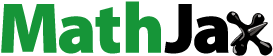
Abstract
The effects of Tenebrio molitor larvae meal (TM) dietary inclusion in rainbow trout diet were evaluated on muscular growth using gene expression and histomorphological features of liver, spleen, kidney, anterior and posterior gut through histopathological analyses. Two hundred fifty-two grow-out rainbow trout were fed four experimental diets containing increasing levels of TM: 0% (TM0), 5% (TM25), 10% (TM50), and 20% (TM100) corresponding to different levels of fish meal replacement (0, 25, 50, and 100%, respectively). Muscular growth was evaluated analysing the expression of various genes involved in different steps of myogenesis. Among the analysed genes, only MyoD expression resulted significantly higher in fish fed TM100 compared to fish fed TM0. The gut histomorphology was not affected by TM dietary inclusion and villus height differs from anterior and posterior segments regardless of the fed diet. Histopathological alterations were observed in all the sampled organs for all the dietary treatments; however, dietary TM inclusion did not influence either development or severity of the observed histopathological changes. The results obtained confirmed the safe utilisation of TM as an alternative protein source in rainbow trout diets and highlighted the necessity to deepen the studies of TM effect on the myogenesis process, especially at a molecular level.
Rainbow trout can effectively be fed with a TM protein source.
Total FM substitution by TM (TM100) decreased MyoD gene expression.
Increasing TM dietary inclusion did not influence gut histomorphology.
Highlights
Introduction
In recent years, a growing interest in insect meal as an alternative protein source in aquafeed has promoted investigations and related scientific production. The implications on growth, nutrient digestibility, fillet quality, and sensorial perception (Borgogno et al. Citation2017; Gasco et al. Citation2018; Lock et al. Citation2018; Ferrer Llagostera et al. Citation2019; Chemello et al. Citation2020; Caimi et al. Citation2020b), as well as the effects on gut health, immune system, and microbiota, have all been analysed in different marine and freshwater species (Elia et al. Citation2018; Henry et al. Citation2018a, Citation2018b; Antonopoulou et al. Citation2019; Rimoldi et al. Citation2019; Roques et al. Citation2020; Caimi et al. Citation2020a). The assessment of animal health status represents a crucial aspect when a novel feed ingredient is tested for its introduction in commercial fish farming. Moreover, optimal growth performance is one of the features that reflects fish health and still represents a crucial aspect for the farmers.
Fish growth consists of muscle requirements. In most teleosts, the axial skeletal muscles represent more than 60% of body mass. Up to 90% of these muscles mainly consist of deep fast white fibres covered by a thin superficial layer of slow red muscle fibres are located under the skin, with an intermediate layer of pink fibres between them (Alami-Durante et al. Citation2010a). Changes in the somatic growth of fish are thus mainly attributable to changes in total white muscle growth, which is the result of muscle hypertrophy (i.e. increase in the size of existing muscle fibres) and/or hyperplasia (i.e. recruitment of new muscle fibres). In rainbow trout (Oncorhynchus mykiss), as well as in other teleost species reaching a large adult body size, the myogenesis of white skeletal muscle occurs by both hyperplasia and hypertrophy not only before hatching but also over a large extent of post-hatching life (Rowlerson and Veggetti Citation2001).
The different steps of myogenesis, such as the commitment of muscle precursors, myoblast proliferation, migration, alignment, and fusion into myotubes – are controlled by the expression of different regulatory factors, including myogenic regulatory factors (MRFs) and growth factors. Therefore, identifying and studying these factors is of utmost importance to understand muscle development and, in turn, fish growth.
MRFs are selectively expressed during the determination, differentiation, activation, and proliferation of satellite cells (also referred to as myogenic precursor cells, MPCs) and in cells entering the terminal differentiation program. Primary myogenic regulatory factors, such as MyoD and Myf-5, play key roles in the specification of muscle lineage; while the secondary myogenic regulatory factors, myogenin (Myog) and Myf-6, control the differentiation of myoblasts into skeletal muscle fibres. Proliferative muscle precursor cells also express PCNA (proliferating cell nuclear antigen), which acts as a cofactor of DNA polymerase-d and is necessary for cell-cycle progression and cell proliferation (Sabourin et al. Citation1999; Sabourin and Rudnicki Citation2000; Buckingham and Rigby Citation2014).
The complex mechanism of myogenesis varies between families and strains and it is influenced by different environmental factors, including the diet (Johnston Citation1999). To date, the effects of nutrients on white muscle development have been studied mainly in fish larvae and juveniles (Bjornevik et al. Citation2003; Ostaszewska et al. Citation2008; Silva et al. Citation2009; Alami-Durante et al. Citation2014; Vo et al. Citation2016; Canada et al. Citation2018; Alami-Durante et al. Citation2019). In particular, changes in dietary macronutrient source and composition have been reported to alter the mRNA level of myosin components in rainbow trout alevins and juveniles (Alami-Durante et al. Citation2014), in Atlantic salmon (Hevrøy et al. Citation2006), and Senegalese sole juveniles (Valente et al. Citation2016). In rainbow trout juveniles, the reduction of growth performances in animals fed a high level of plant protein sources has been linked to changes in the dynamics of white muscle growth and correlated with the differential expression of the Cathepsin D, a gene involved in lysosomal proteolysis in the white muscle (Alami-Durante et al. Citation2010a). Furthermore, white muscle cellularity (distribution of skeletal white muscle fibre size and diameter) has been influenced by changes in dietary protein source and amino acid supplementation in fish juveniles (Alami-Durante et al. Citation2010b; Valente et al. Citation2016), as well as by changes in the dietary protein level (Alami-Durante et al. Citation2019). Unfortunately, the understanding of myogenesis regulation by specific nutrients in grown-out fish and the effects on the related genes is poorly documented or even absent, especially if the use of insect meal as an alternative protein source is considered. To our knowledge, no information on the effects of insect dietary inclusion on the expression of myogenesis regulatory genes in fish is currently available.
Since the digestion of the administered nutrients represents the first step of protein absorption and utilisation, the intestine plays a key role when novel protein sources are included in fish diets. Indeed, gut health status is the main determinant of fish health and performance (Caimi et al. Citation2020a, Citation2020b). Therefore, the parallel evaluation of selected mucosal morphometric features (such as the villus height) and inflammatory cell infiltration – along with the classical histological examination of the main organs – has nowadays become a routine approach. Previous studies have already assessed the effects of dietary Hermetia illucens meal inclusion on gut morphology and histological features of the main organs in rainbow trout (Renna et al. Citation2017; Elia et al. Citation2018), but data about the modulation of such aspects by TM utilisation in this fish species are still lacking.
Based on the above-reported background, the present study described, for the first time, the effects of total fish meal (FM) replacement by TM on MRFs gene expression in muscle tissue and both partial and total FM substitution by TM on histomorphological features in liver, spleen, kidney, anterior and posterior gut of rainbow trout. Our research aims to provide preliminary information on the process that coordinates muscle growth at the molecular level and the structural tissue integrity of fish fed with different dietary TM levels.
Materials and methods
All the samples analysed in this study were collected and processed at the end of a growth trial carried out at the Experimental Facility of the Department of Agricultural, Forest, and Food Sciences (DISAFA) of the University of Turin (Italy). The experimental protocol was approved by the Ethical Committee of the University of Turin (protocol n° 143811).
The experimental protocol, as well as diet preparation, ingredients, and proximate composition of the experimental diets, were thoroughly described in our previous publication (Chemello et al. Citation2020). A summary illustrating diet features, and the experimental protocol is described below.
Fish diets
A partially defatted Tenebrio molitor larvae meal (TM) provided by Ÿnsect (Evry, France) was used to formulate four isonitrogenous (CP: about 42.5% as fed), isolipidic (EE: about 24.2% as fed), and isoenergetic (GE: about 23.8 MJ/kg) experimental diets based on a control diet containing 20% of FM. The extruded diets were prepared including, as fed basis, increasing levels of a partially defatted TM (0% – TM0, 5% – TM25, 10% – TM50, and 20% – TM100) in substitution of 0, 25, 50, and 100% of FM. The experimental diets were prepared by SPAROS LDA (Olhão, Portugal) as extruded pellets.
Because of the different chemical compositions of TM and FM, the amounts of some other dietary ingredients (i.e. wheat gluten, wheat meal, and sardine oil) were slightly modified with the dietary increase of TM inclusion (Table ). Moreover, to ensure that the experimental diets fulfil the rainbow trout essential AA requirements, amino acid (AA) supplementation was included in diets. Details on the chemical composition of diets have been reported by Chemello et al. (Citation2020).
Table 1. Ingredients (g/kg) of the experimental diets.
Sampling
Two hundred and fifty-two grow-out rainbow trout (purchased from a private fish hatchery ‘Troticoltura Bassignana’; Cuneo, Italy) were slightly anaesthetised (MS-222, PHARMAQ Ltd, UK; 60 mg/L), individually weighed (78.3 ± 6.24 g) and randomly distributed into 12 400-L tanks (three replicate tanks per diet, 21 fish per tank).
At the end of a 154-day trial, spleen, kidney, liver, anterior and posterior intestine were sampled from 15 fish per each experimental diet (five fish per tank) for the histomorphological analysis. Simultaneously, fish for the MRFs gene expression analysis were randomly selected from tanks of the two extreme diets: TM0 and TM100 (100% FM and 100% TM, respectively) and killed by over anaesthesia (MS-222; PHARMAQ Ltd., UK; 500 mg/L). The same portion of dorsal white muscle was collected just below the dorsal fin of the left side (1 cm3) from each fish and stored in RNA-later (Sigma–Aldrich, Milano, Italy ) for molecular analysis, according to manufacturer’s guidelines.
Molecular analysis
RNA extraction and cDNA synthesis
Total RNA was extracted from rainbow trout dorsal muscle using Trizol (TRIzol™ reagent, Thermo Fisher Scientific) and following the manufacturer’s protocol. The isolated RNA was eluted in 60 µl RNase-free water (Sigma Aldrich) and treated with DNase I (Thermo Fisher Scientific) to remove potential DNA contamination. Final RNA concentration and quality were assessed as OD260/280 ratio (≥2.0) by Nanodrop ND-1000 Spectrophotometer (Thermo Fisher Scientific Inc., Waltham, MA, USA).
Reverse transcription was performed in a final reaction volume of 20 µl starting from 50 ng of total RNA using the Verso cDNA synthesis kit (Thermo Fisher Scientific) and following the producer's instructions. A mixture of random hexamer (400 ng/µl) and anchored oligo dT (500 ng/µl) in the ratio 3:1 was used to provide a flexible RNA priming method for cDNA synthesis. RT enhancer was also included in the cDNA reaction mixture to ensure the total lack of contaminating DNAs not degraded by DNAse I treatment.
Real-time PCR
Quantitative PCR was performed according to Gu et al. (Citation2020) using 2X QuantiTect SYBR Green PCR Master Mix in presence of ROX passive reference dye (Qiagen), 5 ng/µl of cDNA, and 0.5 µM of each primer. Analyses were carried out with a MiniOpticon Real-time PCR System (Bio-Rad) in triplicate for each RT product.
The thermal profile for all reactions was 3 min at 95 °C, followed by 40 cycles of 15 s at 95 °C, 30 s at 55 °C (annealing) and 30 s at 72 °C (extension). Fluorescence was monitored at the end of each cycle. Amplification specificity was checked using melting curves made from 55 °C to 95 °C by increasing 0.5 °C every 10 s. The genes selected were all important markers of myogenic determination and differentiation [myoblast determination protein (MyoD), myogenic factor 5 (Myf5), myogenic factor 6 (Myf6), and myogenin (Myog)], cell proliferation [proliferative cell nuclear antigen (PCNA)], involved in muscle structure and function [fast-myosin heavy chain (fMHC)] and proteolysis [cathepsin D (cathD)].
Relative quantification of the expression of the selected genes was determined according to Vandesompele et al. (Citation2002) using two [18S and elongation factor1-alpha (EF1-α)] out of three tested housekeeping genes [18S, EF1-α, and β-actin (ACTB)] as endogenous controls and the geometric mean values for normalisation as follows:
where EGOI is the efficiency of the gene of interest; ΔCt GOI is the difference between the threshold cycle of the gene of interest; GeoMean is the geometric mean; EREF is the efficiency of the reference gene; ΔCt REF is the difference between the threshold cycle of the reference gene.
Primer sequences were selected from previous publications (Table ) and synthesised by Microsynth GmbH (Vienna, Austria).
Table 2. Primers sequences and accession numbers.
Histomorphological analysis
Anterior and posterior gut samples of ∼5 cm in length were excised and flushed with 0.9% saline to remove all the content. The liver, spleen, and kidney were also collected. All the samples were fixed in 10% buffered formalin solution, embedded in paraffin wax blocks, sectioned at 5 μm thickness, mounted on glass slides, and stained with Haematoxylin & Eosin for histomorphological investigations.
A total of three serial sections were prepared for each intestinal segment and examined using light microscopy. The same slide among the serial sections was considered for morphometric analysis and then captured with a Nikon DS-Fi1 digital camera coupled to a Zeiss Axiophot microscope using a 2.5× objective lens. NIS-Elements F software was used for image capturing. The villus height (Vh) was evaluated on 10 well-oriented and intact villi per captured field (10 villi/sample) by Image®-Pro Plus software, according to Renna et al. (Citation2017). Following the same procedures firstly adopted by Biasato et al. (Citation2020) in pigs, for each gut segment, the mucosa, submucosa, and muscular layers were also separately assessed for the presence of inflammatory cells infiltrates using a semiquantitative scoring system from 0 (absence of inflammatory cells infiltration) to 3 (severe inflammatory cells infiltration). The total score of each intestinal segment was obtained by adding the mucosa, submucosa, and muscular layer scores, while the total score of each fish was represented by the mean value of the anterior and posterior gut scores. All the slides were blind assessed by three observers and the discordant cases were reviewed, using a multi-head microscope, until unanimous consensus was reached.
Liver, kidney, and spleen sections were also examined using light microscopy to identify histopathological alterations. In particular, a total of three serial sections were prepared for each sampled organ too, with the most representative one per fish being considered for histopathological examination. The observed histopathological findings were evaluated using a semiquantitative scoring system based on their distribution and severity according to Elia et al. (Citation2018): absence of alterations (score 0); focal to multifocal, mild alterations (score 1); multifocal, moderate alterations (score 2); multifocal to diffuse, severe alterations (score 3).
Statistical analysis
Statistical analyses were performed using IBM SPSS Statistics v. 25.0 for Windows. The assumption of normality was checked using the Kolmogorov–Smirnov test. Relative quantification of gene expression was analysed by Student’s t-test. Levene’s homogeneity of variance test was used to assess homoscedasticity. If such an assumption did not hold, the Brown–Forsythe statistic was applied to test the equality of group means instead of the F one.
The intestinal morphometric findings (10 measurements/slide, one slide/gut segment, two gut segments/fish, 15 fish/treatment) were analysed by fitting a general linear mixed model (GLMM). The GLMM allowed the morphometric index (Vh) to depend on three fixed factors (diet, intestinal segment, and the interaction between the diet and intestinal segment). The animal was included as a random effect to account for repeated measurements on the same fish. The interactions between the levels of the fixed factors were evaluated using pairwise comparisons. The histopathological scores (one score/slide, one slide/organ, four organs/fish, 15 fish/treatment) were analysed using the Kruskal-Wallis test (post-hoc test: Dunn’s Multiple Comparison Test).
The results were expressed as the mean (molecular and histopathological data) or least square mean (intestinal morphometric findings) and pooled standard error of the mean (SEM). Significance was set at p ≤ 0.05.
Results
Real-time PCR results
Real-time PCR analysis was performed on genes involved in fish muscle growth. Results showed that total FM replacement with TM (TM100) affected MyoD RNA level, which was significantly (p < .05) higher in TM100 samples compared to the TM0 control diet (Figure ). No further differences in gene expression were observed for the other analysed genes.
Figure 1. Relative mRNA levels of genes involved in fish myogenesis (MyoD, Myf5, Myf6, Myog, and fMHC), cell proliferation (PCNA), and proteolysis (cathD) analysed in the muscles of fish fed with TM0 and TM100. Values are presented as mean ± SEM. Asterisks indicate significant differences (p ≤ 0.05).
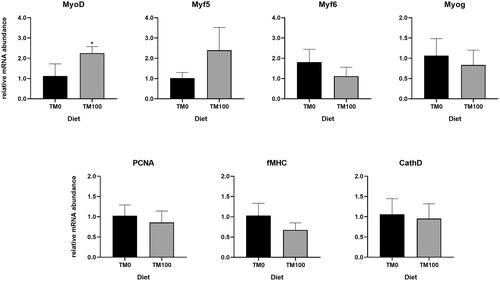
Histomorphological analysis
The Vh in the gut of the fish (Figure ) significantly depended on the gut segment (p < .001), with the posterior intestine showing higher Vh when compared to the anterior one (p < .001). On the contrary, no influence of dietary TM inclusion or interaction between the diet and the gut segment was observed on the histomorphological findings (Figure ).
Figure 2. Effects of dietary Tenebrio molitor larvae meal (TM) inclusion on rainbow trout gut morphology. (A) Villus height (Vh) (expressed in mm) in the four dietary treatments for the two intestine segments (anterior and posterior). (B) Vh (in mm) in the anterior and posterior intestine, independently of the dietary treatment considered. ** indicates p < 0.001.
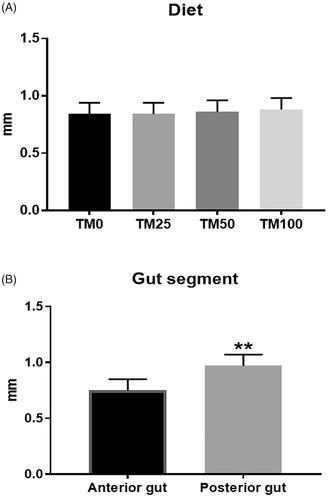
Histopathological alterations were observed in all the sampled organs for all the dietary treatments (Figure ). Gut showed mild, multifocal mucosal/submucosal lymphoplasmacytic infiltrates. Mild to moderate, multifocal lymphoplasmacytic infiltrates, as well as mild to severe, multifocal to diffuse steatosis were identified in the liver. Kidneys showed mild to moderate, multifocal interstitial lymphoplasmacytic infiltrates, while mild to moderate, focal to multifocal white pulp hyperplasia and haemosiderosis were observed in the spleen. However, dietary TM inclusion did not influence either development or severity of the observed histopathological alterations (p > .05, Table ).
Figure 3. Rainbow trout histological features stained with Haematoxylin & Eosin stain. (A) Normal liver, 20× magnification. TM25 diet. (B) Liver, 20× magnification. Diffuse, moderate to severe steatosis, as well as mild, multifocal lymphoplasmacytic perivascular infiltrates (arrowheads), are observed. TM0 diet. (C) Normal kidney, 20× magnification. TM0 diet. (D) Kidney, 20× magnification. Focal, moderate interstitial lymphoplasmacytic infiltrates (arrow) are identified. TM50 diet. (E) Normal spleen, 20× magnification. TM100 diet. (F) Spleen, 20× magnification. Focal, moderate white pulp hyperplasia (arrow), along with mild, multifocal hemosiderosis (arrowheads), are observed. TM0 diet.
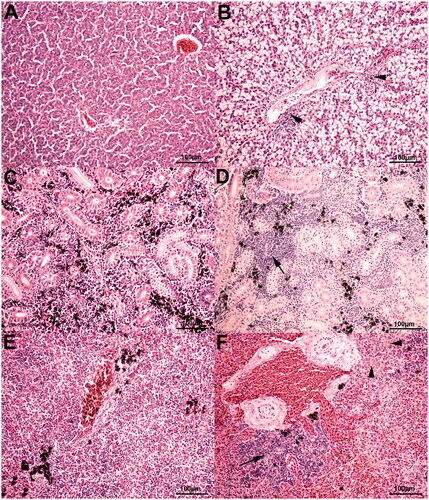
Table 3. Effects of dietary Tenebrio molitor larvae meal(TM) inclusion on the histopathological scores of the fish.
Discussion
Expression of genes involved in muscles growth
Studying the effects of insect meal-containing diets on fish growth is crucial to understanding how nutrients are involved in myogenesis regulation mechanisms.
Data presented in our previous study reporting the results of growth and digestibility trials in rainbow trout revealed that TM dietary inclusion did not negatively affect nutrients digestibility and somatic growth (Chemello et al. Citation2020). However, the lack of detrimental consequences at the somatic level may not be representative of the molecular dynamics regulating muscle growth (Chapalamadugu et al. Citation2009; Alami-Durante et al. Citation2010b). Indeed, the inclusion of 20% TM (TM100) in the rainbow trout diet significantly increased the relative expression of MyoD. It has been proved that MyoD has a specific role in post-juvenile growth in fish promoting the differentiation of activated satellite cells (Sabourin et al. Citation1999). Quiescent satellite cells display no detectable levels of MRFs expression, whereas, upon satellite cell activation, MyoD expression is rapidly up-regulated before other MRFs involved in the myogenesis process (Sabourin and Rudnicki Citation2000). Our results may suggest that TM dietary inclusion could have affected MyoD expression and indirectly, its regulatory function on satellite cells differentiation into myocytes.
It was established that changes in dietary macronutrients could alter the expression of some MRFs (Chapalamadugu et al. Citation2009; Campos et al. Citation2010; Alami-Durante et al. Citation2010b; Valente et al. Citation2016). Alami-Durante et al. (Citation2010b) observed reduced levels of MyoD expression in rainbow trout fed diet rich in soybean meal; whereas Chapalamadugu et al. (Citation2009) found that rainbow trout fed with 15 and 25% carbohydrate-containing diets had an increased transcription of MyoD, Myf5, and myogenin.
Unfortunately, neither study identified with certainty the mechanisms behind such alteration of MyoD and other MRFs expressions. Alami-Durante et al. (Citation2010b) hypothesised that the decrease of MyoD expression may be triggered by the isoflavones normally present in soybean meal that are known to inhibit the proliferation of muscle cells in mammals in vitro (Pan et al. Citation2001). While Chapalamadugu et al. (Citation2009) were not able to clarify whether dietary carbohydrates directly affected MyoD expression or if their positive impact on trout growth were mainly due to the protein-sparing effect. The up-regulation of MyoD expression has been related to the dietary amino acid deficiency as reported by several studies for different species (Michelato et al. Citation2017; Alami-Durante et al. Citation2018; Cai et al. Citation2018; Alami-Durante et al. Citation2019). However, the diets administered in our study were formulated to be isonitrogenous, isolipidic, and isoenergetic and amino acid supplementation was added to meet the fish essential amino acid requirement (Chemello et al. Citation2020). Therefore, the alteration of MyoD expression could not be related to an amino acid deficiency and the role of TM in MyoD up-regulation is still unclear and deserves further investigation.
There is documentary evidence that the expression of genes involved in myogenesis is also influenced by feed intake as reported by Alami-Durante et al. (Citation2010b). However, the results described by Chemello et al. (Citation2020) highlighted no differences in the feed intake among the experimental diets.
It is also important to specify that the existing literature concerning the effects of nutrients on the myogenesis process has been focussed mainly on alevins and juveniles fish. The grow-out rainbow trout used in our study are most likely characterised by a slower growth rate compared to younger fish and therefore muscle growth may be characterised by different regulatory mechanisms. Finally, it is interesting to consider that our results also highlighted a trend of increased Myf5 RNA level in fish fed the TM100 diet although there is no evidence of a significant alteration (p = .08). As already mentioned, Myf5 is involved in the first steps of myogenesis together with MyoD and myogenin, supporting the proliferation of satellite cells (Chapalamadugu et al. Citation2009). This observation may sustain the hypothesis of TM potential influence on myogenesis process activation.
The other genes studied—cathD, PCNA, Myf6, Myog, and fMHC—did not differ between diets, thus suggesting that dietary TM inclusion does not impact the subsequent steps of myogenesis in which these genes are involved.
Gut morphology and histopathological analysis
Dietary TM inclusion did not affect the gut morphology of the rainbow trout of the present study, thus suggesting that insect meal utilisation does not alter the nutrient digestion and absorption processes. This agrees with previous researches assessing the effects of insect-based diets on the intestinal morphometric features of the fish (Renna et al. Citation2017; Józefiak et al. Citation2019; Caimi et al. Citation2020a). Indeed, unaffected Vh was observed in rainbow trout (Renna et al. Citation2017) and juvenile Siberian sturgeon (Józefiak et al. Citation2019; Caimi et al. Citation2020a) fed different inclusion levels of HI (20–40%, Renna et al. Citation2017; 18.5–37.5%, Caimi et al. 2020b ; 15%, Józefiak et al. Citation2019) or TM (15%, Józefiak et al. Citation2019) meals when compared to the control diets. The identification of unaffected gut morphology is also in line with the unaffected growth performance previously reported by Chemello et al. (Citation2020), as a confirmation of the already mentioned influence of the gut health status on the animal performance.
Independently of TM utilisation, the posterior intestine also showed longer villi than the anterior segment. This appears to be in contrast with Khojasteh et al. (Citation2009), which reported progressively shorter villi towards the posterior intestine. However, Verdile et al. (Citation2020) recently reported a detailed characterisation of the epithelial cells lining the intestinal mucosa in rainbow trout, simultaneously observing short and long villi in both the anterior and the posterior gut. The same authors also reported that the long villi length in the proximal intestine increased when the fish reached their highest weight (with the short villi length remaining constant), while the distal intestine showed similar villi length along with the fish development (Verdile et al. Citation2020). Therefore, the identification of differences between the two gut compartments may be related to either the simultaneous presence of short and long villi or the villi length changes throughout the fish cycle. Another interesting aspect to underline is that Verdile et al. (Citation2020) also observed a higher mucosa volume in the posterior than in the anterior intestine, thus herein suggesting a potential role of the villi (which are the major component of the gut mucosa) that deserves future investigations.
Dietary TM inclusion did not influence the histological features of the gut, the liver, the kidney, and the spleen of the fish of the current trial, thus confirming that TM meal utilisation up to 20% of dietary inclusion does not cause the development of specific pathologies. Indeed, the histopathological alterations were identified in both the control- and the TM-fed fish, also resulting to be predominantly mild to moderate. Furthermore, similar histopathological findings were also observed in rainbow trout fed either the FM- or the HI-based diets (Elia et al. Citation2018). The identification of histopathological alterations in the control group is not indicative of altered health status of the fish, as liver, gut, and spleen lesions were considered paraphysiological (attributable to the intensive feeding and farming of rainbow trout), and kidney findings were too non-specific to be related to any infectious and/or metabolic disorders. Furthermore, the fish fed the basal diet more than triplicated their initial weight, also displaying a condition’s factor indicative of a good physiological state of well-being, and either the hepatosomatic index or the hepatic enzymatic activity suggestive of unaltered liver functionality (Chemello et al. Citation2020).
Conclusions
The present study reported, for the first time, preliminary results describing the influence of TM dietary inclusion on rainbow trout myogenesis. Although the mechanism through which TM influenced the expression of MyoD and satellite cell activation needs to be further analysed, we obtained a first insight into the complex mechanism that regulates fish myogenesis. These results may represent the starting point for further studies that could consider the muscle growth dynamics (such as distribution of muscle fibres size) through histomorphological analyses. Moreover, after observing MyoD up-regulation in fish fed TM100, it will be interesting to detect the threshold level of TM dietary inclusion that affects MyoD expression and may influence muscle morphology.
Finally, our results highlighted the absence of variation in gut morphology and other organs (liver, kidney, and spleen) histopathology, confirming the safe utilisation of TM as an alternative protein source in rainbow trout diets.
Acknowledgements
The authors acknowledge Dario Sola, Mario Colombano, and Alessandra Sereno for technical support, and Ÿnsect (Evry, France) for the provision of the Tenebrio molitor larvae meal and diets.
Ethical approval
The experimental protocol was approved by the Ethical Committee of University of Turin (protocol n° 143811) and was designed according to European and Italian guidelines on the care and use of experimental animals (European directive 86 609/EEC, put into 119 law in Italy with D.L. 116/92).
Disclosure statement
No potential conflict of interest was reported by the author(s).
Additional information
Funding
References
- Alami-Durante H, Bazin D, Cluzeaud M, Fontagné-Dicharry S, Kaushik S, Geurden I. 2018. Effect of dietary methionine level on muscle growth mechanisms in juvenile rainbow trout (Oncorhynchus mykiss). Aquaculture. 483:273–285.
- Alami-Durante H, Cluzeaud M, Bazin D, Schrama JW, Saravanan S, Geurden I. 2019. Muscle growth mechanisms in response to isoenergetic changes in dietary non-protein energy source at low and high protein levels in juvenile rainbow trout. Comp Biochem Physiol A Mol Integr Physiol. 230:91–99.
- Alami-Durante H, Cluzeaud M, Duval C, Maunas P, Girod-David V, Médale F. 2014. Early decrease in dietary protein: energy ratio by fat addition and ontogenetic changes in muscle growth mechanisms of rainbow trout: short- and long-term effects. Br J Nutr. 112(5):674–687.
- Alami-Durante H, Médale F, Cluzeaud M, Kaushik SJ. 2010a. Skeletal muscle growth dynamics and expression of related genes in white and red muscles of rainbow trout fed diets with graded levels of a mixture of plant protein sources as substitutes for fishmeal. Aquaculture. 303(1–4):50–58.
- Alami-Durante H, Wrutniak-Cabello C, Kaushik SJ, Médale F. 2010b. Skeletal muscle cellularity and expression of myogenic regulatory factors and myosin heavy chains in rainbow trout (Oncorhynchus mykiss): effects of changes in dietary plant protein sources and amino acid profiles. Comp Biochem Physiol A Mol Integr Physiol. 156(4):561–568.
- Antonopoulou E, Nikouli E, Piccolo G, Gasco L, Gai F, Chatzifotis S, Mente E, Ar Kormas K. 2019. Reshaping gut bacterial communities after dietary Tenebrio molitor larvae meal supplementation in three fish species. Aquaculture. 503:628–635.
- Biasato I, Ferrocino I, Colombino E, Gai F, Schiavone A, Cocolin L, Vincenti V, Capucchio MT, Gasco L. 2020. Effects of dietary Hermetia illucens meal inclusion on cecal microbiota and small intestinal mucin dynamics and infiltration with immune cells of weaned piglets. J Animal Sci Biotechnol. 11(1):64.
- Bjornevik M, Beattie C, Hansen T, Kiessling A. 2003. Muscle growth in juvenile Atlantic salmon as influenced by temperature in the egg and yolk sac stages and diet protein level. J Fish Biol. 62(5):1159–1175.
- Borgogno M, Dinnella C, Iaconisi V, Fusi R, Scarpaleggia C, Schiavone A, Monteleone E, Gasco L, Parisi G. 2017. Inclusion of Hermetia illucens larvae meal on rainbow trout (Oncorhynchus mykiss) feed: effect on sensory profile according to static and dynamic evaluations. J Sci Food Agric. 97(10):3402–3411.
- Buckingham M, Rigby PWJ. 2014. Gene regulatory networks and transcriptional mechanisms that control myogenesis. Dev Cell. 28(3):225–238.
- Cai WC, Liu WB, Jiang GZ, Wang KZ, Sun CX, Li XF. 2018. Lysine supplement benefits the growth performance, protein synthesis, and muscle development of Megalobrama amblycephala fed diets with fish meal replaced by rice protein concentrate. Fish Physiol Biochem. 44(4):1159–1174.
- Caimi C, Gasco L, Biasato I, Malfatto V, Varello K, Prearo M, Pastorino P, Bona MC, Francese DR, Schiavone A, et al. 2020a. Could dietary black soldier fly meal inclusion affect the liver and intestinal histological traits and the oxidative stress biomarkers of Siberian sturgeon (Acipenser baerii) juveniles? Animals. 10(1):155.
- Caimi C, Renna M, Lussiana C, Bonaldo A, Gariglio M, Meneguz M, Dabbou S, Schiavone A, Gai F, Elia AC, et al. 2020b. First insights on Black Soldier Fly (Hermetia illucens L.) larvae meal dietary administration in Siberian sturgeon (Acipenser baerii Brandt) juveniles. Aquaculture. 515:734539.
- Campos C, Valente LMP, Borges P, Bizuayehu T, Fernandes JMO. 2010. Dietary lipid levels have a remarkable impact on the expression of growth-related genes in Senegalese sole (Solea senegalensis Kaup). J Exp Biol. 213(2):200–209.
- Canada P, Engrola S, Mira S, Teodósio R, Yust M. d M, Sousa V, Pedroche J, Fernandes JMO, Conceição LEC, Valente LMP. 2018. Larval dietary protein complexity affects the regulation of muscle growth and the expression of DNA methyltransferases in Senegalese sole. Aquaculture. 491:28–38.
- Chapalamadugu KC, Robison BD, Drew RE, Powell MS, Hill RA, Amberg JJ, Rodnick KJ, Hardy RW, Hill ML, Murdoch GK. 2009. Dietary carbohydrate level affects transcription factor expression that regulates skeletal muscle myogenesis in rainbow trout. Comp Biochem Physiol B Biochem Mol Biol. 153(1):66–72.
- Chemello G, Renna M, Caimi C, Guerreiro I, Oliva-Teles A, Enes P, Biasato I, Schiavone A, Gai F, Gasco L. 2020. Partially defatted Tenebrio molitor larva meal in diets for grow-out rainbow trout, Oncorhynchus mykiss (Walbaum): effects on growth performance, diet digestibility and metabolic responses. Animals. 10(2):229.
- Elia AC, Capucchio MT, Caldaroni B, Magara G, Dörr AJM, Biasato I, Biasibetti E, Righetti M, Pastorino P, Prearo M, et al. 2018. Influence of Hermetia illucens meal dietary inclusion on the histological traits, gut mucin composition and the oxidative stress biomarkers in rainbow trout (Oncorhynchus mykiss). Aquaculture. 496:50–57.
- Ferrer Llagostera P, Kallas Z, Reig L, Amores de Gea D. 2019. The use of insect meal as a sustainable feeding alternative in aquaculture: current situation, Spanish consumers’ perceptions and willingness to pay. J Clean Prod. 229:10–21.
- Fontagné-Dicharry S, Lataillade E, Surget A, Larroquet L, Cluzeaud M, Kaushik S. 2014. Antioxidant defense system is altered by dietary oxidized lipid in first-feeding rainbow trout (Oncorhynchus mykiss). Aquaculture. 424–425:220–227.
- Gasco L, Gai F, Maricchiolo G, Genovese L, Ragonese S, Bottari T, Caruso G. 2018. Fishmeal Alternative Protein Sources for Aquaculture Feeds. In: Springer International Publishing, editor. Feeds for the Aquaculture Sector. Cham: Springer. p. 1–28.
- Gauvry L, Fauconneau B. 1996. Cloning of a trout fast skeletal myosin heavy chain expressed both in embryo and adult muscles and in myotubes neoformed in vitro. Comp Biochem Physiol B Biochem Mol Biol. 115(2):183–190.
- Gu M, Cosenza G, Gaspa G, Iannaccone M, Macciotta NPP, Chemello G, Di Stasio L, Pauciullo A. 2020. Sequencing of lipoprotein lipase gene in the Mediterranean river buffalo identified novel variants affecting gene expression. J Dairy Sci. 103(7):6374–6382.
- Henry MA, Gai F, Enes P, Peréz-Jiménez A, Gasco L. 2018b. Effect of partial dietary replacement of fishmeal by yellow mealworm (Tenebrio molitor) larvae meal on the innate immune response and intestinal antioxidant enzymes of rainbow trout (Oncorhynchus mykiss). Fish Shellfish Immunol. 83:308–313.
- Henry MA, Gasco L, Chatzifotis S, Piccolo G. 2018a. Does dietary insect meal affect the fish immune system? The case of mealworm, Tenebrio molitor on European sea bass, Dicentrarchus labrax. Dev Comp Immunol. 81:204–209.
- Hevrøy EM, Jordal AEO, Hordvik I, Espe M, Hemre GI, Olsvik PA. 2006. Myosin heavy chain mRNA expression correlates higher with muscle protein accretion than growth in Atlantic salmon, Salmo salar. Aquaculture. 252(2–4):453–461.
- Johnston IA. 1999. Muscle development and growth: Potential implications for flesh quality in fish. Aquaculture. 177(1–4):99–115.
- Józefiak A, Nogales-Mérida S, Rawski M, Kierończyk B, Mazurkiewicz J. 2019. Effects of insect diets on the gastrointestinal tract health and growth performance of Siberian sturgeon (Acipenser baerii Brandt, 1869. BMC Vet Res. 15(1):348.
- Khojasteh SMB, Sheikhzadeh F, Mohammadnejad D, Azami A. 2009. Histological, histochemical and ultrastructural study of the intestine of rainbow trout (Oncorhynchus mykiss). World Appl Sci J. 6(11):1525–1531.
- Lock EJ, Biancarosa I, Gasco L. 2018. Insects as raw materials in compound feed for aquaculture. In: Springer International Publishing, editor. Edible insects in sustainable food systems. Cham: Springer. p. 263–276.
- Michelato M, Zaminhan M, Boscolo WR, Nogaroto V, Vicari M, Artoni RF, Rossetto Barriviera Furuya V, Massamitu Furuya W. 2017. Dietary histidine requirement of Nile tilapia juveniles based on growth performance, expression of muscle-growth-related genes and haematological responses. Aquaculture. 467:63–70.
- Moghadam HK, Ferguson MM, Rexroad CE, Coulibaly I, Danzmann RG. 2007. Genomic organization of the IGF1, IGF2, MYF5, MYF6 and GRF/PACAP genes across Salmoninae genera. Anim Genet. 38(5):527–532.
- Ostaszewska T, Dabrowski K, Wegner A, Krawiec M. 2008. The effects of feeding on muscle growth dynamics and the proliferation of myogenic progenitor cells during Pike Perch development (Sander lucioperca). J World Aquaculture Soc. 39(2):184–195.
- Pan W, Ikeda K, Takebe M, Yamori Y. 2001. Genistein, daidzein and glycitein inhibit growth and DNA synthesis of aortic smooth muscle cells from stroke-prone spontaneously hypertensive rats. J Nutr. 131(4):1154–1158.
- Renna M, Schiavone A, Gai F, Dabbou S, Lussiana C, Malfatto V, Prearo M, Capucchio MT, Biasato I, Biasibetti E, et al. 2017. Evaluation of the suitability of a partially defatted black soldier fly (Hermetia illucens L.) larvae meal as ingredient for rainbow trout (Oncorhynchus mykiss Walbaum) diets. J Anim Sci Biotechnol. 8:57.
- Rimoldi S, Gini E, Iannini F, Gasco L, Terova G. 2019. The effects of dietary insect meal from Hermetia illucens Prepupae on autochthonous gut microbiota of rainbow trout (Oncorhynchus mykiss). Animals. 9(4):143.
- Roques S, Deborde C, Guimas L, Marchand Y, Richard N, Jacob D, Skiba-Cassy S, Moing A, Fauconneau B. 2020. Integrative metabolomics for assessing the effect of insect (Hermetia illucens) protein extract on rainbow trout metabolism. Metabolites. 10(3):83.
- Rowlerson A, Veggetti A. 2001. Cellular mechanisms of post-embryonic muscle growth in aquaculture species. In: Johnston IA, editor. Muscle development and growth (fish physiology 18). San Diego: Elsevier Science. p. 103–140.
- Sabourin LA, Girgis-Gabardo A, Seale P, Asakura A, Rudnicki MA. 1999. Reduced differentiation potential of primary MyoD/myogenic cells derived from adult skeletal muscle. J Cell Biol. 144(4):631–643.
- Sabourin LA, Rudnicki MA. 2000. The molecular regulation of myogenesis. Clin Genet. 57(1):16–25.
- Silva P, Valente LMP, Galante MH, Andrade CAP, Monteiro RAF, Rocha E. 2009. Dietary protein content influences both growth and size distribution of anterior and posterior muscle fibres in juveniles of Pagellus bogaraveo (Brunnich). J Muscle Res Cell Motil. 30(1–2):29–39.
- Valente LMP, Cabral EM, Sousa V, Cunha LM, Fernandes JMO. 2016. Plant protein blends in diets for Senegalese sole affect skeletal muscle growth, flesh texture and the expression of related genes. Aquaculture. 453:77–85.
- Vandesompele J, De Preter K, Pattyn F, Poppe B, Van Roy N, De Paepe A, Speleman F. 2002. Accurate normalization of real-time quantitative RT-PCR data by geometric averaging of multiple internal control genes. Genome Biol. 3: 1–12.
- Verdile N, Pasquariello R, Scolari M, Scirè G, Brevini TAL, Gandolfi F. 2020. A detailed study of rainbow trout (Oncorhynchus mykiss) intestine revealed that digestive and absorptive functions are not linearly distributed along its length. Animals. 10(4):745.
- Vo TA, Galloway TF, Bardal T, Halseth CK, Øie G, Kjørsvik E. 2016. Skeletal muscle growth dynamics and the influence of first-feeding diet in Atlantic cod larvae (Gadus morhua L.). Biol Open. 5(11):1575–1584.