.
- Zhang F, Ren L, Zhou SS, Duan P, Xue JC, Chen HQ, Feng YF, Yue XX, Yuan PF, Liu QZ, et al. 2019. Role of B-cell lymphoma 2 ovarian killer (BOK) in acute toxicity of human lung epithelial cells caused by cadmium chloride. Med Sci Monit. 25:5356–5368.
- Zhang YF, Ye CX, Wang AL, Zhu X, Chen CH, Xian JN, Sun ZZ. 2015. Isolated and combined exposure to ammonia and nitrite in giant freshwater pawn (Macrobrachium rosenbergii): effects on the oxidative stress, antioxidant enzymatic activities and apoptosis in haemocytes. Ecotoxicology. 24(7–8):1601–1610.