Abstract
This study investigated the influence of dietary supplementation of rumen-unprotected betaine (RUPB) and rumen-protected betaine (RPB) on growth performance, meat quality and fatty acid profile in intramuscular and subcutaneous fat of growing lambs. A total of 24 Hu male lambs were equally divided into three groups and fed a basal diet or basal diet supplemented with 1.6 g/kg of RUPB or 2.9 g/kg of RPB for 68 days. The water loss, redness of longissimus dorsi (LD) muscle and abdominal fat percentage were reduced in RUPB and RPB groups (p < .05). RPB supplementation decreased the shear force of LD muscle (p < .05). Polyunsaturated fatty acid (PUFA) content in LD muscle was decreased in RPB group, whereas n-3PUFA content was increased in RUPB group (p < .05). RUPB and RPB supplementation reduced the content of saturated fatty acid (SFA), and increased the contents of PUFA, monounsaturated fatty acid (MUFA), and n-6PUFA in subcutaneous fat (p < .05). Furthermore, dietary RUPB and RPB supplementation increased the mRNA expression of acetyl-CoA carboxylase (ACC) and glycerol-3-phosphate acyltransferase 1 (GPAT1) in LD muscle (p<.05); it increased the mRNA expression of fatty acid synthetase (FANS) and lipoprotein lipase (LPL) but reduced the mRNA expression of GPAT1 in subcutaneous fat (p < .05). In conclusion, either RUPB or RPB supplementation may improve the meat quality and slightly change the fat deposition and fatty acid composition of intramuscular and subcutaneous fat, and RUPB is recommended as a supplement for growing male lambs based on its lower cost.
Dietary supplementation of both rumen-unprotected betaine and (RUPB) and rumen-protected betaine (RPB) improved meat quality and altered the fat deposition of growing male lambs.
Both RUPB and RPB supplementation changed fatty acid composition in the longissimus dorsi muscle and subcutaneous adipose tissue.
RUPB is recommended as a supplement for growing lambs based on its lower cost.
Highlights
Introduction
Lamb products contain higher fat levels than beef and pork, and lower contents of polyunsaturated fatty acids (PUFAs) compared with pork, which is a major threat to consumer satisfaction and demand for lambs (Enser et al. Citation1996). Therefore, nutritional strategies to reduce fat content and enrich the fat of meat with more PUFA are warranted for lamb production. Betaine, a naturally occurring and stable compound derived from sugar beets, is involved in lipid transport and metabolism (Eklund et al. Citation2005; Deminice et al. Citation2015), methyl group donation (Figueroa-Soto and Valenzuela-Soto Citation2018) and regulation of cellular osmotic pressure (Niculescu and Zeisel Citation2002). In animals, betaine is either produced by choline oxidation endogenously or obtained from the diet through wheat bran and condensed molasses soluble or feed-grade betaine additives (Eklund et al. Citation2005). In recent decades, dietary betaine has been observed to regulate lipid metabolism, reduce fat accumulation and alter fat distribution in the body of both monogastric animals and ruminants (Fernández et al. Citation1998; Matthews et al. Citation2001; Bock et al. Citation2004; Chen et al. Citation2019). Therefore, it is widely considered as a ‘carcase modifier’ in animal nutrition.
Several studies in ruminants have focussed on the degradation of betaine by rumen microbes; however, the findings are inconsistent. For example, Loest et al. (Citation2001) found that >60% of feed-grade betaine remained after 24 h in vitro with a forage-based diet as the fermentation substrate. However, a rapid disappearance of betaine was observed in an experiment involving the rumen simulation technique (RUSITEC) when the forage-to-concentrate ratio of the fermentation substrate was 5:5 (Mahmood et al. Citation2020). Therefore, over the decades, two forms of betaine have been used in ruminant feeding: rumen-unprotected betaine (RUPB) and rumen-protected betaine (RPB). Feeding RUPB has been demonstrated to increase milk yield and fat content in cows and goats (Fernandez et al. Citation2004; Wang et al. Citation2010; Peterson et al. Citation2012). However, no alteration in milk yield and components was observed when RPB was fed to lactating dairy cows (Davidson et al. Citation2008). For meat-producing ruminants, diets supplemented with RUPB did not influence the weight gain; however, it decreased the thickness of subcutaneous fat in growing lambs (Fernández et al. Citation1998; Fernandez et al. Citation2000). Another study reported that supplementing RUPB increased both weight gain and subcutaneous fat thickness in finishing steers (Bock et al. Citation2004). Therefore, studies on the effects of dietary betaine on growth performance and carcase fat composition in ruminants seem to be inconsistent. Moreover, to date, no study has examined differences in the effectiveness of RUPB and RPB supplementation in ruminant feeding. To analyse these differences, we compared the effects of RUPB and RPB on the growth performance, meat quality, and fatty acid profile in intramuscular and subcutaneous fat of growing lambs in this study.
Materials and methods
Animals, diets, and experimental design
A total of 24 male Hu lambs (5 months old; 21.74 ± 2.54 kg) were divided into three groups in a complete randomised design (CRD). Animals were kept in individual stalls equipped with feeders and free access to tap water. Eight lambs in each group were randomly assigned to one of the following three experimental diets: a basal diet (control group), a basal diet supplemented with 1.6 g/kg of betaine anhydrous (RUPB group) and a basal diet supplemented with 2.9 g/kg of RPB (RPB group). The basal diet was formulated to meet the National Research Council requirements (NRC Citation2007) for growing lambs. The diet components and nutrient levels are presented in Table . The net content of betaine anhydrous in the RPB was 55%, thus, the actual addition amounts of betaine anhydrous in RUPB and RPB groups were identical. Doses of RUPB and RPB were chosen according to prior dose-response trials in lambs (Dong et al. Citation2020). The RUPB and RPB (which contained 55% betaine anhydrous protected by hydroxypropyl methylcellulose) were provided by the Tianshi Feed Company, Yixing, Jiangsu Province, China. The rumen protection rate of RPB measured with an in situ protocol was 60% (Dong et al. Citation2020).
Table 1. Composition and nutrient levels of the diets (dry matter basis).
The experimental period lasted 68 days, with the first 7 days for adaptation. The body weight (BW) of each lamb on the first day of feeding trial was regarded as the initial BW. All lambs were weighed at 15-day intervals just before feeding to calculate the average daily gain (ADG). During the whole experiment, the lambs were fed ad libitum twice each day at 8 am and 4 pm. At the daily feeding, RUPB and RPB were pre-mixed into a small part of the ration, and the pre-mixed ration was first consumed by the lambs to ensure that the full dose was consumed. Furthermore, the individual orts were collected and weighed before each feeding to calculate the average daily feed intake (ADFI).
Sampling
On the 60th day of the experiment, blood samples were collected via the jugular vein before morning feeding and centrifuged at 3000 × g for 10 min at room temperature. The resulting serum samples were then stored at −80 °C for biochemical index analysis. The lambs were then fasted for 24 h but had free access to water until approximately 2 h before slaughter. The shrunk BW (SBW) of lambs was measured after fasting. Subsequently, the lambs were stunned by electric shocks before being slaughtered by exsanguination. The hot carcase weight was recorded immediately and dressing percentage was calculated as the percentage of hot carcase weight to SBW. Furthermore, the longissimus dorsi (LD) muscle area and subcutaneous fat thickness between the 12th and 13th ribs were measured as previously described (Kirton et al. Citation1984). The weight of abdominal, perirenal, and tail fats was recorded to calculate the fat index as follows: fat index (%) = [fat weight (g)/SBW (g)] × 100. Subsequently, adipose tissue samples were collected from subcutaneous fat between the 10th and 13 th ribs. Simultaneously, a small piece of muscle sample was collected from the left LD muscle between the 10thand 13 th ribs. The collected tissues were immediately snap-frozen in liquid nitrogen and stored at −80 °C until further use. In addition, the right half of the LD muscle was cooled at 4 °C for 24 h before analysing the meat quality.
Serum biochemical index analysis
Serum biochemical indices, including albumin, urea, total protein, cholesterol, triglyceride, low-density lipoprotein, high-density lipoprotein and glucose, were detected using commercial assay kits purchasing from Sinnowa Medical Science and Technology Co. Ltd (Nanjing, China). Other indices, including free fatty acid, growth hormone, cortisol, resistin, leptin, insulin, adiponectin, were detected using commercial assay kits purchasing from Shanghai Yuduo Biological Product Co. Ltd (Shanghai, China). All indices were determined referring to the manufacturer’s instructions using automatic biochemical analyser (DH-364, Sinnowa Medical Science and Technology Co. Ltd., Nanjing, China).
Meat quality, IMF content and fatty acids analysis
The final pH (pH 24 h) of the LD muscle was measured using a pH metre (pH-STAT, SFK-Technology, Copenhagen, Denmark) after it was chilled for 24 h. The meat colour descriptors including lightness (L*), redness (a*) and yellowness (b*) were determined using the Minolta CR-310 spectrophotometer (Konica Minolta Inc., Tokyo, Japan). Subsequently, a shear test instrument (Bulader C-LM3B, Beijing, China) was used to measure the shear force. Water losses from muscle were determined using a drip loss analyser (RH-1000, Guangzhou RunHu Instruments Co. Ltd., Guangzhou, China) within 12 h of slaughter. The measurements for pH 24 h, meat colour, shear force and water losses of the LD muscle were taken three times for each sample. The IMF content of the LD muscle was measured via Soxhlet petroleum ether extraction, referring to the method described by Zhao et al. (Citation2017). The fatty acid profile in intramuscular and subcutaneous fat was analysed using an Agilent gas chromatography system (7890A-7697A; Agilent Technologies, Santa Clara, CA) equipped with an HP-88 capillary column (20 m × 0.25 mm × 0.2 μm), referring to a method described previously (Dong et al. Citation2020). All samples were measured in triplicates.
RNA extraction and gene expression analysis
Total RNA was extracted from muscle tissues using TRIzol (Invitrogen, Shanghai, China) according to the manufacturer’s protocol. The fat tissue samples were pre-treated before RNA isolation as follows: Briefly, fat samples of 150 mg were weighed and transferred into 1 mL of pre-cooled TRIzol solution for homogenisation (24,000 × g, 3 min). The homogenised mixture was incubated for 30 min at −20 °C and centrifuged at 12,000 × g at 4 °C for 10 min. Subsequently, the bottom-phase solution was carefully transferred into a new tube avoiding the fat layer and then centrifuged again according to the previous centrifugation conditions. The lower layer was transferred into a new tube again and left for 5 min at room temperature, and total RNA was extracted using the TRIzol method. The quantity and quality of total RNA were evaluated using the Agilent 2100 Bioanalyzer system (Agilent Technologies, Palo Alto, CA) and agarose gel electrophoresis. Subsequently, the total RNA was reverse transcribed to complementary DNA (cDNA) using a Quantscript Reverse Transcriptase Kit (KR103, Tiangen, Biotech Co., Ltd., Beijing, China) according to the manufacturer’s protocol. Primers designed for housekeeping genes and target genes are listed in Table . Primers efficiencies were assessed using a serial 10-fold dilution of pooled cDNA and calculated using the formula E (%) = [−1 + 10(−1/slope)] × 100, with the slope of the linear curve of cycle threshold (Ct) values plotted against the log dilution. RefFinder (http://blooge.cn/RefFinder/), including Normfinder, geNorm, BestKeeper and the comparative ΔCT method, was used to compare and rank the candidate reference genes (ACTB, GAPDH, and RPL13). Based on the rankings from each program, an appropriate weight to individual gene was assigned and the geometric mean of their weights was calculated for the overall final ranking. A higher expression stability was indicated by a lower gene geomean of ranking value. Eventually, the most stable reference gene was used for gene expression normalisation. For quantitative real-time polymerase chain reaction (RT-qPCR) analysis, the generated cDNA was amplified using the SuperReal PreMix Plus (SYBR Green) (FP205, Tiangen, Biotech Co., Ltd., Beijing, China). The reaction was first performed in a 20-μL reaction solution containing 10 μL of 2× SuperReal PreMix Plus, 1.6 μL of primer, 1 μL of cDNA, 0.4 μL of 50× ROX, and 7.0 μL of RNase-free water. The reaction of a negative control without the cDNA sample was performed. The RT-qPCR was performed using an ABI 7500 Real-Time PCR System. The cycling conditions were as follows: 95 °C for 15 min, followed by 45 cycles of amplification at 95 °C for 10 s and 60 °C for 32 s. All samples were measured in triplicates. Gene expression was calculated as fold change using the 2−△△Ct method (Livak and Schmittgen Citation2001).
Table 2. Primer sequences for real time-qPCR.
Statistical analysis
The SPSS software (IBM Statistics, version 22, SPSS, Chicago, IL) was used for statistical analysis. The data were tested for normal distribution using the Shapiro–Wilk test. One-way analysis of variance (ANOVA) followed by the Duncan's multiple comparisons test was used for data normally distributed and a non-parametric test (Kruskal–Wallis) was used for the data not normally distributed. The threshold for statistically significant differences was set at p < .05. The results were expressed as the mean and pooled standard error of the mean (SEM).
Results
Growth performance, slaughter traits and serum biochemical indices
As shown in Table , no difference was found in any parameters of growth performances and slaughter traits among the three groups (p > .05). The serum biochemical indices were also not affected by dietary supplementation of RUPB and RPB (p > .05; Table ).
Table 3. Effects of RUPB and RPB on growth and slaughter performance of lambs.
Table 4. Effects of RUPB and RPB on serum biochemical indices of lambs.
Meat quality
Table shows the meat quality characteristics of the LD muscle in male lambs fed diets containing RUPB and RPB. There was no effect of dietary RUPB and RPB supplementation on pH24 h (p > .05). Water loss was decreased in the RUPB and RPB groups as compared with the control group (p < .05). The shear force was decreased in the RPB group (p < .05), and no difference was observed in shear force between the control and RUPB groups (p > .05). Dietary RUPB and RPB supplementation led to increased redness (a*) of the LD muscle (p < .05) but did not affect its lightness (L*) and yellowness (b*) (p > .05).
Table 5. Effects of RUPB and RPB on the meat quality of lambs.
Fat deposition traits
Table displays the fat deposition traits of male lambs fed diets containing RUPB and RPB. Supplementation with either RUPB or RPB did not affect the thickness of subcutaneous fat and the weight of abdominal, perirenal and tail fats (p > .05). Dietary RUPB and RPB supplementation significantly reduced the abdominal fat index (p < .05) but did not affect the perirenal and tail fat indices (p > .05). No significant difference was observed in the IMF content of the LD muscle among the three groups (p > .05).
Table 6. Effects of RUPB and RPB on fat deposition of lambs.
Fatty acid profiles in the LD muscle and subcutaneous adipose tissue
Table shows the fatty acid profiles in the LD muscle of male lambs fed diets containing RUPB and RPB. Dietary RUPB and RPB supplementation did not influence the contents of SFA, unsaturated fatty acid (UFA), monounsaturated fatty acid (MUFA) and n-6PUFA and the n-6/n-3PUFA ratio (p > .05). However, lambs in the RPB group exhibited a lower content of PUFA than those in the control and RUPB groups (p < .05). The content of n-3 PUFA and the ratio of PUFA to SFA in the LD muscle were higher in RUPB-fed lambs than in RPB-fed lambs (p < .05). Concerning individual fatty acids, dietary RUPB supplementation decreased the contents of palmitic acid (C16:0) and palmitoleate (C16:1N7) (p < .05), but increased the content of heptadecanoic acid (C17:0) (p < .05) in the LD muscle of lambs. The content of eicosadienoic acid (C20:2) in the LD muscle was lower in the RPB than in the control group (p < .05).
Table 7. Effects of RUPB and RPB on fatty acid composition of LD muscle in lambs.
Table shows the fatty acid profiles in the subcutaneous adipose tissue of male lambs fed diets containing RUPB and RPB. The content of palmitic acid (C16:0) in the subcutaneous fat of lambs was lower (p < .05) in the RUPB and RPB groups than in the control group. The content of oleic acid (C18:1N9C) was higher in the RUPB group than in the control group (p < .05), and that of docosadienoic acid (C22:2N6) was higher in the RUPB group than in the other two groups (p < .05). RUPB and RPB supplementation decreased the content of SFA (p < .05), but increased the contents of MUFA and n-6PUFA and the ratio of PUFA to SFA in the subcutaneous fat of lambs (p < .05). The content of PUFA was increased in the RUPB group than in the control group (p < .05).
Table 8. Effects of RUPB and RPB on fatty acid composition of subcutaneous adipose tissue in lambs.
mRNA expression of genes involved in lipid metabolism in the LD muscle and subcutaneous adipose tissue
As demonstrated in Figure , the mRNA expression of the acetyl-coenzyme A carboxylase (ACC) in the LD muscle was increased in the RUPB and RPB groups (p < .05). The relative expression of glycerol-3-phosphate acyltransferase 1 (GPAT1) in the LD muscle was higher in the RPB group than in the control group (p < .05). However, the gene expression of fatty acid synthetase (FANS) and lipoprotein lipase (LPL) in the LD muscle did not significantly differ among the groups (p > .05).
Figure 1. The relative abundance of mRNA for genes involved in lipid metabolism of LD muscle in lambs. Control, basal diets; RUPB, treatment with 1.6 g/kg dietary rumen-unprotected betaine supplementation; RPB, treatment with 2.9 g/kg dietary rumen-protected betaine supplementation. ACC: acetyl-CoA carboxylase; FANS: fatty acid synthetase; LPL: lipoprotein lipase; GPAT1: glycerol-3-phosphate acyltransferase 1.
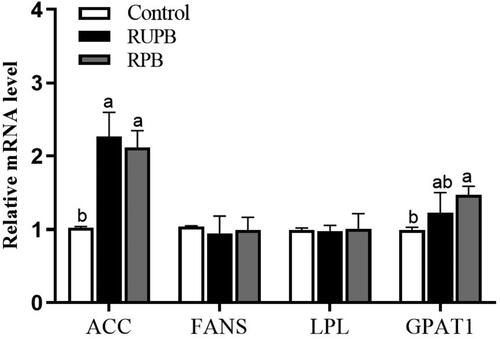
Figure demonstrates the relative expression of genes involved in lipid metabolism in the subcutaneous adipose tissue. RUPB and RPB supplementation increased the gene expression of FANS and decreased the gene expression of GPAT1 in the subcutaneous adipose tissue (p < .05). The expression of ACC was significantly lower in the RUPB group than in the other two groups (p < .05). Dietary RUPB and RPB supplementation increased the relative expression of LPL in the subcutaneous adipose tissue (p < .05), and this effect was more pronounced in the RPB group than in the RUPB group (p < .05).
Figure 2. The relative abundance of mRNA for genes involved in lipid metabolism of subcutaneous adipose tissue in lambs. Control, basal diets; RUPB, treatment with 1.6 g/kg dietary rumen-unprotected betaine supplementation; RPB, treatment with 2.9 g/kg dietary rumen-protected betaine supplementation. ACC: acetyl-CoA carboxylase; FANS: fatty acid synthetase; LPL: lipoprotein lipase; GPAT1: glycerol-3-phosphate acyltransferase 1.
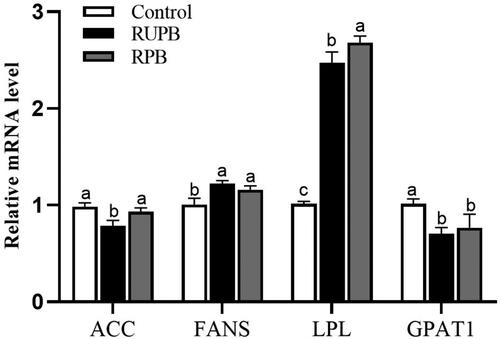
Discussion
Consistent with previous findings, the results of our study demonstrated that diets supplemented with RUPB and RPB did not cause noticeable changes in growth performance, including the ADG, ADFI and feed efficiency of lambs (Fernández et al. Citation1998; Nezamidoust et al. Citation2014; Dong et al. Citation2020). This result was likely observed because the total energy intake of male lambs was equivalent among groups, and dietary betaine may not have altered the energy expenditure of male lambs in this study, though betaine is previously reported to reduce the energy requirements for maintenance and thus increase energy availability for growth in heat-stressed ruminants (DiGiacomo et al. Citation2014; DiGiacomo et al. Citation2016; Lakhani et al. Citation2020). This discrepancy presumably arises because animals of present study reared under thermoneutral conditions did not respond to betaine supplementation to a greater extent than those reared under heat-stressed conditions. Fernandez et al. (Citation2000) found a significant difference in daily gain between female lambs fed RUPB and RPB; however, no difference in daily gain was observed in this study. Differences in the gender of lambs could potentially explain the variable response to betaine supplementation between the two studies. Similar to growth performance, carcase traits including dressing percentage and LD muscle area were also not impacted by RUPB and RPB supplementation. These findings are consistent with previous findings of studies on lambs (Fernandez et al. Citation2000; Dong et al. Citation2020) and feedlot cattle (Loest et al. Citation2002; DiGiacomo et al. Citation2014).
The water content of muscle, which is highly related to the juiciness of the meat, is up to 75% (Pedersen et al. Citation2003). Therefore, the water-holding capacity of muscles is the most important qualitative characteristic of meat quality. Water loss is a direct indicator of water-holding capacity of the meat. Therefore, reducing water loss in meat products is highly desirable. The present study revealed that dietary supplementation of both RUPB and RPB significantly reduced water loss in the LD muscle. Therefore, both RUPB and RPB may be used as feed additives in lamb production for enhancing the water-holding capacity of muscles. Similar effects of betaine on water-holding capacity of muscles in lambs were also observed by Dong et al. (Citation2020).
The accumulation of lactic acid and the dissolution of carbon dioxide in muscle have been reported to decrease the pH of the meat during storage (Hamoen et al. Citation2013; Lee et al. Citation2017). The ultimate pH of muscle affects meat quality because it can alter water-holding capacity, colour and tenderness (Hamoen et al. Citation2013). In this study, we did not observe evident changes in the pH of the LD muscle in lambs fed RUPB- and RPB-containing diets, which is consistent with previous findings in feedlot cattle (DiGiacomo et al. Citation2014). However, an increase in the pH24 h of meat in lambs after dietary RUPB supplementation was reported by Dong et al. (Citation2020). Inconsistent findings in our study and the study by Dong et al. may result from factors such as different growth stages of lambs, environmental temperature and handling before and at slaughter.
In keeping with findings from Dong et al. (Citation2020), RPB supplementation also weakened the shear force of the LD muscle in the present study, which suggests that RPB can be used as a feed additive for improving the tenderness of meat in lambs. The tenderness of the meat is inversely related to the collagen levels of intramuscular connective tissues (Purslow Citation2014). Furthermore, the accumulation of IMF can remould the intramuscular connective tissues, reducing the cross-linking of collagen and further enhancing the tenderness of meat (Nishimura et al. Citation1999). In this study, the content of IMF in the LD muscle increased by 42.3% with RPB supplementation, which suggests that the reduction of shear force in the RPB-supplemented group might be a result of the increased IMF content of the meat.
The meat colour of fresh mutton after oxygenation plays an important role in a customer’s willingness to purchase the meat (Calnan et al. Citation2016). It has been reported that the meat colour parameters of lambs (L*, a* and b*) are affected by muscle traits, such as pH24 h and the concentrations of myoglobin, iron and zinc (Calnan et al. Citation2016). In addition, the meat colour is known to be strongly correlated with oxidant capacity. Therefore, natural antioxidants, such as vitamin E or some plant extracts, have been used extensively for improving colour stability in meat production (Karre et al. Citation2013). Previous studies have reported that supplementation of vitamin E or the extracts of grape seed, pine bark and plum puree may potentially increase the redness of beef (Yildiz-Turp and Serdaroglu Citation2010; Suman et al. Citation2014). Therefore, in this study, the increased redness value of the LD muscle may be attributed to the antioxidant protection of RUPB and RPB on lambs. However, consumer acceptability of increased redness of lamb meat should be examined.
Although fat is considered to be an important raw material in food and cosmetic industries (Peng et al. Citation2016), excess fat deposition in adipose tissue, specifically in the abdominal fat, should be avoided in lamb production. Various dietary strategies to reduce fat deposition and increase IMF content have been proposed to improve the meat quality of lambs. In meat production, betaine is often used as a ‘carcase modifier’ to redistribute fat deposition and improve lean percentage owing to its involvement in lipid metabolism. In lambs, diets supplemented with 2 g/kg betaine reduced the subcutaneous fat thickness (Fernández et al. Citation1998). A further study demonstrated that betaine anhydrous reduced the fat thickness, perirenal fat and intramuscular content of lipids in female lambs, while no effect of rumen escape betaine or betaine anhydrous was found on fat deposition in male lambs (Fernandez et al. Citation2000). The backfat thickness and visceral fat of steers were also not altered by dietary betaine (Loest et al. Citation2002; DiGiacomo et al. Citation2014). Similarly, in this study, we did not observe changes in fat deposition in the tail, perirenal areas and subcutaneous tissue of male lambs after RUPB and RPB supplementation. However, the percentage of abdominal fat was decreased in lambs fed with RUPB and RPB-supplemented diets, which suggests that both RUPB and RPB supplementation slightly reduced abdominal fat deposition, with RUPB exerting more potent effects.
In ruminants, fatty acid deposition in muscle and adipose tissue results from diet, post-absorption metabolism, endogenous synthesis and fatty acid transport (Lanza et al. Citation2015). In this study, the dietary composition of lambs in different groups was the same, except for the presence of either RUPB or RPB. Therefore, changes observed in the composition of fatty acids in the present study may be associated with post-absorption metabolism, endogenous synthesis and fatty acid transport. It has been reported that the fatty acid profile of muscles is closely associated with meat quality traits, such as meat hardness, flavour and shelf life (Wood et al. Citation2004). Furthermore, high levels of UFAs in the mutton are known to reduce the melting point and shelf life and improve the flavour of the meat (Mottram Citation1998). In this study, we found a reduced content of PUFA in the LD muscle in the RPB group, which might extend shelf life of the meat. This finding is consistent with that of the previous study, which reported that the diet containing betaine and distillers’ dried grains with solubles could decrease the percentage of PUFA in the muscle of finishing pigs (Wang et al. Citation2015). In addition, this study revealed that the percentage of long-chain n-3PUFA, which is known to be beneficial for human health (Bessa et al. Citation2007), in the LD muscle was higher in the RUPB group than in the other two groups. This result is in agreement with what was reported by El-Bahr et al. (Citation2021). As expected, RUPB and RPB supplementation increased the contents of MUFA and PUFA in the subcutaneous adipose tissue and reduced the SFA content. This reveals that RUPB and RPB affect the fatty acid profile of adipose tissue to a much greater extent than that of muscle. We also analysed the mRNA levels of genes involved in fatty acid metabolism in the LD muscle and subcutaneous adipose tissue. Of these genes, ACC and FANS were found to be involved in de novo fatty acid synthesis, LPL was responsible for fatty acid uptake and GPAT1 was closely associated with fatty acid esterification (Li et al. Citation2015). The data of gene expression in the present study suggested that the feeding strategies used in this study had different effects on fatty acid metabolism of the LD muscle and subcutaneous adipose tissue, which is potentially associated with differences in lipid metabolism of muscle and adipose tissues. This may partially explain why the effects of betaine supplementation on the fatty acid profile of the subcutaneous adipose tissue were more profound than that of the LD muscle.
Conclusion
In conclusion, both RUPB and RPB supplementation may improve meat quality in growing lambs by changing the fat deposition and fatty acid composition of the LD muscle and subcutaneous adipose tissue in carcase; however, no effects were observed on the carcase traits and growth performance of lambs. Considering the cost, we recommend RUPB as a supplement for growing lambs.
Ethical statement
The study was performed at the experimental ranch of Yangzhou University (China), and all animal management and sampling procedures were approved by the Institutional Animal Care and Use Committee (IACUC) of the Yangzhou University Animal Experiments Ethics Committee (permit number: SYXK [Su] IACUC 2012-0029).
Disclosure statement
The authors declare no conflicts of interest.
Data availability statement
The data that support the findings of this study are available from the corresponding author upon reasonable request.
Correction Statement
This article has been republished with minor changes. These changes do not impact the academic content of the article.
Additional information
Funding
References
- Bessa RJB, Alves SP, Jeronimo E, Alfaia CM, Prates JAM, Santos-Silva J. 2007. Effect of lipid supplements on ruminal biohydrogenation intermediates and muscle fatty acids in lambs. Eur J Lipid Sci Technol. 109(8):868–878.
- Bock B, Brethour J, Harmoney K, Goodall S. 2004. Influence of betaine on pasture, finishing, and carcass performance in steers. Professional Anim Scientist. 20(1):53–57.
- Calnan H, Jacob RH, Pethick DW, Gardner GE. 2016. Production factors influence fresh lamb longissimus colour more than muscle traits such as myoglobin concentration and pH. Meat Sci. 119:41–50.
- Chen R, Wen C, Cheng Y, Chen Y, Zhuang S, Zhou Y. 2019. Effects of dietary supplementation with betaine on muscle growth, muscle amino acid contents and meat quality in cherry valley ducks. J Anim Physiol Anim Nutr (Berl). 103(4):1050–1059.
- Davidson S, Hopkins BA, Odle J, Brownie C, Fellner V, Whitlow LW. 2008. Supplementing limited methionine diets with rumen-protected methionine, betaine, and choline in early lactation holstein cows. J Dairy Sci. 91(4):1552–1559.
- Deminice R, da Silva RP, Lamarre SG, Kelly KB, Jacobs RL, Brosnan ME, Brosnan JT. 2015. Betaine supplementation prevents fatty liver induced by a high-fat diet: effects on one-carbon metabolism. Amino Acids. 47(4):839–846.
- DiGiacomo K, Simpson S, Leury BJ, Dunshea FR. 2016. Dietary betaine impacts the physiological responses to moderate heat conditions in a dose dependent manner in sheep. Animals-Basel. 6(9):51.
- DiGiacomo K, Warner RD, Leury BJ, Gaughan JB, Dunshea FR. 2014. Dietary betaine supplementation has energy-sparing effects in feedlot cattle during summer, particularly in those without access to shade. Anim Prod Sci. 54(4): 450–458.
- Dong L, Jin YQ, Cui HH, Yu LH, Luo Y, Wang SN, Wang HR. 2020. Effects of diet supplementation with rumen-protected betaine on carcass characteristics and fat deposition in growing lambs. Meat Sci. 166:108154.
- Dong L, Zhong ZX, Cui HH, Wang SN, Luo Y, Yu LH, Loor JJ, Wang HR. 2020. Effects of rumen-protected betaine supplementation on meat quality and the composition of fatty and amino acids in growing lambs. Animal. 14(2):435–444.
- Eklund M, Bauer E, Wamatu J, Mosenthin R. 2005. Potential nutritional and physiological functions of betaine in livestock. Nutr Res Rev. 18(1):31–48.
- El-Bahr SM, Shousha S, Khattab W, Shehab A, El-Garhy O, El-Garhy H, Mohamed S, Ahmed-Farid O, Hamad A, Sabike I. 2021. Impact of dietary betaine and metabolizable energy levels on profiles of proteins and lipids, bioenergetics, peroxidation and quality of meat in Japanese quail. Animals – Basel. 11(1):117.
- Enser M, Hallett K, Hewitt B, Fursey GA, Wood JD. 1996. Fatty acid content and composition of English beef, lamb and pork at retail. Meat Sci. 42(4):443–456.
- Fernández C, Gallego L, Lopez-Bote C. 1998. Effect of betaine on fat content in growing lambs. Anim Feed Sci Technol. 73(3–4):329–338.
- Fernandez C, Lopez-Saez A, Gallego L, de la Fuente JM. 2000. Effect of source of betaine on growth performance and carcass traits in lambs. Anim Feed Sci Technol. 86(1–2):71–82.
- Fernandez C, Sanchez-Seiquer P, Sanchez A, Contreras A, de la Fuente JM. 2004. Influence of betaine on milk yield and composition in primiparous lactating dairy goats. Small Ruminant Res. 52(1–2):37–43.
- Figueroa-Soto CG, Valenzuela-Soto EM. 2018. Glycine betaine rather than acting only as an osmolyte also plays a role as regulator in cellular metabolism. Biochimie. 147:89–97.
- Hamoen JR, Vollebregt HM, van der Sman RG. 2013. Prediction of the time evolution of ph in meat. Food Chem. 141(3):2363–2372.
- Karre L, Lopez K, Getty KJK. 2013. Natural antioxidants in meat and poultry products. Meat Sci. 94(2):220–227.
- Kirton AH, Woods EG, Duganzich D. 1984. Predicting the fatness of lamb carcasses from carcass wall thickness measured by ruler or by a total depth indicator (tdi) probe. Livest Prod Sci. 11(2):185–194.
- Lakhani P, Kumar P, Alhussien MN, Lakhani N, Grewal S, Vats A. 2020. Effect of betaine supplementation on growth performance, nutrient intake and expression of igf-1 in karan fries heifers during thermal stress. Theriogenology. 142:433–440.
- Lanza M, Scerra M, Bognanno M, Buccioni A, Cilione C, Biondi L, Priolo A, Luciano G. 2015. Fatty acid metabolism in lambs fed citrus pulp. J Anim Sci. 93(6):3179–3188.
- Lee SJ, Lee SY, Kim GD, Kim GB, Jin SK, Hur SJ. 2017. Effects of self-carbon dioxide-generation material for active packaging on pH, water-holding capacity, meat color, lipid oxidation and microbial growth in beef during cold storage. J Sci Food Agric. 97(11):3642–3648.
- Li H, Wang H, Yu L, Wang M, Liu S, Sun L, Chen Q. 2015. Effects of supplementation of rumen-protected choline on growth performance, meat quality and gene expression in longissimus dorsi muscle of lambs. Arch Anim Nutr. 69(5):340–350.
- Livak KJ, Schmittgen TD. 2001. Analysis of relative gene expression data using real-time quantitative pcr and the 2(-Delta Delta C(T)) Method . Methods. 25(4):402–408.
- Loest CA, Titgemeyer EC, Drouillard JS, Blasi DA, Bindel DJ. 2001. Soybean hulls as a primary ingredient in forage-free diets for limit-fed growing cattle. J Anim Sci. 79(3):766–774.
- Loest CA, Titgemeyer EC, Drouillard JS, Coetzer CM, Hunter RD, Bindel DJ, Lambert BD. 2002. Supplemental betaine and peroxide-treated feather meal for finishing cattle. J Anim Sci. 80(9):2234–2240.
- Mahmood M, Petri RM, Gavrău A, Zebeli Q, Khiaosa‐Ard R. 2020. Betaine addition as a potent ruminal fermentation modulator under hyperthermal and hyperosmotic conditions in vitro. J Sci Food Agric. 100(5):2261–2271.
- Matthews JO, Southern LL, Higbie AD, Persica MA, Bidner TD. 2001. Effects of betaine on growth, carcass characteristics, pork quality, and plasma metabolites of finishing pigs. J Anim Sci. 79(3):722–728.
- Mottram DS. 1998. Flavour formation in meat and meat products: a review. Food Chem. 62(4):415–424.
- National Research Council. 2007. Nutrient requirements of small ruminants: Sheep, goats, cervids, and new world camelids, first ed. Washinton, DC: National Academy Press.
- Nezamidoust M, Alikhani M, Ghorbani GR, Edriss MA. 2014. Responses to betaine and inorganic sulphur of sheep in growth performance and fibre growth. J Anim Physiol Anim Nutr. 98(6):1031–1038.
- Niculescu MD, Zeisel SH. 2002. Diet, methyl donors and DNA methylation: interactions between dietary folate, methionine and choline. J Nutr. 132(8 Suppl):2333S–2335S.
- Nishimura T, Hattori A, Takahashi K. 1999. Structural changes in intramuscular connective tissue during the fattening of Japanese black cattle: effect of marbling on beef tenderization. J Anim Sci. 77(1):93–104.
- Pedersen DK, Morel S, Andersen HJ, Balling Engelsen S. 2003. Early prediction of water-holding capacity in meat by multivariate vibrational spectroscopy. Meat Sci. 65(1):581–592.
- Peng Y, Wang J, Lin J, Liu J. 2016. Effect of dietary soybean oil and antioxidants on fatty acids and volatile compounds of tail subcutaneous and perirenal fat tissues in fattening lambs. J Anim Sci Biotechnol. 7:24.
- Peterson SE, Rezamand P, Williams JE, Price W, Chahine M, McGuire MA. 2012. Effects of dietary betaine on milk yield and milk composition of mid-lactation Holstein dairy cows. J Dairy Sci. 95(11):6557–6562.
- Purslow PP. 2014. New developments on the role of intramuscular connective tissue in meat toughness. Annu Rev Food Sci Technol. 5:133–153.
- Suman SP, Hunt MC, Nair MN, Rentfrow G. 2014. Improving beef color stability: practical strategies and underlying mechanisms. Meat Sci. 98(3):490–504.
- Wang C, Liu Q, Yang WZ, Wu J, Zhang WW, Zhang P, Dong KH, Huang YX. 2010. Effects of betaine supplementation on rumen fermentation, lactation performance, feed digestibilities and plasma characteristics in dairy cows. J Agric Sci. 148(4):487–495.
- Wang LS, Shi Z, Gao R, Su BC, Wang H, Shi BM, Shan AS. 2015. Effects of conjugated linoleic acid or betaine on the growth performance and fatty acid composition in backfat and belly fat of finishing pigs fed dried distillers grains with solubles. Animal. 9(4):569–575.
- Wood JD, Richardson RI, Nute GR, Fisher AV, Campo MM, Kasapidou E, Sheard PR, Enser M. 2004. Effects of fatty acids on meat quality: a review. Meat Sci. 66(1):21–32.
- Yildiz-Turp G, Serdaroglu M. 2010. Effects of using plum puree on some properties of low fat beef patties. Meat Sci. 86(4):896–900.
- Zhao JX, Li K, Su R, Liu WZ, Ren YS, Zhang CX, Du M, Zhang JX. 2017. Effect of dietary tartary buckwheat extract supplementation on growth performance, meat quality and antioxidant activity in ewe lambs. Meat Sci. 134:79–85.