Abstract
Weaning transition is often associated with the occurrence of the post-weaning diarrhoea syndrome (PWDS). The reduction of dietary crude protein (CP) has been intensively used as a strategy for controlling PWDS. The aim of the present meta-analysis was to report the effect of the reduction of dietary CP on the intestinal parameters related to the gut health, growth and diarrhoea of post-weaning piglets. A literature review of the articles published from 2006 to 2019 produced 48 articles and, of these 26 were selected. Parameters (bacterial metabolites, pH, microbiota diversity, intestinal morphology, inflammation markers, growth, faecal score) were extracted, expressed as a percentage of the control diet and analysed using a general linear model which included the study, reduction in points of dietary CP, and the ratio of digestible Lysine and dietary CP (dLys/CP) as factors. The reduction of dietary CP decreased ammonia (p < .0001), pH (p = .039), total short-chain fatty acids (SCFAs) (p = .027), biogenic amines including cadaverine (p = .034) and putrescine (p = .030) and the expression of TLR4 (p < .0001) in the intestine and also reduced the faecal score (p = .002). The dLys/CP ratio increased the level of Lactobacilli and the expression of TLR4 (p < .05), and tended to increase acetic acid (p < .1). Crypt depth, villus height, microbiota diversity and growth remained unchanged. In conclusion, this study confirmed that a reduction of dietary CP could reduce bacterial protein fermentation and the production of potentially toxic metabolites. In turn, this could result in lower intestinal inflammation and a decreased risk of diarrhoea in weaning piglets.
Reducing the dietary crude protein can decrease the fermentation of undigested dietary protein, especially in the large intestine.
Lowering undigested dietary protein results in a reduction of the intestinal pH and potentially toxic metabolites, including ammonia and amines.
Diets with lower crude protein reduce inflammation of the intestinal mucosa and the post-weaning diarrhoea of piglets without compromising performance.
Highlights
Graphical Abstract
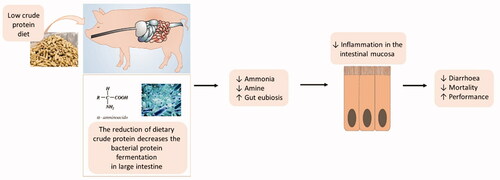
Introduction
Weaning has been recognised to be one of the most critical phases of the pig rearing system owing to a number of stressor factors, including social stress, change of diet and environment, coupled with the immature digestive tract and immature immune system of the piglets. Therefore, post-weaning period is often associated with the occurrence of post-weaning diarrhoea syndrome (PWDS). Currently, several nutritional and feeding strategies have been considered to maintain and restore piglet intestinal health during the weaning transition period, and to improve the robustness of the piglets. Of the nutritional strategies available to counteract post-weaning diarrhoea (PWD), the reduction of the dietary crude protein (CP) level in the post-weaning diet has been widely adopted, mainly thanks to commercially available feed-grade amino acids (AAs) (Gao et al. Citation2019; Heo et al. Citation2013). In fact, starting from weaning, piglets require a high nutritional level of AAs in order to ensure recovery from the stressors associated with weaning as well as for the correct development of the intestine, and also to sustain immune response and growth. Thus, the reduction of dietary CP can represent a risk and must always be coupled with the inclusion of a sufficient quantity of essential and conditionally essential AAs in the feed formula (Le Floc’h et al. Citation2018).
In the past, several studies, reviews and meta-analyses suggesting the beneficial effects of reducing the level of CP in the diet of piglets in terms of economic cost (Wang et al. Citation2018), gut health (Wang et al. Citation2018; Pluske et al. Citation2002), and environmental impact have been carried out. In fact, high-protein (HP) diets can lead to excesses of undigested or unabsorbed peptides and AAs, lower nitrogen efficiency, and higher excretion of ammonia and nitrogen in faeces and urine, resulting in a higher environmental impact (Sajeev et al. Citation2018; Wang et al. Citation2020). Focussing on gut health, HP diets can favour protein fermentation in the hindgut which can alter the gut mucosal homeostasis. In fact, the positive effects associated with the reduction of dietary CP have been ascribed to reduced intestinal fermentation of undigested protein and AAs by the bacteria in the distal part of the small intestine and in the large intestine (Pieper et al. Citation2016). Protein fermentation by bacteria can result in the production of several metabolites including short-chain fatty acids (SCFAs), branched-chain fatty acids (BCFAs), ammonia, polyamines, sulfur-containing bacterial metabolites (methanethiol, H2S) and aromatic compounds (phenolic, indolic) which can be considered rather beneficial or which can disturb intestinal homeostasis (Pieper et al. Citation2016; Wang et al. Citation2020). On the other hand, low CP diets can be deficient in AAs which are essential for maintaining gut health (Yang and Liao Citation2019).
Although numerous studies have been carried out, little is still known regarding the effect of the reduction of dietary CP on the intestinal parameters associated with gut health, including the intestinal barrier function, tight junction, microbiota profile and SCFA composition (Diether and Willing Citation2019; Heo et al. Citation2013). Thus, the primary aim of the present meta-analysis was to report the effect of the reduction of dietary CP on the intestinal parameters related to gut health in post-weaning piglets; it also reported its effect on growth and diarrhoea.
Material and methods
Data collection and methods of analyses
A systematic literature review was carried out using peer-reviewed articles published between 2006 and 2019. This framework was chosen to have studies in which the reduction of dietary CP was balanced as possible by the addition of common essential amino acids and that the parameters expected to be the object of the meta-analysis were considered. The online database “Pubmed” was used to search for the relevant articles by combining keywords related to diet ["Low protein diet" OR "Low protein level "OR" Decreased protein level "OR" Reduced protein level "] AND to health [" Immunity "OR" Gut health "OR" microbiota "OR" diarrhoea "OR" microflora "] AND to the species ["Pigs" OR "piglets"].
Once all the articles were collected, only those fulfilling the following criteria were selected: a) in-vivo swine studies including a control group and a reduced level of protein group in post-weaning pigs, b) published in English and c) reporting at least one of the parameters included in Table .
Table 1. Parameters considered in order for inclusion of the study in the meta-analysis.
For all the articles included, the diet composition of the different experimental groups was recalculated using EvaPig software, a widely used calculator of nutritional values of ingredients and diets for piglets and sows (Noblet et al. Citation2008). This step was performed because several studies did not report the analysis of the nutrient composition of the diet and therefore to homogenise the error in the estimation of the nutrient composition of the diet all the formulations of the studies were recalculated with respect to a single database. EvaPig includes a database of the chemical composition and nutritive values of approximately 100 ingredients taken from the INRA-AFZ Tables (Sauvant et al. Citation2002, Citation2004).
The experimental groups in the articles were then defined as follows: control group (CO), namely the group with the highest CP level within the study, and the reduced CP group (RD), namely, the groups in which the dietary CP was reduced within a study (some studies had more than one type of diet). The reduction of dietary CP (DIFF CP) was expressed as the reduction of crude protein in percentage points (pts%) between the CO and the RD groups within each study. A minimum of 2 pts% between the CO and the RD groups was considered to be the cut-off for including the study in the analysis. The ratio between digestible lysine (dLys) and percentage of dietary CP (dLys/CP) was calculated for each diet as an indicator of the protein quality as Lys is considered to be the first limiting AA in pigs.
The average daily gain (ADG) was calculated for the studies when information regarding body weight and duration of the experiment was available. Data regarding the faecal score were standardised among the studies according to the following 1 to 4 scale: 1, normal faeces; 2, soft faeces; 3, mild diarrhoea and 4, severe diarrhoea (Pedersen and Strunz, Citation2013). All response data regarding piglets were expressed as relative to that observed in the CO group.
Statistical analysis
As a first step, a statistical analysis aimed at evaluating the effect of the reduction of dietary CP on the diet nutrient components was carried out. The variability in the nutrient composition of the CO and the RD diets was analysed carrying out Pearson correlation among the nutrient components. Subsequently, a general linear model (GLM) including the study (general encoding) and the DIFF CP as factors was carried out.
As a second step, a statistical analysis aimed at evaluating the effect of the reduction of dietary CP on health parameters, growth performance and faecal score was carried out. GLM model including the study (general encoding), the DIFF CP, the level of the dLys/CP ratio, the intestinal tract (only for ammonia and pH) and age of the animals (only for the ADG and diarrhoea) was carried out. All the statistical analyses were carried out using R (version 3.6.0; Citation2019) and Minitab 18 Statistical Software (Citation2018) software.
Results and discussion
Forty-eight searches on Pubmed using the defined keywords were carried out. One thousand nine hundred and eighteen references were obtained. These references were screened as described in the flow chart (Figure ). Thirty-four studies coming from twenty-six peer-reviewed articles were included. In fact, several articles included more than one RD group (Supplementary Table 1). The articles collected were published between 2006 and 2019. In the studies included, the average initial age of the piglets was 25.83 ± 8.43 days and the average initial body weight was 8.11 ± 3.92 kg (Supplementary Table 1). Supplementary Figure 1 reports the percentage of dietary CP in the CO and the RD groups in the studies included. The overall average percentage of dietary CP was 18.76% ± 2.81% while the average dietary CP was 21.40% ± 1.51% in the CO groups and 16.84% ± 1.81%in the RD groups. The average DIFF CP between the CO and the RD groups was 4.60 pts% ± 2.04 pts%.
The effect of the reduction of dietary crude protein on the nutrient composition of diets
The results of the correlation analysis among the components of the diets are reported in Supplementary Table 2. According to the correlation analysis (r = −0.49; p < .0001), the linear regression model showed a significant (p < .001; coefficient = −1.49) and negative association between the protein and starch percentages, which was consistent among the studies investigated (Supplementary Figure 2). These results suggested that the level of protein was reduced by its substitution with starch products, mainly represented by cereals. On the other hand, no significant correlation or effect in the regression model was observed between the dietary CP percentage and the level of fibre, neutral detergent fibre (NDF) and acid detergent fibre (ADF), suggesting that the formulas of the diets of the CO and the RD groups were well calculated. Furthermore, no significant correlation or effect in the regression model was observed between dietary CP percentage and the level of Ca, P and their ratio while the dLys/CP ratio was significantly and negatively correlated (r = −0.451, p < .0001), and was associated with the dietary CP percentage (p = .001). These latest results indicated that, overall, the dLys levels in the reduced CP groups were balanced as compared with the CO groups in the studies included.
Moreover, some AAs were particularly low as compared to the AA recommendation. The reduction of dietary CP in experimental diets was often obtained without fully considering the ideal protein profile; in fact, in some studies, some AAs (dThr, dTrp and dVal) in the diets of the RD groups were particularly low as compared with the AA recommendation reported by Gloaguen et al. (Citation2013).
The effect of the dietary protein level on intestinal microbiota
The intestinal microbiota is known to play a pivotal role in preventing infections while all the implications derived from the host-microbiota interplay have still not been sufficiently clarified, especially for the gut-brain axis as well as for pig welfare and behaviour (Kraimi et al. Citation2019). Several factors have been known to contribute to modifying the intestinal microbial profile and, of them, diet has been proven to exert a rapid and time lasting effect (David et al. Citation2014; Carmody et al. Citation2015). In the present meta-analysis, some parameters were selected as indicators of intestinal microbial homeostasis, such as the alpha diversity indices, the abundance of Lactobacilli and Enterobacteriaceae, and the Firmicutes/Bacteroides ratio. In fact, regarding the alpha diversity indices (Chao1, Shannon and Simpson indices), a decrease in their values during the post-weaning period has been associated with dysbiosis (Gresse et al. Citation2017). The abundance of Lactobacilli and Enterobacteriaceae in the intestinal content and faeces has been considered to be a marker of intestinal microbial homeostasis as these families are known to include bacteria considered to be beneficial for intestinal microbial homeostasis and potentially harmful for the host, respectively (Ducatelle et al. Citation2018; Lebeer et al. Citation2008). The Firmicutes/Bacteroides ratio has generally been recognised to be a marker of gut microbial homeostasis; in fact, a decrease in the Firmicutes/Bacteroides ratio is regarded as dysbiosis while an optimal Firmicutes/Bacteroides ratio is associated with good gut health and better growth performance (Mariat et al. Citation2009; Mulder et al. Citation2009; Salaheen et al. Citation2017). The meta-analysis showed that the reduction of dietary CP did not affect the alpha diversity indices (Chao1, Shannon and Simpson indices), the abundance of Lactobacilli and of Enterobacteriaceae and the Firmicutes/Bacteroides ratio in the gut (Table ). The literature data are not consistent regarding the effect of reducing dietary protein on gut microbial balance and bacterial abundancy. In agreement with the present study, Zhou et al. (Citation2016) did not find any effect on the diversity index when CP was reduced by 3% in the diet of growing barrows. However, the authors suggested that adaptation of the gut microbiota to a lower dietary protein level may occur after a relatively long period of low CP diet (45 days). Pollock et al. (Citation2019) suggested that the effect of the reduction of dietary CP on the gut microbiota could vary according to the part of the intestinal tract since the authors observed a more significant effect for the microbial profile in the ileum than in the faeces. On the other hand, several studies based on next generation sequencing have shown a significant effect of dietary protein level on the gut microbial profile and bacterial abundancy of growing pigs (Chen et al. Citation2018; Fan et al. Citation2017; Yu et al. Citation2019; Zhou et al. Citation2016). Differences among the results of the studies in the literature could be due to a different reduction of dietary CP pts%, the duration of the feeding regimes and/or the age of the animals. Furthermore, the fact that the microbial parameters may have some limitations should be considered. First of all, a taxonomic analysis fails to discern live or dead microorganisms; thus, it is an indication of but not a full expression of the metabolic potential of the bacterial community. Second, although taxonomical entities, i.e., phylum and family level, are considered to be potential markers, their analysis does not allow understanding the differences of individual species within them and does not consider the redundancy of the metabolic capacity of microbes. Therefore, these limitations could affect the data interpretation (Langille Citation2018; Trevisi et al. Citation2021); additional studies are needed to investigate the effect of dietary protein on gut microbial functions.
Table 2. The effect of dietary crude protein level on intestinal microbiota of post-weaning pigs.
Interestingly, after the adjustment for the dietary CP content in the statistical model, a significant correlation of the dLys/CP ratio with the abundance of Lactobacilli in the gut was seen (p = .004; coefficient = 25.44). Under some specific conditions, Lactobacilli show decarboxylase activity against acids (Arena and Manca de Nadra Citation2001); however, no specific evidence for the requirement of Lys for the growth of Lactobacilli in the pig intestine was found in the literature. Infusion with Lactobacillus plantarum did not increase the disappearance of Lys over 72 h in liquid feed formulated for pigs (Niven et al. Citation2006). Conversely, it cannot be excluded that other microorganisms were favoured by an increased presence of other AAs in the case of a lower dLys/CP ratio.
The effect of dietary protein level on intestinal pH and microbial fermentation products
pH
The results of the GLM showed that a reduction in dietary CP could significantly reduce the pH in the large intestine of piglets (p = .013; coefficient = −0.43), indicating that each DIFF CP point reduced the value of the pH by 0.43 while no effect was observed in the small intestine (Table ). Protein feedstuffs are known to have a higher acid-buffering capacity as compared to cereal and cereal by-products (Giger-Reverdin et al. Citation2002); thus, a reduction of dietary CP resulted in a reduced buffering effect and a lower intestinal pH (Gao et al. Citation2019). In addition, a decrease in intestinal pH due to the reduction of dietary CP could indicate lower fermentation of the proteins and AAs by the intestinal proteolytic microbes as has been previously suggested by Ma et al. (Citation2017) and Zhang et al. (Citation2020). A low intestinal pH is known to favour the development and colonisation of the gut by beneficial bacteria and to reduce the risk of invasion by harmful bacteria (Fuller Citation1977). The different results obtained for the large and small intestine can be explained by the fact that a higher bacterial fermentation of proteins and AAs would occur in the large intestine than in the small intestine. In fact, in the small intestine, a large part of the AAs can be absorbed by the host after digestion while, in the large intestine, the bacterial fermentation can be favoured by better conditions, such as the subacid pH (approximately 6 in the colon) which can promote the activation of bacterial deaminases (Pieper et al. Citation2016; Blachier et al. Citation2007) due to the longer transit time (Wen et al. Citation2018) and by the higher number of present bacteria (Gresse et al. Citation2019).
Table 3. The effect of the dietary crude protein level on the intestinal pH and microbial fermentation products of post-weaning pigs.
Ammonia
Ammonia produced by improper pig management still remains one of the main factors associated with air pollution (Wang et al. Citation2020). Ammonia is produced by bacteria by means of the deamination of AAs and, to a lesser extent, by means of the bacterial urease activity on urea (Windey et al. Citation2012). The results of the GLM showed that a reduction of dietary CP could significantly reduce the amount of ammonia in the large intestine of piglets (p = .002; coefficient = −4.10) while no effect was observed in the small intestine (p > .1) (Table ). It is, however, interesting to note that in the small intestine, the level of ammonia was significantly influenced by the dLys/CP ratio (p = .037, coefficient = −9.19) (Table ). Overall, these results confirmed that a reduction of dietary CP could contribute to a reduction of ammonia production in the piglet intestine as suggested by Diether and Willing (Citation2019), and Zhang et al. (Citation2020). These results are in agreement with previous meta-analyses and life cycle analyses (LCAs) which observed a reduction of ammonia concentration in the slurry with a reduction of dietary protein (Wang et al. Citation2018; Sajeev et al. Citation2018; Wang et al. Citation2020); in addition to that, the present meta-analysis clearly confirmed that ammonia was reduced not only in the slurry but also in the large intestine of piglets. In the intestine, ammonia can be used by bacteria for their own metabolism, for the synthesis of protein, or it can be used by the host. In the host, the ammonia can be absorbed by the colonocytes, transformed into urea in the liver and then excreted in the urine (Windey et al. Citation2012). However, little is known regarding the potential effect of ammonia on gut health, and some contrasting results have been reported in vitro. For instance, an infusion of ammonium chloride aided the proliferation of the epithelial cells isolated from the colon of rats (Ichikawa and Sakata Citation1998) while exposure to NH4Cl (50 mM) for 4 h did not modify the cell proliferation of isolated pig colonic crypts (Leschelle et al. Citation2002). More recently, ammonia was found to provoke an inhibition of the oxidative metabolism of SCFAs (especially acetate oxidation) (Cremin et al. Citation2003) and a dose-dependent reduction of oxygen consumption in the isolated absorbing colonocytes in mice fed a high protein diet; this could contribute to decreased cellular energy production (Andriamihaja et al. Citation2010).
Intestinal ammonia and pH are closely connected. In fact, the bacterial production of ammonia is known to vary according to the pH of the intestinal tract (Vince et al. Citation1973), and the pH itself can be determined according to the level of urea hydrolysis, ammonia nitrogen, volatile fatty acids and the dietary electrolyte balance (Canh et al. Citation1998); thus, the same pattern of variation as for pH and ammonia was expected with the reduction of dietary CP.
Short-chain fatty acids
Short-chain fatty acids are molecules with seven carbon atoms or less which are mainly produced by the fermentation of indigestible carbohydrates and proteins by the gut microbiota. The present meta-analysis collected 8 studies which reported data regarding total SCFAs, namely 11 for acetic acid and propionic acids, 6 for isobutyric acid, 10 for butyric acid, 6 for isovaleric acid and 7 for valeric acid. The results obtained showed that the reduction of dietary CP significantly reduced the quantity of total SCFAs in the intestinal tract of weaned piglets (p = .027; coefficient = −1.72) and, in particular, the quantity of acetic acid (p = .004; coefficient = −3.25) (Table ). The production of SCFAs from dietary protein sources derives from the metabolisation of the carbon skeleton which, in turn, is derived from the microbial deamination of the AAs; it is known that, of the different SCFAs, the microbial utilisation of AAs strongly contributes to the production of acetate (Friend et al. Citation1963). Although the SCFAs generated by means of proteolytic fermentation are considered to be lower as compared to the quantity which is generated from carbohydrate fermentation (Aguirre et al. Citation2016), it follows that the reduction of dietary CP resulted in a reduction of SCFAs and acetate due to a reduction of protein fermentation; this is in agreement with the results obtained for ammonia. It is interesting to note that the quantity of acetate in the gut tended to be positively associated with the dLys/CP ratio (p = .072; coefficient = 4.41) (Table ); as acetate can derive from the microbial utilisation of specific AAs (alanine, aspartate, glycine, threonine, lysine) (KEEG pathways: map00010, map00430, map00620, map00660, map01110, map01120), the increase in acetate in relation to the level of the dLys/CP ratio would be reasonable. In line with this, the intestinal level of propionic acid also tended to increase according to the dLys/CP ratio (p = .093; coefficient = 7.88). For the host, acetate is a precursor for the fatty acid systems, and propionate is mainly utilised for gluconeogenesis in the liver. However, the effects of the SCFAs on the intestinal mucosa can vary according to age and concentration. On the one hand, SCFAs ensure energy for the cells; on the other hand, an abnormal level in newborns increases inflammation leading to mucosal injury (Lin Citation2004) so much so that an abnormal level of propionic acid was used to induce intestinal injury in newborn piglets as a challenge model (Di Lorenzo et al. Citation1995).
Biogenic amines
Biogenic amines can play a key role in intestinal tract homeostasis. It has been shown that luminal amines, at a low concentration and particularly under stress conditions, can stimulate the production of nucleic acids and the synthesis of proteins contributing to stabilising the cellular membranes (Wang and Johnson Citation1992; Blachier et al. Citation2007). On the other hand, a high luminal concentration of amines can lead to an over proliferation of cells which can compromise the normal mucosal function/turnover (Paulsen et al. Citation1997). In the small intestine, a large part of the amines derives from the feed and can be absorbed by the host while, in the large intestine, the concentration of amines is more ascribed to microbial activity. Different bacteria are involved and share different parts of the metabolic pathways for the production of amines (Nakamura et al. Citation2019). The results of the present meta-analysis indicated that a reduction of dietary CP could significantly reduce the level of cadaverine (p = .012; coefficient = −6.16) and putrescine (p = .030; coefficient = −4.89) in the intestinal content of post-weaning pigs, while no effect was observed for the level of spermidine and spermine (Table ). Cadaverine and putrescine are considered to be the most abundant biogenic amines in the guts of pigs (Wang et al. Citation2019; Zhang et al. Citation2016), deriving from bacterial fermentation of sources provided through the diet, mainly by those bacteria belonging to the Bacteroidetes phylum. The reduction of cadaverine and putrescine according to the level of dietary protein could be explained by a reduction of the substrate available for the microbial metabolism in the intestinal content of pigs fed a low dietary protein diet as previously reported in adult pigs and rats (Wang et al. Citation2019; Zhang et al. Citation2016; Fan et al. Citation2017; CitationMu et al. 2016, ). The level of biogenic amines in the colon may also vary in function of the type of protein source; in fact, Wang et al. (Citation2019) showed that putrescine was significantly higher in pigs fed a low protein diet having a peptide source (casein hydrolysate) as compared to a low protein diet supplied with free AAs. In general, when free AAs addition increases the quantity of intact protein is reduced and, thus, fewer undigested proteins are expected to reach the hind segments of the intestine. In addition, the Authors found that the level of cadaverine was significantly associated with an increase in the dLys/CP ratio (p = .034; coefficient = 12.01) which is reasonable since cadaverine is produced by lysine decarboxylation (Kuley et al. Citation2012).
The effect of the dietary protein level on the intestinal mucosa
In the present meta-analysis, some parameters associated with intestinal mucosal homeostasis were included. Morphological parameters of the intestine, including villous height, crypt depth and their ratio have been widely used as markers of the gut health of weaned piglets (Lallès et al. Citation2007). A higher villous height has been associated with an increase in absorptive area while deeper crypts have been associated with rapid epithelial turnover as undifferentiated cells migrate along the crypt-villus axes (Yang et al. Citation2013). A total of 11 studies reporting data for crypt depth and villus height were included in the meta-analysis; the results showed that the intestinal morphology parameters were not affected by the dietary CP content. The intestinal crypt depth was negatively affected by the level of the dLys/CP ratio (p = .016; coefficient = −2.54), suggesting that crypt depth could be influenced by the level of dLys instead of by the total dietary CP (Table ). A previous study by He et al. (Citation2013), investigating the effect of a lysine-restricted diet pointed out that dietary supplementation with dLys could increase crypt depth in the jejunum and reduce it in the ileum. In the present meta-analysis, the intestinal tract (small or large intestine) was included as a factor but it was not significant; however, it cannot be excluded that dietary dLys may play a different role according to the different parts of the intestinal tract. Furthermore, it should be considered that dLys, in the large intestine, cannot be directly utilised by the epithelial cells but is catabolised by microbes (Chen et al. Citation2009; Dai et al. Citation2010; Dai et al. Citation2012); thus, what was observed was the overall effect of the microbial protein formation and AA utilisation occurring in the guts of piglets.
Table 4. The effect of the dietary crude protein level on the intestinal mucosa of post-weaning pigs.
In addition, some data regarding the expression of the genes related to epithelial inflammation and the tight junction, including interleukins (ILs) 1, 6 and 10, Tumour Necrosis Factor-Alpha (TNF -α), Interferon gamma (IFNγ), Toll-like receptor 4 (TLR-4), claudins 1 and 3, and occluding and zonulae occludes were recorded. However, very few studies which included these data were found; thus, statistical analysis was carried out only on the level of TLR-4 for which the data were reported in 4 studies. The results showed that the intestinal (in the ileum and the colon) expression of TLR-4 was significantly reduced with the reduction of dietary CP (p < .001; coefficient = −15.88) (Table ). Like the other TLRs, TLR-4 can recognise some of the molecular patterns conserved present in large groups of microbes (Stokes et al. Citation2004); the activation of TLR-4 protein causes the stimulation of the intracellular signalling pathway NF-κB and, in turn, the production of inflammatory cytokines. Based on the results obtained for TLR-4 expression, and those for SCFAs, pH and amine concentration, it could be hypothesised that a reduction of dietary CP could reduce the microbial fermentation in the piglet large intestine, also resulting in reduced stimulation of TLR-4. Furthermore, TLR-4 was positively associated with the dLys/CP ratio (p = .048; coefficient = 12.58) (Table ). No previous information has been reported in the literature; however, a study of Han et al. (Citation2018) suggested that piglets under dietary Lys restriction could have an increase in inflammatory response while no effect on the expression of TLR-4 in the kidney, liver, and spleen was observed. As discussed previously, since the Lys in the gut is mainly utilised by microbes, it can be hypothesised that an increase in dietary Lys promoted the microbial metabolism and their proliferation resulting in an increase in TLR-4 expression. However, the results obtained were based on four studies; thus, additional data are needed to confirm the present results. Overall, the results obtained for the intestinal morphology and inflammatory markers suggested that both total dietary CP level and the dLys/CP ratio could play a key role in maintaining gut homeostasis and integrity in weaned pigs.
The effect of the dietary protein level on average daily gain and faecal score
The analysis was carried out on 15 studies for the ADG and 10 studies for the faecal score. The results of the GLM showed that a reduction in dietary CP percentage in the weaning diet did not affect the ADG of piglets (p > .1) while the increasing level of the dLys/CP ratio significantly increased the ADG (p = .024; coefficient = 23.40) (Table ). The present results for ADG in post-weaning piglets agreed with the previous meta-analysis carried out by Wang et al. (Citation2020) in adult pigs in which the authors observed no significant decrease inADG and the gain to feed ratio when the CP reduction in the diet was less than 4 pts%.
Table 5. The effect of the dietary crude protein level on growth performance and the faecal score of post-weaning pigs.
Furthermore, the increase in ADG compared with the increase in the dLys/CP ratio observed in the present meta-analysis suggested that more than the level of dietary CP itself, it was the dietary composition of the AAs which could influence piglet ADG. In fact, as supported by the studies of Ren et al. (Citation2015) and Zheng et al. (Citation2016), a diet with a reduction of CP supplied with branched-chain amino acids (BCAAs) could contribute to more efficient maintenance of the growth performance.
The faecal score was significantly reduced with the reduction of the percentage of CP (p = .002; coefficient = −1.77) and the reduction of the dLys/CP ratio (p = .006; coefficient = −6.91) (Table ). The higher faecal consistency (indicated by the lower score) with the decreasing CP level could be due to an improvement in the gut health of piglets fed diets with lower CP. Better gut health would be associated with lower intestinal inflammation due to a lower production of metabolites derived from the undigested protein microbial fermentation in the digestive tract as supported by the results and discussion in the previous sections of the present meta-analysis.
Conclusion
In conclusion, this meta-analysis showed that a reduction of dietary crude protein could reduce the intestinal fermentation of undigested dietary protein, especially in the large intestine, represented by a reduction in pH, ammonia, cadaverine, putrescine and acetic acid. The reduction of potentially toxic metabolites derived from protein fermentation contributed to lower inflammation of the intestinal mucosa and, as final outcome, to a decrease in piglet diarrhoea. Overall, this meta-analysis showed evidence that the reduction of dietary protein in the diets of post-weaning piglets could be a reliable strategy for improving and sustaining the gut health of weaned piglets and for controlling the occurrence of diarrhoea by reducing microbial fermentation in the large intestine without compromising performance. Differences in the levels of AAs among the studies should be considered as a source of variation for the parameters investigated, and additional studies are needed to investigate in-depth the potential contribution of essential/non-essential and functional AAs on gut health. Moreover, a lack of knowledge and data were evident regarding some specific parameters, namely intestinal bacteria taxa abundancy, intestinal gene expression and blood parameters; therefore, additional studies are needed to confirm and evaluate the relationships between dietary protein level and those health markers.
Supplemental Material
Download MS Excel (23.6 KB)Supplemental Material
Download MS Excel (28.9 KB)Disclosure statement
The Authors declare that there is no conflict of interest.
Data availability statement
The authors confirm that the data supporting the findings of this study are available within the article [and/or] its supplementary materials.
References
- Aguirre M, Eck A, Koenen ME, Savelkoul PHM, Budding AE, Venema K. 2016. Diet drives quick changes in the metabolic activity and composition of human gut microbiota in a validated in vitro gut model. Res Microbiol. 167(2):114–125.
- Andriamihaja M, Davila A-M, Eklou-Lawson M, Petit N, Delpal S, Allek F, Blais A, Delteil C, Tomé D, Blachier F, et al. 2010. Colon luminal content and epithelial cell morphology are markedly modified in rats fed with a high-protein diet. Am J Physiol Gastr L. 299(5):G1030–1037.
- Arena ME, Manca de Nadra MC. 2001. Biogenic amine production by Lactobacillus. J Appl Microbiol. 90(2):158–162.
- Blachier F, Mariotti F, Huneau JF, Tomé D. 2007. Effects of amino acid-derived luminal metabolites on the colonic epithelium and physiopathological consequences. Amino Acids. 33(4):547–562.
- Canh TT, Aarnink AJA, Schutte JB, Sutton A, Langhout DJ, Verstegen MWA. 1998. Dietary protein affects nitrogen excretion and ammonia emission from slurry of growing-finishing pigs. Livest Prod Sci. 56(3):181–191.
- Carmody RN, Gerber GK, Luevano JM, Gatti DM, Somes L, Svenson KL, Turnbaugh PJ. 2015. Diet dominates host genotype in shaping the murine gut microbiota. Cell Host Microbe. 17(1):72–84.
- Chen L, Li P, Wang J, Li X, Gao H, Yin Y, Hou Y, Wu G. 2009. Catabolism of nutritionally essential amino acids in developing porcine enterocytes. Amino Acids. 37(1):143–152.
- Chen X, Song P, Fan P, He T, Jacobs D, Levesque CL, Johnston LJ, Ji L, Ma N, Chen Y, et al. 2018. Moderate dietary protein restriction optimized gut microbiota and mucosal barrier in growing pig model. Front Cell Infect Microbiol. 8:246.
- Cremin JD, Jr, Fitch MD, Fleming SE. 2003. Glucose alleviates ammonia-induced inhibition of short-chain fatty acid metabolism in rat colonic epithelial cells. Am J Physiol Gastrointest Liver Physiol. 285(1):G105–G114.
- Dai ZL, Li XL, Xi PB, Zhang J, Wu G, Zhu WY. 2012. Metabolism of select amino acids in bacteria from the pig small intestine. Amino Acids. 42(5):1597–1608.
- Dai ZL, Zhang J, Wu G, Zhu WY. 2010. Utilization of amino acids by bacteria from the pig small intestine. Amino Acids. 39(5):1201–1215.
- David LA, Maurice CF, Carmody RN, Gootenberg DB, Button JE, Wolfe BE, Ling AV, Devlin AS, Varma Y, Fischbach MA, et al. 2014. Diet rapidly and reproducibly alters the human gut microbiome. Nature. 23(505(7484):559–563.
- Diether NE, Willing BP. 2019. Microbial fermentation of dietary protein: An important factor in diet–microbe–host interaction. Microorganisms. 7(1):19.
- Di Lorenzo M, Bass J, Krantis A. 1995. An intraluminal model of necrotizing enterocolitis in the developing neonatal piglet. J Pediatr Surg. 30(8):1138–1142.
- Ducatelle R, Goossens ED, Meyer F, Eeckhaut V, Antonissen G, Haesebrouck FV, Immerseel F. 2018. Biomarkers for monitoring intestinal health in poultry: Present status and future perspectives. Vet. Res. 49:1–9.
- Fan P, Liu P, Song P, Chen X, Ma X. 2017. Moderate dietary protein restriction alters the composition of gut microbiota and improves ileal barrier function in adult pig model. Sci Rep. 7:43412.
- Friend DW, Cunningham HM, Nicholson JWG. 1963. Volatile fatty acids and lactic acid in the alimentary tract of the young pig. Can. J Anim Sci. 43(1):174–181.
- Fuller R. 1977. The Importance of Lactobacilli in maintaining normal microbial balance in the crop. Br Poult Sci. 18(1):85–94.
- Gao J, Yin J, Xu K, Li T, Yin Y. 2019. What is the impact of diet on nutritional diarrhea associated with gut microbiota in weaning piglets: a System Review. Biomed Res Int. 2019:6916189.
- Giger-Reverdin S, Duvaux-Ponter C, Sauvant D, Martin O, Do Prado IN, Müller R. 2002. Intrinsic buffering capacity of feedstuffs. Anim Feed Sci Tech. 96(1-2):83–102.
- Gloaguen M, Le Floc’h N, Van Milgen J. 2013. Couverture des besoins en acides aminés chez le porcelet alimenté avec des régimes à basse teneur en protéines. Prod Anim. 26:277–288.
- Gresse R, Chaucheyras-Durand F, Fleury MA, Van de Wiele T, Forano E, Blanquet-Diot S. 2017. Gut microbiota dysbiosis in postweaning piglets: understanding the keys to health. Trends Microbiol. 25(10):851–873.
- Gresse R, Chaucheyras Durand F, Dunière L, Blanquet-Diot , Forano E. 2019. Microbiota composition and functional profiling throughout the gastrointestinal tract of commercial weaning piglets. Microorganisms. 7(9):343.
- Han H, Yin J, Wang B, Huang X, Yao J, Zheng J, Fan W, Li T, Yin Y. 2018. Effects of dietary lysine restriction on inflammatory responses in piglets. Sci Rep. 8:1–8.
- He L, Yang H, Hou Y, Li T, Fang J, Zhou X, Yin Y, Wu L, Nyachoti M, Wu G. 2013. Effects of dietary l-lysine intake on the intestinal mucosa and expression of CAT genes in weaned piglets. Amino Acids. 45(2):383–391.
- Heo JM, Opapeju FO, Pluske JR, Kim JC, Hampson DJ, Nyachoti CM. 2013. Gastrointestinal health and function in weaned pigs: A review of feeding strategies to control post-weaning diarrhoea without using in-feed antimicrobial compounds. J Anim Physiol Anim Nutr. 97(2):207–237.
- Ichikawa H, Sakata T. 1998. Stimulation of epithelial cell proliferation of isolated distal colon of rats by continuous colonic infusion of ammonia or short-chain fatty acids is nonadditive. J Nutr. 128(5):843–847.
- Kuley E, Balikci E, Özoǧul I, Gökdogan S, Özoǧul F. 2012. Stimulation of cadaverine production by foodborne pathogens in the presence of lactobacillus, lactococcus, and streptococcus spp. J Food Sci. 77(12):M650–M658.
- Lallès JP, Bosi P, Smidt H, Stokes CR. 2007. Nutritional management of gut health in pigs around weaning. Proc Nutr Soc. 66(2):260–268.
- Langille MG. 2018. Exploring linkages between taxonomic and functional profiles of the human microbiome. MSystems. 3(2):e00163-17.
- Le Floc’h N, Wessels A, Corrent E, Wu G, Bosi P. 2018. The relevance of functional amino acids to support the health of growing pigs. Anim Feed Sci Technol. 245:104–116.
- Lebeer S, Vanderleyden J, De Keersmaecker SCJ. 2008. Genes and molecules of lactobacilli supporting probiotic action. Microbiol Mol Biol Rev. 72(4):728–764.
- Leschelle X, Robert V, Delpal S, Mouillé B, Mayeur C, Martel P, Blachier F. 2002. Isolation of pig colonic crypts for cytotoxic assay of luminal compounds: Effects of hydrogen sulfide, ammonia, and deoxycholic acid. Cell Biol Toxicol. 18(3):193–203.
- Lin J. 2004. Too much short chain fatty acids cause neonatal necrotizing enterocolitis. Med Hypotheses. 62(2):291–293.
- Ma N, Tian Y, Wu Y, Ma X. 2017. Contributions of the interaction between dietary protein and gut microbiota to intestinal health. Curr Protein Pept Sci. 18(8):795–808.
- Mariat D, Firmesse O, Levenez F, Guimarăes V, Sokol H, Doré J, Corthier G, Furet J-P. 2009. The firmicutes/bacteroidetes ratio of the human microbiota changes with age. BMC Microbiol. 9:123–126.
- Minitab 18 Statistical Software 2018. [Computer software]. State College (PA): Minitab, Inc. Available from: www.minitab.com
- Mu C, Yang Y, Luo Z, Guan L, Zhu W. 2016. The colonic microbiome and epithelial transcriptome are altered in rats fed a high-protein diet compared with a normal-protein diet J. Nutri. 146(3): 474-483.
- Mulder IE, Schmidt B, Stokes CR, Lewis M, Bailey M, Aminov RI, Prosser JI, Gill BP, Pluske JR, Mayer CD, et al. 2009. Environmentally-acquired bacteria influence microbial diversity and natural innate immune responses at gut surfaces. BMC Biol. 7:79–20.
- Nakamura A, Ooga T, Matsumoto M. 2019. Intestinal luminal putrescine is produced by collective biosynthetic pathways of the commensal microbiome. Gut Microbes. 10(2):159–171.
- Kraimi N, Dawkins M, Gebhardt-Henrich SG, Velge P, Rychlik I, Volf J, Creach P, Smith A, Colles F, Leterrier C. 2019. Influence of the microbiota-gut-brain axis on behavior and welfare in farm animals: A review. Physiol Behav. 210:112658.
- Niven SJ, Beal JD, Brooks PH. 2006. The effect of controlled fermentation on the fate of synthetic lysine in liquid diets for pigs. Anim. Feed Scie Techn. 129(3-4):304–315.
- Noblet J, Valancogne A, Tran G. 2008. Ajinomoto Eurolysine sas, EvaPig®.[1.0. 1.4]. Computer program.
- Paulsen JE, Reistad R, Eliassen KA, Sjaastad ØV, Alexander J. 1997. Dietary polyamines promote the growth of azoxymethane-induced aberrant crypt foci in rat colon. Carcinogenesis. 18(10):1871–1875.
- Pedersen KS, Strunz AM. 2013. Evaluation of farmers’ diagnostic performance for detection of diarrhoea in nursery pigs using digital pictures of faecal pools. Acta Vet Scand . 55(1):72.
- Pieper R, Villodre Tudela C, Taciak M, Bindelle J, Pérez JF, Zentek J. 2016. Health relevance of intestinal protein fermentation in young pigs. Anim Health Res Rev. 17(2):137–147.
- Pluske JR, Pethick DW, Hopwood DE, Hampson DJ. 2002. Nutritional influences on some major enteric bacterial diseases of pig. Nutr Res Rev. 15(2):333–371.
- Pollock J, Hutchings MR, Hutchings KEK, Gally DL, Houdijk JGM. 2019. Changes in the ileal, but not fecal, microbiome in response to increased dietary protein level and enterotoxigenic Escherichia coli exposure in pigs. Appl Environ Microbiol. 85(19):e01252–e01219.
- R Core Team 2019. A language and environment for statistical computing. Vienna: R Foundation for statistical computing.
- Ren M, Zhang SH, Zeng XF, Liu H, Qiao SY. 2015. Branched-chain amino acids are beneficial to maintain growth performance and intestinal immune-related function in weaned piglets fed protein restricted diet. Asian Australas J Anim Sci. 28(12):1742–1750.
- Sajeev EPM, Amon B, Ammon C, Zollitsch W, Winiwarter W. 2018. Evaluating the potential of dietary crude protein manipulation in reducing ammonia emissions from cattle and pig manure: A meta-analysis. Nutr Cycl Agroecosyst. 110(1):161–175.
- Salaheen S, Kim SW, Haley BJ, Van Kessel JAS, Biswas D. 2017. Alternative growth promoters Modulate broiler gut microbiome and enhance body weight gain. Front Microbiol. 8:2088–2011.
- Sauvant D, Perez JM, Tran G. 2002. Tables de composition et de valeur nutritive des matières premières destinées aux animaux d'élevage. Porcs, volailles, bovins, ovins, caprins, lapins, chevaux, poissons. Paris: INRA Editions.
- Sauvant D, Perez JM, Tran G. 2004. Tables of composition and nutritional value of feed materials. Pigs, pultry, cattle, sheep, goats, rabbits, horses, fish. Paris: INRA Editions.
- Stokes CR, Bailey M, Haverson K, Harris C, Jones P, Inman C, Pié S, Oswald IP, Williams B. a, Akkermans ADL, et al. 2004. Postnatal development of intestinal immune system in piglets: implications for the process of weaning. Anim Res. 53(4):325–334.
- Trevisi P, Luise D, Correa F, Bosi P. 2021. Timely control of gastrointestinal eubiosis: a strategic pillar of pig health. Microorganisms. 9(2):313.
- Vince A, Dawson AM, Park N, O'Grady F. 1973. Ammonia production by intestinal bacteria. Gut. 14(3):171–177.
- Wang H, Long W, Chadwick D, Velthof GL, Oenema O, Ma W, Wang J, Qin W, Hou Y, Zhang F. 2020. Can dietary manipulations improve the productivity of pigs with lower environmental and economic cost? A global meta-analysis. Agric Ecosyst Environ. 289:106748.
- Wang H, Shen J, Pi Y, Gao K, Zhu W. 2019. Low-protein diets supplemented with casein hydrolysate favor the microbiota and enhance the mucosal humoral immunity in the colon of pigs. J Anim Sci Biotechnol. 10:1–13.
- Wang JY, Johnson LR. 1992. Luminal polyamines substitute for tissue polyamines in duodenal mucosal repair after stress in rats. Gastroenterology. 102(4 Pt 1):1109–1117.
- Wang Y, Zhou J, Wang G, Cai S, Zeng X, Qiao S. 2018. Advances in low-protein diets for swine. J Anim Scie Biotech. 9(1):60.
- Wen X, Wang L, Zheng C, Yang X, Ma X, Wu Y, Chen Z, Jiang Z. 2018. Fecal scores and microbial metabolites in weaned piglets fed different protein sources and levels. Anim Nutr. 4(1):31–36.
- Windey K, de Preter V, Verbeke K. 2012. Relevance of protein fermentation to gut health. Mol Nutr Food Res. 56(1):184–196.
- Yang Z, Liao SF. 2019. Physiological effects of dietary amino acids on gut health and functions of swine. Front Vet Sci. 6:169.
- Yang H, Xiong X, Yin Y. 2013. Development and renewal of intestinal villi in pigs. In: Nutritional and physiological functions of amino acids in pigs . Vienna: Springer, p. 29–47.
- Yu D, Zhu W, Hang S. 2019. Effects of long-term dietary protein restriction on intestinal morphology, digestive enzymes, gut hormones, and colonic microbiota in pigs. Animals. 9(4):180–114.
- Zhang C, Yu M, Yang Y, Mu C, Su Y, Zhu W. 2016. Effect of early antibiotic administration on cecal bacterial communities and their metabolic profiles in pigs fed diets with different protein levels. Anaerobe. 42:188–196.
- Zhang K, Wang N, Lu L, Ma X. 2020. Fermentation and metabolism of dietary protein by intestinal microorganisms. Curr Protein Pept Sci. 21(8):807–811.
- Zheng L, Wei H, Cheng C, Xiang Q, Pang J, Peng J. 2016. Supplementation of branched-chain amino acids to a reduced-protein diet improves growth performance in piglets: involvement of increased feed intake and direct muscle growth-promoting effect. Br J Nutr. 115(12):2236–2245.
- Zhou L, Fang L, Sun Y, Su Y, Zhu W. 2016. Effects of the dietary protein level on the microbial composition and metabolomic profile in the hindgut of the pig. Anaerobe. 38:61–69.