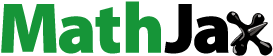
Abstract
The residual feed intake (RFI) and residual intake and gain (RIG) are indices that measure ruminant feed efficiency. Their application has become alternatives to improve the profitability of intensive lamb production systems. This study aimed to evaluate the accuracy of RFI and RIG to measure the non-carcase organ size and cavitary fat of lambs. Thirty non-castrated male lambs were fed for 92 days and slaughtered, and non-carcase organs were weighed. RFI and RIG were classified in low, medium and high efficiency groups, and correlated to carcase and non-carcase organ size. The average RFI values were 0.07, 0.00, and −0.07 kg DM/d and the RIG values were 1.86, −0.20, and −1.91, for high, medium and low, respectively. Low-RFI lambs had lower (p < .05) dry matter intake (DMI) and percentage of DMI standardised by metabolic weight. High-RIG lambs had a higher feed conversion ratio (p ≤ .05) and tended (p < .10) towards higher average dairy gain. The efficient lambs (low-RFI and high-RIG) had a higher heart weight (p ≤ .05). Trends (p < .10) towards higher blood volume and lower relative weight in omental and total cavitary fat deposits were found in lambs with low RFI. Lambs classified as low-RFI and high-RIG had larger hearts, which could be related to improved cardiovascular performance and feed efficiency.
Heart size is related to feed efficiency.
Lambs with improved feed efficiency have larger hearts.
High-RIG lambs have higher intestinal mass.
Highlights
Introduction
The residual feed intake (RFI) and residual intake and gain (RIG) are two indices to measure the feed efficiency of livestock. They have become popular in recent decades because they are independent of body size and productivity (Koch et al. Citation1963; Berry and Crowley Citation2012). Both indices have become alternative measures applied to improve the profitability of intensive sheep meat production systems, where feed represents more than 70% of total production costs (Lima et al. Citation2017).
The RFI, proposed by Koch et al. (Citation1963), is estimated by the difference between the actual feed intake and the expected feed intake for a given live weight and productive level during a period of time, and its objective is to identify the most efficient individuals in the use of feed, to enable selective breeding for genetic improvement (Arthur and Herd Citation2008). In turn, RIG aims to identify individuals with higher growth rates, to allow reducing confinement and slaughter times because commercial weights are reached at younger ages, in addition to having the advantage of improving feeding efficiency (Berry and Crowley Citation2012; Carneiro et al. Citation2019).
Among the biological mechanisms that contribute to the variation in feeding efficiency, non-carcase components have been reported to contribute 5% (Richardson and Herd Citation2004). The variation in the size and functionality of the liver and organs of the gastrointestinal tract is associated with a high metabolic cost, affecting the energy requirements of basal metabolism (Kenny et al. Citation2018). In addition, there is a relationship between feed intake and energy used to digest it, whereby higher intake is associated with greater the energy expenditure, due to an increase in the size of the digestive organs and the energy expended within the tissues of these organs (Herd and Arthur Citation2009). In this regard, it has been reported that greater feed efficiency of lambs is related to lower dry matter intake (DMI), higher feed conversion ratio (FCR) and smaller size of organs other than the carcase. For example, Zhang et al. (Citation2017) reported that low-RFI lambs had a difference of 240 g/d of DMI compared to inefficient ones, smaller size of liver, lungs, kidneys and total stomach weight, and greater length of the duodenum and ileum. In turn, Carneiro et al. (Citation2019) reported that high-RIG lambs had higher average daily gain (ADG, 0.31 versus 0.26 kg/d), improved FCR (3.91 versus 4.99 kg), larger size of skin and hooves, and smaller size of testicles and cavitary fat deposits (omental, mesenteric and total fat). Montelli et al. (Citation2021) reported smaller size of lungs and diaphragm in high-RFI lambs.
High feed efficiency of animals is very important to increase the profitability of production systems and reduce the environmental impacts of livestock breeding, since animals with low-RFI have lower DMI and produce less enteric methane per unit of dry matter consumed (Nkrumah et al. Citation2006; Fitzsimons et al. Citation2013).
In Mexico, sheep production is one of the largest livestock activities, with an inventory of 8,708,246 head according to the most recent census (SIAP Citation2020), and Pelibuey is the most numerous tropical breed because of its maternal ability, high prolificacy, rusticity, resistance to parasites and good adaptation to tropical climates in Mexico (Chay-Canul et al. Citation2016). Nevertheless, there is little information about the efficiency of RFI and RIG as measures to guide herd management of tropical sheep breeds. It is important to validate new indices to select more efficient ewes and rams in order to reduce the production costs and select animals for breeding programs to improve genetic merit. Therefore, the aim of this study was to evaluate the RFI and RIG indices, and their relationship with non-carcase components, organ size, and internal fat in tropical Pelibuey lambs.
Methodology
The lambs included in this study were managed according to the guidelines for the use and care of animals destined for research in the tropics, and this study was approved by the Committee on the Use and Care of Animals of Colegio de Postgraduados, Mexico (SUBINVTAB.CP. −105/18).
Location, management and diet
The study was carried out at the Centro Integral de Ovinos del Sureste (CIOS), located in Villahermosa, Tabasco, Mexico (17°78″N, 92°96″W; 10 m altitude); with humid tropical climate. Thirty non-castrated male Pelibuey lambs with age of 123 ± 36 d and initial body weight (BWi) of 21 ± 5 kg were used. Lambs were housed in individual pens (2 × 2 m). The fattening period lasted 92 days after previous adaptation of 15 days. The diet provided contained 80% concentrated feed and 20% hay on a dry basis (Table ). Fresh water was always available. The lambs were fed ad libitum at two times (08:00 and 15:00 h), and the feed was offered in excess to obtain a minimum feed rejected daily of 10%. The diet was formulated based on the energy (11 MJ/kg DM) and protein (85 g/d) requirements for a lamb weighing 20 kg with an ADG of 250 g, according to the AFRC (Citation1993).
Table 1. Ingredients and composition of experimental diet.
Samples of feed offered and individual orts were collected during the week 3, 6, 9, 12, and were dried in a forced air oven at 55 °C for 72 h and then were ground on a Wiley mill with a 1 mm screen to obtain composite samples at the end of the trial. The DM content (930.15) was determined according to official method of the Association of Official Analytical Chemists (AOAC, Citation1990).
Lamb performance
The individual DMI was calculated daily by the difference between the feed offered and rejected on a dry basis. Body weight (BW) was recorded weekly, initial BW (BWi) was determined after the adaptation period, and final BW (BWf) was recorded 92 days after measuring feed efficiency. The mean body weight (MBW) was calculated once a week, from which the mean metabolic weight (BW0.75) was calculated.
The BW was modelled over time by linear regression to determine ADG using the REG procedure (SAS Citation2012). FCR was calculated as the ratio between DMI and ADG.
Feed efficiency
The residual feed intake (RFI) and residual gain (RG) indices were modelled by multiple linear regression using the REG procedure (SAS Citation2012). The independent variables were ADG, DMI, and BW0.75 (Koch et al. Citation1963), resulting in following equations:
Subsequently, the equation RFI was used to determine the expected DMI (DMIe) and the equation RG for expected ADG (ADGe). The residuals of the RFI index were calculated as the difference between the DMI and DMIe. Likewise, the residuals of the RG index were calculated as the difference between ADG and ADGe. Residual intake and gain (RIG) was calculated by the formula RIG = (−1 × RFI) + RG, as described by Berry and Crowley (Citation2012). Previously, the residuals of the RFI and RG indices were standardised (mean = 0 and standard error = 1) using the STANDARD routine (SAS Citation2012). Lambs were classified as low-RFI and low-RIG (<0.5 SE below mean), medium-RFI and medium-RIG (±0.5 SE from mean), and high-RFI and high-RIG (>0.5 SE above mean).
Weights of visceral organs, gastrointestinal tract, fat deposits and non-carcase components
Lambs were slaughtered according to the Mexican Standard NOM-033-SAG/ZOO-2014 after fasting for 18 h, and the slaughter weight (SBW) recorded. After slaughter, the blood was collected in plastic bags and the weight recorded. The weights of the visceral organs (spleen, heart, liver, lungs and trachea, pancreas, kidneys), gastrointestinal tract (empty stomach and intestines), cavitary fat deposits (total, mesenteric, omental and perirenal fat) and non-carcase external components (head, hooves, skin, and testicles) were immediately recorded. Empty body weight (EBW) was calculated by the difference between the SBW and the content of the gastrointestinal tract. The proportional weight of each organ, fat deposits and non-carcase external components was calculated in relation to EBW.
Statistical analysis
A completely randomised design was used, where each lamb was an experimental unit. For the statistical analysis, the GLM procedure (SAS Citation2012) was used. Least mean squares were calculated and compared using the Tukey test at significance of p < .05.
Results
In this study, 30% of the lambs presented negative RFI values and were classified as low-RFI (efficient); 30% of lambs had positive RFI values and were grouped in the high-RFI class (inefficient); and the remaining 40% were classified as medium-RFI (Table ). In the RIG index, 40% of the lambs had a positive value and were classified as high-RIG (efficient), 37% had negative RIG and were grouped in the low-RIG class (inefficient), and the remaining 23% were medium-RIG (Table ). Low-RFI lambs had lower DMI and DMI percentage expressed in g/kg of BW0.75 compared to high-RFI animals (Table ), while high-RIG lambs had improved FCR (p < .05) compared to low-RIG lambs. The EBW was not significant (p > .05) for both feed efficiency indices (RFI and RIG, Table ).
Table 2. Least square means and standard errors of the mean (SEM) for empty body weight (EBW) and feed efficiency traits of Pelibuey lambs classified using residual feed intake (RFI) and residual intake and gain (RIG).
The carcase yield was not significant (p > .05) for both feed efficiency indices (RFI and RIG, Table ), but it had a significant effect on absolute (p ≤ .05) and relative (p ≤ 0.01 and p ≤ .05, respectively) heart weights. The efficient lambs (low-RFI and high-RIG) had heavier hearts than the inefficient lambs (high-RFI and low-RIG), without differences with the intermediate groups (except for relative weight in the RFI index, Table ). Low-RFI and high-RIG lambs had hearts with greater relative weight (0.05 and 0.06%, respectively) compared to high-RFI and low-RIG lambs. The RIG index had a significant effect (p ≤ .05) on absolute intestine weight. Lambs with high RIG had 240 g heavier intestine weight than those with medium RIG, and non-significant differences of 190 g higher in the absolute weight of intestines in high-RIG lambs compared to low-RIG animals were found (Table ). Except for the heart and intestines, no significant differences (p > .05) were found in the weights of the other visceral organs evaluated by both feed efficiency indices (Table ). However, trends (p < .1) to higher weight (absolute and relative) of blood were observed in lambs with low RFI compared to lambs with high RFI (Table ).
Table 3. Least square means and standard errors of the mean (SEM) for carcase and visceral organ size in Pelibuey lambs classified using residual feed intake (RFI) and residual intake and gain (RIG).
The amounts of total, omental, perirenal and mesenteric cavitary fat were not related to either feed efficiency index (Table ). However, trends (p < .1) to lower relative weight of total and omental cavitary fat deposits were observed in lambs with low RFI compared to lambs with high RFI (Table ).
Table 4. Least square means and standard errors of the mean (SEM) for visceral fat deposit weight in Pelibuey lambs classified using residual feed intake (RFI) and residual intake and gain (RIG).
Significant differences (p < .05) were observed in absolute hooves weight for the RIG index (Table ). Lambs classified with high-RIG had larger hooves weights than low-RIG lambs, and no difference was observed with medium-RIG lambs (Table ). The weight of head, skin, and testicles did not differ (p > .05) according to both feed efficiency indices (Table ).
Table 5. Least square means and standard errors of the mean (SEM) for non-carcase external component size in Pelibuey lambs classified using residual feed intake (RFI) and residual intake and gain (RIG).
Discussion
As expected, the EBW and the size of the carcase did not differ when using both indices. This is explained because the residual feed intake (RFI) and residual intake and gain (RIG) are estimated independently of body size (Koch et al. Citation1963; Berry and Crowley Citation2012). In this study, the low-RFI lambs had lower DMI, with a difference of 10.33 g/kg of BW0.75 compared to high-RFI lambs, without affecting the growth rate. This agrees with the results of other hair breed lambs (Lima et al., Citation2017; Montelli et al., Citation2019). High-RIG lambs had improved FCR, with a difference of 1.04 kg, and similar DMI in comparison with low-RIG lambs, which also agrees with the results for other hair breed lambs (Lima et al., Citation2017; Carneiro et al., Citation2019; Montelli et al., Citation2019). Efficient animals may have lower energy requirements for maintenance (Castro-Bulle et al. Citation2007; Gomes et al. Citation2012), which allows more ingested nutrients to be distributed towards growth (Redden et al. Citation2013). The results reported by Montelli et al. (Citation2021) showed that efficient lambs (low-RFI and high-RIG) have higher concentrations of the IGF-1 hormone compared to inefficient lambs (99.5 versus 79.7 for RFI, and 88.8 versus 73.9 for RIG). Therefore, efficient lambs have increasing muscle mass, since this hormone is involved in the regulation of metabolism and growth (Rechler and Nissley Citation1991). In this sense, efficient lambs (low-RFI and high-RIG) have more efficient metabolism, since they require less feed.
On the other hand, the size of the visceral organs and gastrointestinal tract has a high metabolic cost, so it is likely that the variation between animals in the size and functionality of these organs can impact the energy requirements (Fitzsimons et al. Citation2017). Although the gastrointestinal tract and liver account for a small proportion of body weight (6–13%), they utilise 38–50% of the maintenance energy required to absorb and metabolise digested nutrients (Burrin et al. Citation1990; Seal and Reynolds Citation1993). It has been reported that efficient beef cattle have smaller organs, such as liver (Basarab et al. Citation2003; Bonilha et al. Citation2009, Nascimento et al. Citation2016), gastrointestinal tract (Basarab et al. Citation2003; Bonilha et al. Citation2009) and empty reticulum-rumen (Fitzsimons et al. Citation2014). Additionally, efficient individuals have lower DMI, so there is a relationship between feed intake and the energy used to digest it (the higher the intake, the greater the energy expenditure), due to an increase in size of the digestive organs and the energy expended within the tissues of these organs (Herd and Arthur Citation2009).
In this study, we did not observe differences in liver and stomach weight between efficient and inefficient lambs. Differences in the absolute weight of intestines between medium-RIG and high-RIG lambs were found. Although the low-RIG group presented an absolute weight similar to that of efficient lambs, numerical differences were appreciated. These differences numerical and statistical were 190 and 240 g greater in efficient lambs, respectively, which suggests that greater gut mass improves nutrient absorption in high-RIG lambs, resulting in higher feed efficiency (Zhang et al. Citation2017, Fitzsimons et al. Citation2017). Zhang et al. (Citation2017) reported that low-RFI lambs had greater duodenum and ileum length than high-RFI lambs. Montanholi et al. (Citation2013) reported that bulls with low RFI had a greater number of cell nuclei in the duodenum and ileum compared to high-RFI animals, indicating that efficient bulls have a more metabolically active small intestine, a trait that improves absorption of nutrients and energy efficiency.
Regarding the size of visceral organs, our results showed that lambs with low RFI and high RIG had larger hearts, which could be due to greater cardiovascular efficiency and improved feed efficiency. The data reported by Munro et al. (Citation2015) indicated greater relative weight of the right ventricle (22.0 versus 21.3%, p = .04) and of the total ventricular weight (86.6 versus 85.9%, p = .05) in relation to the whole heart weight, in cattle with low RFI. In addition, a positive correlation (0.29, p = .005) of thickness of the right ventricle with the RFI index was reported, indicating that cattle with high RFI have increased cardiovascular rate by spending less time resting, increasing DM intake with higher digestibility. The increased cardiovascular flow raises the heart rate and blood volume, and it is assumed that the pressure of the right atrium also increases, which can result in hypertrophy of the right ventricle. Therefore, cattle with high RFI have a higher workload of the right ventricle of the heart, and the selection of cattle with low RFI does not have a negative impact on cardiovascular structure and function (Munro et al. Citation2019). In this sense, a heavier heart and improved cardiovascular rate could explain why lambs with low RFI tend to have greater blood weight.
The difference in heart size found in this study differs from that reported in temperate and tropical sheep breeds, since no difference was reported in the heart weight among the classes using the RFI (Meyer et al. Citation2015; Zhang et al. Citation2017; Montelli et al. Citation2021), and RIG (Carneiro et al. Citation2019; Montelli et al. Citation2021). Likewise, it differs from that reported in cattle classified by RFI index (Basarab et al. Citation2003; Gomes et al. Citation2012; Fitzsimons et al. Citation2014; Nascimento et al. Citation2016) and RIG index (Nascimento et al. Citation2016).
On the other hand, Herd and Arthur (Citation2009) reported a difference in the efficiency of the deposition of lean tissue (40–50%) and cavitary fat (70–95%), and this variation in muscle was due to protein turnover. Lambs with low RFI tend to deposit less cavitary fat (total and omental fat) compared to lambs with high RFI. These results can be explained because lambs with low RFI have lower feed intake (146 g DM/d less compared to high-RFI), and therefore lower energy intake. In this regard, Carneiro et al. (Citation2019) reported that the lambs with high-RIG level had lower relative weight of total cavitary fat (4.95 versus 5.91%), omental fat (1.89 versus 2.30%) and mesenteric fat (1.20 versus 1.48%) compared to lambs with low-RIG values. Montelli et al. (Citation2021) also reported a trend towards lower omental fat deposition in efficient lambs (low-RFI and high-RIG animals) compared to inefficient lambs (0.748 versus 0.876 kg for RFI and 0.786 versus 1.041 kg for RIG, respectively). However, several authors have reported that lambs classified by the RFI index do not differ in the amount of cavitary fat deposited (Meyer et al. Citation2015; Zhang et al. Citation2017; Rocha et al. Citation2018).
Regarding the non-carcase external components, high-RIG lambs showed higher hooves weight. This result agrees with that reported by Carneiro et al. (Citation2019), who found higher relative weight of hooves in crossbred Texel × Pantaneira lambs classified by the RIG index. Likewise, no difference in hooves size has been reported using the RFI index (Zhang et al. Citation2017; Rocha et al. Citation2018), which agrees with our results.
Conclusions
Low-RFI and high-RIG lambs had improved feed efficiency and larger hearts, which could be related to improved cardiovascular performance. In addition, low-RFI lambs tended to have higher blood volume and deposited less cavitary fat, while that high-RIG have higher intestinal mass, which could be related to improved feed efficiency. More experimental studies are required to determine the relationship of feed efficiency with non-carcase organ size in tropical hair sheep. Finally, anatomy and physiology of the heart in tropical hair sheep are topics that need to be further evaluated.
Ethics approval
The animals included in the present study were managed in compliance with the regulations for the use and care of animals intended for research and, and the study was approved by the Ethics Committee on Animal Use and Animal Care of Colegio de Postgraduados (SUBINVTAB.CP. -105/18).
Acknowledgements
The first author is grateful for the research grant provided by the National Council for Science and Technology of Mexico (CONACYT) for his PhD studies at the Colegio de Postgraduados. The authors are grateful for the assistance of Walter Lanz-Villegas, who granted access to the facilities of the Centro de Integracion Ovina del Sureste (CIOS).
Disclosure statement
The authors declare they have no conflicts of interest.
Data availability statement
Data are available from Carlos Arce-Recinos, e-mail: [email protected] upon reasonable request.
Additional information
Funding
References
- [AFRC] Agricultural and Food Research Council. 1993. Technical Committee on Responses to Nutrients. Energy and protein requirements of ruminants. Wallingford (UK): CAB International.
- [AOAC] Association of Official Analytical Chemists. 1990. Official methods of analysis. 15th ed. Washington, DC, EEUU: AOAC International.
- Arthur JPF, Herd RM. 2008. Residual feed intake in beef cattle. R Bras Zootec. 37(spe):269–279.
- Basarab JA, Price MA, Aalhus JL, Okine EK, Snelling WM, Lyle KL. 2003. Residual feed intake and body composition in young growing cattle. Can J Anim Sci. 83(2):189–204.
- Berry DP, Crowley JJ. 2012. Residual intake and body weight gain: a new measure of efficiency in growing cattle. J Anim Sci. 90(1):109–115.
- Bonilha SFM, Branco RH, Corvino TLS, Alleoni GF, Figueiredo LA, Razook AG. 2009. Relationships between residual feed intake and internal organs of Nellore bulls. In: Proceedings of American Dairy Science Association, Canadian Society of Animal Science, American Society of Animal Science Joint Annual Meeting, 12–16 July 2009; Montreal, Quebec, Canada. p. 295.
- Burrin DG, Ferrell CL, Britton RA, Bauer M. 1990. Level of nutrition and visceral organ size and metabolic activity in sheep. Br J Nutr. 64(2):439–448.
- Carneiro MMY, Morais MG, Souza ARDL, Fernandes HJ, Feijó GLD, Bonin MN, Franco GL, Rocha RFAT. 2019. Residual intake and gain for the evaluation of performance, non-carcass components, and carcass characteristics of confined crossbred Texel lambs. Rev Bras Zootecn. 48:e2018206.
- Castro-Bulle FCP, Paulino PV, Sanches AC, Sainz RD. 2007. Growth, carcass quality, and protein and energy metabolism in beef cattle with different growth potentials and residual feed intakes. J Anim Sci. 85(4):928–936.
- Chay-Canul AJ, Magaña-Monforte JG, Chizzotti ML, Piñeiro-Vázquez AT, Canul-Solís JR, Ayala-Burgos AJ, Ku-Vera JC, Tedeschi LO. 2016. Energy requirements of hair sheep in the tropical regions of Latin America. RMCP. 7(1):105–125.
- Fitzsimons C, Kenny DA, Deighton MH, Fahey AG, McGee M. 2013. Methane emissions, body composition, and rumen fermentation traits of beef heifers differing in residual feed intake. J Anim Sci. 91(12):5789–5800.
- Fitzsimons C, Kenny DA, McGee M. 2014. Visceral organ weights, digestion and carcass characteristics of beef bulls differing in residual feed intake offered a high concentrate diet. Animal. 8(6):949–959.
- Fitzsimons C, McGee M, Keogh K, Waters SM, Kenny DA. 2017. Molecular physiology of feed efficiency in beef cattle. In: Scanes CG, Hill RA, editors. Biology of domestic animals. Boca Raton, FL: CRC Press; p. 120–163.
- Gomes RC, Sainz RD, Silva SL, César MC, Bonin MN, Leme PR. 2012. Feedlot performance, feed efficiency reranking, carcass traits, body composition, energy requirements, meat quality and calpain system activity in Nellore steers with low and high residual feed intake. Livest Sci. 150(1–3):265–273.
- Herd RM, Arthur PF. 2009. Physiological basis for residual feed intake. J Anim Sci. 87(14 Suppl):E64–E71.
- Kenny DA, Fitzsimons C, Waters SM, McGee M. 2018. Invited review: Improving feed efficiency of beef cattle – The current state of the art and future challenges. Animal. 12(9):1815–1826.
- Koch RM, Swiger LA, Chambers D, Gregory KE. 1963. Efficiency of feed use in beef cattle. J Anim Sci. 22(2):486–494.
- Lima NLL, Ribeiro CRF, Sá HCM, Júnior IL, Cavalcanti LFL, Santana RAV, Furusho-García IF, Pereira IG. 2017. Economic analysis, performance, and feed efficiency in feedlot lambs. R Bras Zootec. 46(10):821–829.
- Meyer AM, Vraspir RA, Ellison MJ, Cammack KM. 2015. The relationship of residual intake and visceral organ size in growing lambs fed a concentrate- or forage-based diet. Livest Sci. 176:85–90.
- Montanholi Y, Fontoura A, Swanson K, Coomber B, Yamashiro S, Miller S. 2013. Small intestine histomorphometry of beef cattle with divergent feed efficiency. Acta Vet Scand. 55(1):9.
- Montelli NLLL, Almeida AK, Ribeiro CRF, Grobe MD, Abrantes MAF, Lemos GS, Garcia IFF, Pereira IG. 2019. Performance, feeding behavior and digestibility of nutrients in lambs with divergent efficiency traits. Small Ruminant Res. 180:50–56.
- Montelli NLLL, Alvarenga TIRC, Almeida AK, Alvarenga FAP, Furusho-Garcia IF, Greenwood PL, Pereira IG. 2021. Associations of feed efficiency with circulating IGF-1 and leptin, carcass traits and meat quality of lambs. Meat Sci. 173:108379.
- Munro J, Lam S, Macdonald A, Physick-Sheard P, Schenkel F, Miller S, Montanholi Y. 2015. Cardiac function and anatomy in relation to feed efficiency in beef cattle. In: European Federation of Animal Science, Book of Abstracts of the 66th Annual Meeting of the European Federation of Animal Science. Warsaw, Poland: Wageningen Academic Publisher; p. 362.
- Munro JC, Physick-Sheard PW, Pyle WG, Schenkel FS, Miller SP, Montanholi YR. 2019. Cardiac function and feed efficiency: increased right-heart workload in feed inefficient beef cattle. Livest Sci. 229:159–169.
- Nascimento ML, Souza ARDL, Chaves AS, Cesar ASM, Tullio RR, Medeiros SR, Mourão GB, Rosa AN, Feijó GLD, Alencar MM, et al. 2016. Feed efficiency indexes and their relationships with carcass, non-carcass and meat quality traits in Nellore steers. Meat Sci. 116:78–85.
- Nkrumah JD, Okine EK, Mathison GW, Schmid K, Li C, Basarab JA, Price MA, Wang Z, Moore SS. 2006. Relationships of feedlot feed efficiency, performance and feeding behavior with metabolic rate, methane production, and energy partitioning in beef cattle. J Anim Sci. 84(1):145–153.
- Rechler MM, Nissley SP. 1991. Insulin-like growth factors. In: Sporn MB, Roberts AB, editors. Peptide growth factors and their receptors I. New York (NY): Springer Study Edition; p. 263–267.
- Redden RR, Surber LMM, Grove AV, Kott RW. 2013. Growth efficiency of ewe lambs classified into residual feed intake groups and pen fed a restricted amount of feed. Small Ruminant Res. 114(2–3):214–219.
- Richardson EC, Herd RM. 2004. Biological basis for variation in residual feed intake in beef cattle. 2. Synthesis of results following divergent selection. Aust J Exp Agric. 44(5):431–440.
- Rocha RFAT, Souza ARDL, Morais MG, Carneiro MMY, Fernandes HJ, Feijó GLD, Menezes BB, Walker CC. 2018. Performance, carcass traits, and non-carcass components of feedlot finished lambs from different residual feed intake classes. SCA. 39(6):2645–2658.
- [SAS] Statistical Analysis Software. 2012. SAS/STAT: guide of personal computers, version 9.3. Cary, NC: Institute Inc.
- Seal CJ, Reynolds CK. 1993. Nutritional implications of gastrointestinal and liver metabolism in ruminants. Nutr Res Rev. 6(1):185–208.
- [SIAP] Agricultural and Fisheries Information Service. 2020. Sheep livestock population 2010–2019. Secretary of Agriculture and Rural Development, Mexico. [Accessed 2021 May 26]. https://www.gob.mx/cms/uploads/attachment/file/564337/Inventario_2019_ovino.pdf.
- Zhang X, Wang W, Mo F, La Y, Li C, Li F. 2017. Association of residual feed intake with growth and slaughtering performance, blood metabolism, and body composition in growing lambs. Sci Rep. 7(1):12681.