Abstract
The current study was conducted to evaluate the effects of a yeast cell wall product (YCWP) on the performance, the population of Lactobacillus and coliform bacteria and PGC-1α, TLR4, IL-10 and PPARγ genes expression in the intestine of broilers. Dietary treatments were as follows: basal diet (control), control diet + 0.3 g/kg colistin or 0.15, 0.3, 0.45 and 0.6 g/kg YCWP. Feed intake and feed conversion ratio were lower in the YCWP-fed birds compared to the control. Colistin and YCWP fed birds had higher Lactobacillus and lower coliform bacteria counts than control birds (p < .05). Total and IgM antibody against sheep red blood cells was linearly increased by adding the YCWP to the diet (p < .05). Adding the YCWP to the diet linearly increased the caecal concentration of propionate (p < .05). Orthogonal and polynomial contrasts showed that the YCWP significantly improved the villus height, crypt depth, villus height: crypt depth, villus width, and villus surface area (p < .05). The lower concentrations of the YCWP (0.15 and 0.30 g/kg) increased the PPARγ transcription (p < .05), while its upper concentration (0.45 and 0.6 g/kg of diet) did not change the PPARγ gene expression. PGC-1α transcription was increased with 0.15 g/kg of the YCWP supplementation (p < .05). Dietary inclusion of the YCWP decreased the TLR4 gene expression (p < .05) and increased the downstream IL-10 cytokine (p < .05). In conclusion, YCWP improved the broiler’s performance by modifying the gut microflora. Also, YCWP supplementation had an immune regulatory effect via local and systemic responses involving TLR4, IL-10, and PPARγ.
Adding YCWP to the broilers diet did not significantly affect body weight gain, but reduced FCR.
Adding 0.45 and 0.6 g of YCWP to the diet, increased the Lactobacillus number and reduced the coliform bacteria number in the ileum.
YCWP supplementation increased the production of antibodies (total, IgG, and IgM) against SRBC and developed lymphatic organs.
Adding 0.6 g of YCWP to the diet increased villus height and villus surface area and reduced the crypt depth.
YCWP supplement had an immune regulatory effect via local and systemic responses involving TLR4, IL-10, and PPARγ.
Highlights
Introduction
During the last 30 years, scientists have attempted to develop strategies to enhance livestock production, reduce production costs and mitigate the harmful environmental effects. The increase in pathogenic bacteria in the birds’ gastrointestinal tract (GIT) could impair growth and feed utilisation. Such pathogenic bacteria may attach to the various parts of the GIT in the poultry, causing considerable damage to the epithelial layer cells (Vermeulen et al. Citation2001; Ritzi et al. Citation2016). Therefore, there is an immediate need to develop new strategies that can sufficiently protect broiler chickens against the population of harmful bacteria without detrimental effects on the broilers’ performance (Ott et al. Citation2018). Alternative additives to antibiotics are being sought to provide a helpful product for animal production due to the ban and strict standards in using antibiotics. As such, the poultry industry is under immense pressure to decrease the use of antibiotics in commercial production, leading to more research to find the compounds capable of improving the GIT health and immune function in the poultry (Ott et al. Citation2018).
Today, the use of prebiotics in poultry feeds is widely considered to increase the bird’s performance. Prebiotics are defined as the indigestible feeds that can stimulate the growth of one or a limited number of bacteria in the gut, with positive effects on the host (Gadde et al. Citation2017). The most common prebiotics used in poultry feeds are yeast cell wall products (YCWPs) such as inulin, fructooligosaccharides (FOSs), mannan-oligosaccharides (MOSs), β-glucan, xylooligosaccharides, and galactooligosaccharides (GOSs) (Ricke Citation2018). For example, MOSs are oligomers made of mannose linked together by β-1, 4 glycoside bonds; they are found in the cell wall of Saccharomyces cerevisiae (Pourabedin et al. Citation2014). Mannan-oligosaccharides are usually derived from yeast cells, which modulate the immune system and eliminate pathogenic bacteria from the GIT. Mannans present in MOSs can bind to mannose-specific type-I fimbriae in gram-negative bacteria (such as E. coli, Salmonella and Clostridium), preventing them from attaching and colonising the intestinal epithelium (Suresh et al. Citation2018).
To find out how YCWP is working on the surface of the GIT cells, the present study investigated the expression of genes that are possibly involved in the regulation of metabolism and reaction to the pathogenic bacteria and lipopolysaccharides (LPS) in the epithelial cells under the YCWP treatment. Peroxisome proliferator-activated receptor-γ coactivator (PGC-1α) is a transcriptional coactivator characterised as a regulator of mitochondrial biogenesis and function, including oxidative phosphorylation and detoxification of the reactive oxygen species. PGC-1α is highly expressed in the tissues with high-energy demands (Rius-Pérez et al. Citation2020). PGC-1α also regulates the expression of mitochondrial antioxidant genes, which alleviate oxidative injuries and mitochondrial dysfunctions. Dysregulation of PGC-1α exacerbates the inflammatory response, which is commonly accompanied by a metabolic disorder. Following the inflammation, the small levels of PGC-1α downregulate mitochondrial antioxidant gene expression, persuade oxidative stress and promote the nuclear factor kappa B activation. Toll-like receptors (TLRs) is one of the vital plasma membrane receptors that could create connections between luminal pathogens and genes expression of intestinal epithelial cells involved in metabolism such as PGC-1α . TLRs are a class of proteins that play a crucial role in the innate immune system, serving as the first line of the host’s response against besetting microbial pathogens (Luo et al. Citation2014). Various factors can affect TLRs expression and modulate TLRs signalling pathways, leading to the release of endogenous molecules serving as TLR ligands and the responsiveness of innate and adaptive immune systems to combat pathogenic bacteria (Arslan et al. Citation2011; Napper et al. Citation2015; Paul et al. Citation2015). Previous studies demonstrated that TLR4 transmits LPS signals (Yang et al. Citation1998). However, rearing conditions could modulate the inhibition of the GIT microflora of broilers for the recognition of pathogen-associated molecular patterns to occur by TLRs, enabling the activation of the immune cells in the GIT (Li et al. Citation2020). Besides, resistance to the pathogens has been shown to be closely correlated with the up-regulation of TLR4, as well as various chemokines and cytokines (Heni et al. Citation2020; Takizawa et al. Citation2020). Therefore, PGC-1α acts as a necessary factor connecting metabolic modifications and inflammatory pathways. It is, therefore, an attractive target gene that has considerable benefits for the regulation of the GIT homeostasis under the effect of bacterial populations (Rius-Pérez et al. Citation2020). There is, however, limited information regarding the effects of YCWP on the expression of genes involved in the immune responses of broiler chickens, including peroxisome proliferator-activated receptor γ (PPARγ), PGC1α, TLR4 and interleukin-10 (IL-10). Based on the previous studies, we hypothesised that the effects of YCWP on the immune system of the broiler chickens could be mediated by regulating the gene expression involved in inflammation and mitochondrial energy metabolism. Therefore, this study aimed to evaluate the effects of adding a YCWP to the broiler diets on the performance, the population of Lactobacillus and coliform bacteria in the ileum, morphology of jejunum, immune system and PPARγ PGC1α, TLR4 and IL-10 genes expression, which have not been previously explored.
Materials and methods
Birds management and experimental design
This experiment was conducted according to the comprehensive guide of animal welfare, as adopted by FASS (Citation2010). The Animal Policy and Welfare Committee of the Isfahan University of Technology approved all animal care and experimental procedures. This experiment was conducted on a broiler research farm. This broiler research house was equipped to optimise the environmental condition and provide the best animal welfare during the experimental period. The uniform environmental conditions between all experimental units (floor pen) provided by synchronising of heating systems (floor heating system and warm air tunnels), sidewall and tunnel fans, cooling systems (the cooling pads were located behind sidewall inlets), fogging system (one nozzle was situated in front of each sidewall inlet) and controlled through a computerised ventilation system controller. The temperature was maintained at 33 °C for the first two days and then reduced gradually to 22 °C until 39 days of age. The light regime was 23 L: 1 D for the first three days and then 20 L: 4 D till the end of the experiment. Chickens were fed ad libitum with the standard mash diets (based on Ross 308 recommendation, Table ) during the starter (1–10 d), grower (11–24 d), and finisher phases (25–39 d).
Table 1. The composition and nutrient content of the control diet (g/Kg, as-fed basis).
A total of 1440 day-old as-hatch broilers (Ross 308) with an average weight of 37.35 ± 0.82 g were used in a completely randomised design with six treatments and ten replicates of 24 chickens. The treatment groups included corn-soy based diet (control), control + 0.15 g/kg of YCWP, control + 0.3 g/kg of YCWP, control + 0.45 g/kg of YCWP, control + 0.6 g/kg of YCWP, and control + 0.3 g/kg of colistin antibiotic. In this experiment, YeaMune-UP® (Pathway Intermediates Company, Seoul, South Korea) was used as a YCWP supplement. The YeaMune-Up is a functional fermented product made from Saccharomyces cerevisiae boulardii; it is rich in β-glucan and mannan-oligosaccharides (MOS). The body weight of each bird was recorded individually on days 10, 24, and 39 of age after 2 hours of fasting. Feed intake was recorded at 10, 24, and 39 d of age. The average daily weight gain, average daily feed intake, feed conversion ratio (FCR), and European efficiency factor (EEF) were calculated accordingly. Also, at 40 days of age, one male bird from each replicate was selected and humanely euthanized by CO2 for samples collection.
Ileal sample collection for detecting the Lactobacillus and Coliform bacteria
Ileal contents of six chickens from each treatment were used to enumerate Lactobacillus and coliform bacteria at the end of the experiment. One g of digesta was collected (from the last 10 cm of the ileum) and mixed with 9 mL of peptone water broth; 100 µL of the solution was then homogenised in 900 µL phosphate-buffered saline (PBS) and serially (1:10) diluted. The population of Lactobacillus was counted on the De Man, Rogosa, and Sharpe (MRS) agar (Man, Rogosa, and Sharpe agar) and incubated in an anaerobic incubator at 37 °C for 48 hours. The coliform bacteria were also counted on the MacConkey agar and incubated at 37 °C for 24 hours. The results were expressed as log10 of colony-forming units (CFU) per gram of ileal digesta.
Analysis of cecal short chain fatty acids (SCFAs)
To measure the concentration of short-chain fatty acids in the caecum, ten birds from each treatment were chosen randomly at the age of 40 days; the caecum contents for each were collected and stored at −20 °C. One g of caecum contents was suspended in 4 mL of distilling water in a sterile tube. The samples were homogenised and centrifuged at 4,000 × g for 15 min. One mL of the supernatant was then transferred into an Eppendorf tube and mixed with 250 µL metaphosphoric acid solution. The solutions were then centrifuged again at 4,000 × g for 15 min. After that, 1 mL of the supernatant was transferred into an Eppendorf tube mixed with 200 µL crotonic acid solution as the internal standard. The solutions were centrifuged again at 4,000 × g for 15 min. The supernatant was analysed by gas chromatography (GC) for the concentrations of acetate, propionate, butyrate, isobutyrate, valerate, and isovalerate (Zhang et al. Citation2003).
Analysis of the immune system response
Weighing the lymphatic organs
At the end of the experiment, lymphoid organs such as the bursa of Fabricius, thymus, and spleen were separated and weighed individually from ten euthanized chickens per treatment. The relative lymphoid organs weight was calculated and presented as a percentage of live body weight.
Anti-SRBC antibody assay
Ten birds from each treatment were injected with 0.5 mL of 5% suspension of sheep red blood cell (SRBC) on days 22 and 29 of age to evaluate the antibody production potential (Seidavi et al. Citation2017). Blood samples were collected 7 and 14 days after the first injection. The serum of each sample was isolated and frozen at −20 °C. Anti-SRBC antibody was measured using the microhemagglutination assay in 96 well microplates, as described by Akhlaghi et al. (Akhlaghi et al. Citation2013).
Intestinal morphological analysis
For enteric morphology analysis, at the end of the experiment, the jejunal samples were collected. One cm of the jejunal's midpoint from each bird was removed and fixed in 10% buffered formalin. Samples were prepared as described by Ekim (Ekim et al. Citation2020) for evaluation by optical microscope (Olympus CX31, Tokyo, Japan) and photographed with a digital microscope camera. Crypt depth, height and width of villi, and muscular layers thickness were measured using ImageJ software (Sakamoto et al. Citation2000; Ekim et al. Citation2020). The formula for calculating the villus surface area was 2π × (villus width/2) × villus height (Prakatur et al. Citation2019).
Samples preparation and total RNA extraction
At the age of 40 days, 2 cm of the ileum of 3 chickens from each treatment was obtained for gene expression analysis. The collected tissue samples were washed with distilled water and immediately frozen in liquid nitrogen and stored at −80°C until further use. Total RNA was isolated from the intestine tissue samples of the broilers separately using TRIzol (Sinaclon, Tehran, Iran), according to the manufacturer’s protocol; RNase-free DNaseI was used to remove DNA contamination. Dried RNA samples were dissolved in DEPC water. RNA integrity was verified by agarose gel electrophoresis and visualisation of the glyceraldehyde 3-phosphate dehydrogenase (GAPDH) expression RNA. The concentration of the RNA samples was determined using Nano Drop-2000 (Thermo, USA).
Real-time quantitative RT-PCR (qRT-PCR) analysis
The expression of four candidate genes, including PPARγ, PGC-1α, TLR4, and IL-10, was determined using quantitative reverse transcription-PCR (qRT-PCR, ABI StepOne™ Real-Time PCR System – Thermo Fisher Scientific). The reaction was performed using RealQ Plus 2x Master Mix Green (Amplicon). Primers were designed based on the target genes sequences and blasted with the NCBI Blast Primer; then, they were synthesised commercially (TAG, Copenhagen, Denmark, Table ). The primers are listed in Table . Complementary DNA (cDNA) was synthesised from the total RNA using cDNA synthesis® RT reagent Kit (Sinaclon). For each sample, 25 ng of cDNA was used as a template in the final volume of 25 μL reaction. The thermal cycling conditions had an initial denaturation step at 95 °C for 10 min; this was followed by 40 cycles, including a denaturation step at 95 °C for the 30 s, and the annealing and extension step at 60 °C for 30 s. The GAPDH gene was used as an internal control. Each experiment was performed in triplicate and repeated three times independently. The triplicate PCRs' cycle threshold (Ct) values were averaged, and the relative quantification of the transcript levels was performed using the comparative 2−ΔΔCT method. The fold change in the target gene, relative to GAPDH, was determined based on the following formula: fold change= 2−ΔΔCT, where ΔCT = CT (a target gene) −CT (a reference gene), ΔΔCT = ΔCT (a target sample) −ΔCT (a reference sample). Each sample was further amplified without reverse transcription to confirm that no DNA contamination was in the sample.
Table 2. Specific amplification of the gene and internal reference primer.
Statistical analyses
Data were analysed using the GLM procedures of SAS 9.4 statistical software (SAS (Statistical Analysis System) Citation2009), based on a completely randomised design (CRD). Tukey’s test was then used to determine the difference between treatments. The significance was considered at p < .05. Linear and quadratic effects from feeding control, 0.15 g YCWP, 0.3 g YCWP, 0.45 g YCWP and 0.6 g YCWP treatments were analysed using the polynomial contrasts. Additionally, the orthogonal contrast was used in SAS 9.4 (2009) to test the control versus YCWP-supplemented birds.
Results
Growth performance
The effects of adding YCWP to the experimental diets on the performance of the birds during the experimental periods are shown in Table . Dietary treatments did not affect ADWG, ADFI, and FCR of the broilers during the starter period (p > .05). The ADWG was similar in different dietary treatments during the grower and finisher periods, but the inclusion of the YCWP linearly decreased ADFI and FCR (p < .05). The same results were found in the overall period, where the lowest FCR was related to the birds fed with 0.45 and 0.6 g/kg YCWP treatments (p < .05). There was no significant difference among experimental diets in terms of EEF during the overall period (p > .05). Contrast analysis also showed that during the grower, finisher and overall periods, adding YCWP to the diets significantly reduced FCR, as compared to the control group (p < .05). Also, with increasing the level of the YCWP in the diet, FCR and ADFI were decreased linearly.
Table 3. Effect of adding yeast cell wall product (YCWP) to the diet on performance during experimental periods.
Ileal bacterial population
The effects of dietary inclusion of the YCWP on the microbial population in the ileal content are shown in Table . Chickens fed with the 0.45 and 0.6 g of YCWP and colistin had higher Lactobacillus counts in comparison to those fed the control diet (p < .05). Also, dietary inclusion of 0.45 g/kg of the YCWP reduced the coliform bacteria counts compared to the control treatment (p < .05). The contrast comparison showed that the addition of YCWPs to the diet significantly increased Lactobacillus, while it decreased coliform bacteria than the control birds (p < .05). Also, with increasing the YCWP level in the diet, the population of Lactobacillus was increased, while the population of coliform bacteria was decreased linearly (p < .05).
Table 4. Effects of yeast cell wall product (YCWP) on microbial population in the ileal contents (Log10 CFU/g).
Immune system responses
Antibody response data (anti-SRBC) was measured as the serum concentrations of total anti-SRBC antibodies, IgG, and IgM (Table ). In the primary (29 d) and secondary (36 d) responses, the production of anti-SRBC antibodies was not significantly affected by the dietary treatments (p > .05). However, the results of the secondary response showed that those chickens fed with the YCWP had higher levels of anti-SRBC antibodies and IgM, as compared to the control ones (p < .05). Also, the level of anti-SRBC antibodies and IgM was increased linearly with raising the level of the YCWP in the diet (p < .05). At the end of the experiment, the lymphoid organs were weighted; the related data as a percentage of the live body weight are also presented in Table . Chickens fed with 0.45 g YCWP had the highest weight of thymus and bursa of Fabricius (p < .05). Besides, the weight of the thymus was increased linearly with raising the level of the YCWP in the diet (p < .05). The spleen weight was similar in different dietary treatments (p > .05).
Table 5. Effect of yeast cell wall product (YCWP) and antibiotic in response to SRBC and weight of lymphoid organs at the end of the experiment.
The concentration of cecal SCFAs
The concentration of SCFAs (acetate, propionate, butyrate, isobutyrate, valeric, isovaleric and total volatile fatty acids) in the caecum on the 40th day can be seen in Table . The SCFAs concentration was not affected by the dietary treatments (p > .05). However, the addition of YCWP increased the propionate concentration, as compared to the control group (p < .05); consequently, it tended to decrease the acetate to propionate ratio (p = .055). Also, the concentration of propionate in the caecum was increased linearly with raising the level of the YCWP in the diet (p < .05).
Table 6. Effect of adding yeast cell wall product (YCWP) to the diets on the concentration of short-chain fatty acid in the caecum at 40 days of age (mmol/g).
Histological changes of the jejunum
The results of the assessed histological parameters of the jejunum (villus height, crypt depth, villus width, villus surface area, and muscle thickness) are shown in Table and . Adding the YCWP to the diet increased the villus height linearly (p < .05). The highest and shortest villus height was observed in the birds fed with 0.6 g of the YCWP and colistin, respectively (p < .05). The control birds had the deepest crypt, as compared to those fed the diets containing colistin and the YCWP (p < .05). Also, the highest and lowest VH: CD ratio was related to 0.6 g YCWP and control treatments, respectively (p < .05). Furthermore, with increasing the level of the YCWP in the diet, the width of the intestinal villus was increased linearly (p < .05); so, the maximum villus width was observed in the birds receiving 0.45 and 0.6 g of the YCWP, while the lowest villus width was observed in those fed the control, 0.15 and 0.3 g YCWP. Chickens fed with 0.6 g YCWP had the highest villus surface area, while those using colistin and 0.15 g of YCWP had the lowest villus surface area (p < .05). The muscle thickness was similar in treatments (p > .05). According to the results of the contrast analysis, birds whose diet was supplemented with YCWP had a lower crypt depth and higher VH: CD, as compared to those receiving the control diet (p < .05). Also, with the increasing the YCWP level in the diet, VH: CD and villus surface area were increased linearly, while the crypt depth was decreased linearly and quadratically (p < .05).
Figure 1. Effect of yeast cell wall product (YCWP) and antibiotic on jejunal histological changes in broilers at 40 d of age. Arrows: (a) villus height; (b) villus width; (c) crypt depth; d: muscle thickness.
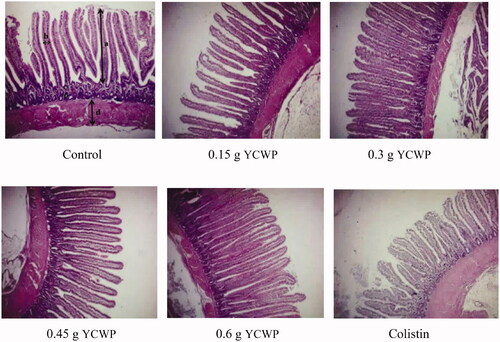
Table 7. Effect of adding yeast cell wall product (YCWP) to the diets on histological changes of the jejunum.
Gene expression
The results of the gene expression are presented in Figure . Feeding birds with 0.15 and 0.3 g of the YCWP increased PPARγ transcription, while the inclusion of the YCWP at the level of 0.45 and 0.6 g did not change the PPARγ gene expression, as compared to the control birds (p < .05). As demonstrated in Figure , the PGC1-α mRNA level was increased in the chickens fed with 0.15 g YCWP (p < .05). However, birds fed the diet containing other levels of the YCWP did not change PGC1-α mRNA expression, as compared to the others (p < .05). Colistin did not change PGC1- α gene expression than the control group. The TLR4 gene expression in 0.3 and 0.45 g YCWP-fed birds was significantly lower than that in the control birds (Figure ; p < .05). However, the inclusion levels of 0.15 and 0.6 g YCWP did not change the gene expression of TLR4, as compared to the control birds (p < .05). The effects of the dietary treatments on the gene expression of IL-10 are presented in Figure . As expected, feeding birds with 0.15 and 0.3 g of YCWP/kg of the diet resulted in the greater transcription of the IL-10 gene. However, at the greater inclusion levels (0.45 and 0.6 g/kg of diet), YCWP supplementation and colistin did not change the IL-10 expression, as compared to the control group (p < .05). The orthogonal contrasts showed that the mRNA for PPARγ (p = .021), PGC-1α (p = .000) and IL-10 (p = .012) were elevated in the YCWP group, while TLR4 mRNA (p = .002) was decreased with adding YCWP compared to the control diet (Figure ).
Discussion
In this study, the effects of the dietary inclusion of YCWP on the growth performance of the broiler chickens reared under normal physiological conditions were evaluated. prebiotics and YCWP supplementation in the broiler chickens generally improves their performance (Teng and Kim Citation2018; Ricke et al. Citation2020); however, in this experiment, YCWP (MOS and β-Glucan) supplementation only improved the FCR of chickens. These results were in agreement with those of Pourabedin et al, reporting that adding MOS to the broilers diet had no significant effect on their daily weight gain. However, in their study, YCWP supplementation did not influence the FCR of 35-d-old broilers, which was in contrast with our findings (Pourabedin et al. Citation2014). Similar results have also been obtained in the birds fed with MOS and grown under an experimentally controlled condition (Sims et al. Citation2004). Improving the performance of broiler chickens by dietary supplementation with β-glucans or MOS could be mainly attributed to the modulation of the intestinal microbiota and enhanced innate immune function (Kim et al. Citation2011). For example, in a recent study, it was reported that adding prebiotics (containing 2.6 Beta LevaFructan 100 gm on 1000 mL distilled water) to the water reduced the adverse effects of Salmonella and increased the performance of broiler chickens during the bacterial challenge (El-Shall et al. Citation2019). It has also been reported that prebiotics (isomaltooligosaccharide) could significantly improve the growth performance and modulate the broiler chickens' intestinal microbiota when challenged by E.coli O78 (Tarabees et al. Citation2019). In the present study, the experimental birds were reared in a controlled environment and not exposed to any exogenous bacteria or environmental challenge; this may explain why adding the YCWP to the diets had no effect on the bird’s performance in the present study (Wang et al. Citation2016).
In general, yeast cell wall products and prebiotics modulate the microbial population of GIT by increasing the number of beneficial bacteria such as Lactobacillus and Bifidobacteria and decreasing such pathogenic bacteria as Salmonella and E.coli (Zoumpopoulou et al. Citation2018). In this experiment, we observed that with increasing the YCWP levels in the diet, the number of Lactobacillus in the ileum was significantly increased, resulting in decreasing the number of coliform bacteria in the ileal content (Table ). Prebiotics could affect the gut microbiota in several ways: 1. providing the nutrients for the growth of commensal bacteria, 2. inhibiting the growth of pathogenic bacteria by increasing the development of commensal bacteria and creating competitive exclusion between them, and preventing the attachment of pathogenic bacteria to the intestinal epithelial sites (Pourabedin and Zhao Citation2015; Adhikari and Kim Citation2017), and 3. binding to the pathogenic bacteria, which possess type-1 fimbriae such as E. coli and Salmonella species. Mannan oligosaccharides have receptor properties for E. coli and Salmonella's fimbriae that can prevent them from attaching to the intestinal epithelium and removing with the digesta flow; MOS can also block the bacterial lectins and reduce the colonisation of these pathogens in the bird’s GIT (Fernandez et al. Citation2002; Teng and Kim Citation2018).
In this experiment, YCWP had no significant effect on the antibody production against SRBC, as compared to the control group (Table ). However, the heavier bursa of Fabricius and thymus weight in YCWP-fed birds indicated the improved immune system function. This might be due to a direct interaction between prebiotics and host GIT immune cells, as well as the indirect effects of prebiotics on the immune system by modulating the intestinal microbiota and increasing the beneficial bacteria (Janardhana et al. Citation2009). Microbiota such as Lactobacillus and Bifidobacterium support the animal's immune system against invasive pathogens by stimulating the GIT immune responses. Prebiotics indirectly strengthen the bird's immune system by stimulating the growth of these bacteria. Also, some YCWPs such as MOS, can interact directly with antigens and increase the immune signals in chickens (Teng and Kim Citation2018). Generally, prebiotics can strengthen the host immune system in several ways. Firstly, this can be through their effects on the lymphoid tissues of chickens, such as the caecal tonsil, spleen, thymus, and bursa of Fabricius (Table ). Secondly, this can be realised by enhancing the antibody titres of IgM and IgG in plasma and IgA level in the intestine. Thirdly, it could be due to the enhanced phagocytosis and proliferation of monocytes and macrophages. As the fourth way, increasing the expression of mucin genes in the gut can be noted. Finally, the GIT immune functions could be enhanced (Huang et al. Citation2015; Adhikari and Kim Citation2017). In the present study, the lymphatic organs were weighed at the end of the rearing period. The weight of the lymphatic organs can indicate the general condition and health of the birds. In chickens, the bursa of Fabricius and thymus can be regarded as the sites for T and B lymphocytes maturation; therefore, the size and weight of the bursa and thymus can provide important information about the growth and development of the immune system (Grasman Citation2002). Generally, when the bursa of Fabricius weight to body weight ratio is about 0.2%, it can be concluded that the bird has an immune system with a good performance and health (Sellaoui et al. Citation2012). In our study, the increase in the thymus and bursa weight could indicate the development of the bird's immune system due to the use of YCWP.
One of the positive effects of YCWP is increasing the production of short-chain fatty acids (specifically propionate, acetate and butyrate) in the caecum by stimulating the growth of gastrointestinal bacteria. However, in the present study, YCWP supplementation had no significant effect on the caecal concentration of SCFAs. According to Table , the YCWP could numerically increase the concentration of propionate in the caecum. In addition to the reasons mentioned earlier, the reduction of pathogenic bacteria in the intestine could be attributed to the decrease of pH due to the increased SCFAs in the intestine (Micciche et al. Citation2018; Kim et al. Citation2019).
This study showed that adding the YCWP to the diet increased the villus height and reduced the crypt depth (Table ). The effect of YCWP on intestinal morphology is probably due to its impact on the GIT microbiota. Some YCWPs, such as MOS, can improve the villi growth and structure in the small intestine and increase the activity of such jejunal enzymes as maltase, aminopeptidase, and alkaline phosphatase (Ricke et al. Citation2020). It has been shown that adding MOS to the broilers’ diets could increase Lactobacillus in the ileum and, consequently, the activity of digestive enzymes in the chickens. Clavijo and Flórez have also reported that the activity of digestive enzymes is affected by the intestinal microflora. For example, Bifidobacterium and Lactobacillus increase the activity of digestive enzymes such as proteases and lipases (Clavijo and Flórez Citation2018). Also, the higher levels of intestinal Lactobacillus in the birds supplemented with MOS resulted in a healthier GIT (Teng and Kim Citation2018). The intestinal microbiota balance and intestinal morphology, including the villus height, crypt depth and villus height to crypt depth ratio, are the critical indices of gut health and function. Previous studies have also reported that MOS supplementation increases the villus height and surface area, decreases the crypt depth, and up-regulates the gene expression of mucin genes related to mucin secretion (Yang et al. Citation2008; Baurhoo et al. Citation2009; Chee et al. Citation2010). Improved intestinal morphology in MOS-fed chickens may also be due to reducing the population of pathogenic bacteria in the GIT. For instance, some pathogenic bacteria, such as E.coli, can impair the digestive enzymes' secretion in two ways. Firstly, this is done by secreting the microbial proteolytic enzymes that degrade the host digestive enzymes; secondly, this occurs by damaging the intestinal villus and microvillus (Pan and Yu Citation2014).
Previous reports have also shown that inflammation induces the production of reactive oxygen species (ROS) in the mitochondria and, finally, in the cells. However, almost all cells produce modulators through the expression of genes, which regulate the production of inflammatory factors such as ROS. The uncoupling proteins (UCP) are mitochondrial transporters present in the inner membrane of mitochondria (Ledesma et al. Citation2002). Some subsets of UCP (UCP-2 and UCP-3) protect the cells against the oxidative damage of superoxide by the mild uncoupling (Brand et al. Citation2002, Citation2004). PGC-1α is the most dominant regulatory protein in mitochondrial biogenesis (Wu et al. Citation1999). PGC-1α stimulates the nuclear respiratory factor-1 and the mitochondrial transcription factor expression; these, in turn, can lead to up-regulating the expression of nuclear and mitochondrial genes that encode the mitochondrial proteins (Andersson and Scarpulla Citation2001; Nisoli et al. Citation2003). As illustrated in Figure , the dietary supplementation of broiler chickens with the prebiotic at 0.15 g/kg level resulted in the greatest PGC1-α transcription among birds. It has also been reported that the increased UCP could significantly down-regulate the ROS production following fasting (Abe et al. Citation2006). Also, acute heat stress induces mitochondrial superoxide production in the broiler skeletal muscle through the down-regulation of UCP (Mujahid et al. Citation2006). Besides, some previous studies have suggested that PPARγ, PGC-1α, and nitric oxide play an important role in mitochondrial biogenesis (Wu et al. Citation1999; Nisoli et al. Citation2003). Nisoli et al. (Citation2003) reported that NOS-/- mice had lower PGC-1α mRNA levels than the wild-type ones. It has also been shown that the exposure of chickens to a cold environment results in the prompt up-regulation of the PGC-1α expression (Ueda et al. Citation2005). Therefore, the up-regulation of PGC-1α may up-regulate such PGC-1α dependent factors as UCPs; these may, in turn, decrease oxidative stress and, at the next level, increase mitochondrial biogenesis.
The family of peroxisome proliferation-activated receptors includes three isoforms: PPARα, PPARβ, and PPARγ (Berger and Moller Citation2002). The PPARγ is expressed in white and brown adipose tissues and the large intestine. In the molecular epithet, PPARs are a family of ligand-activated nuclear hormone receptors (NRs) belonging to the steroid receptor superfamily. PPARs are nuclear receptor proteins involved in many regulatory functions (Desvergne and Wahli Citation1999). PPARγ is the dominant regulator of the expression of genes encoding proteins that control adipocyte differentiation and fatty acids uptake and metabolism (Kliewer et al. Citation1997; Rosen et al. Citation1999). In chickens, PPAR-γ plays a crucial role in fat deposition (Sato et al. Citation2004). It was reported that dietary supplementation of sphingomyelin reduces inflammation in the intestinal epithelial cells through the upregulation of PPARγ (Mazzei et al. Citation2011). many studies reported that PPARγ regulates a large number of activities such as glucose and lipid metabolism, cellular proliferation, differentiation, and inflammation (Auwerx Citation2002; Dubuquoy et al. Citation2006; Leonardini et al. Citation2009; Duszka et al. Citation2016). The PPARγ is highly expressed in the GIT; PPARγ is expressed in the duodenum and especially in the proximal colon (Mansen et al. Citation1996; Escher et al. Citation2001; Harmon et al. Citation2010). The PPARγ transcription and activity are stimulated in the GIT by numerous bacterial metabolites and bacterial by-products such as butyrate (Wachtershauser et al. Citation2000; Schwab et al. Citation2007), H2O2 (Voltan et al. Citation2008), and LPS (Dubuquoy et al. Citation2003). Expression of PGC-1α mRNA was not different among control, antibiotic group, and birds supplemented with high levels of the YCWP in the ileal tissue (Figure ). However, we observed a higher level of PPARγ mRNA in the birds fed the diet containing 0.15 and 0.3 g/kg YCWP, thus implying that a threshold of PPARγ transcription and finally, PPARγ protein expression would be needed for triggering the functional roles of PGC-1α. Also, as illustrated in Figure , in the birds fed with 0.45 and 0.6 g/kg YCWP, PPARγ and PGC-1α mRNA were the lowest; this might indicate the reduced inflammation in their intestinal cells. Besides, the greater PGC-1α mRNA in 0.15 g/kg YCWP -fed birds reflected the improved metabolism and biogenesis in their intestinal cells. On the other hand, one of the critical roles of PPARγ is to prevent lipotoxicity by different mechanisms and to regulate the targets relevant to inflammation (Clark Citation2002). When a YCWP with a specific function destroys the pathogenic bacteria and their pieces, the endothelial cells prefer to increase the homeostasis instead of activating the anti-inflammation cascade. A previous study showed that PPARγ agonists could increase anti-inflammatory factors such as adiponectin and reduce tissue necrosis factors (Krishnaswami et al. Citation2010). Researchers have investigated the effects of PPARγ activation on the intramuscular fat in the growing beef bulls, using the oral administration of 2, 4-thiazolidinedione (TZD) to activate PPARγ. They have shown that the oral administration of TZD did not affect the carcase quality, but it could decrease the expression of PPAR (α, -δ and, -γ) in the liver (Arevalo-Turrubiarte et al. Citation2012). Our results also demonstrated that feeding birds with the YCWP at the levels of 0.45 and 0.6 g/kg decreased the inflammation, indicating that the IL-10 and PPARγ expression were similar to control in their ileum; finally, improving metabolism in them (Janani and Kumari Citation2015). However, as displayed in and , with increasing the YCWP in the broilers diet, the histological changes of the jejunum were considerably better than those in the control and antibiotic groups. However, we demonstrated that in the lowest level of the YCWP, the PPARγ and PGC-1α expression was significantly increased. These all indicate that PPARγ and PGC-1α may be involved in the proliferation of the GIT and goblet cells in the crypt depth. Some researchers have also shown that PGC-1α is one of the critical factors in cell proliferation and differentiation (Zheng et al. Citation2016).
Toll-like receptors, also known as pathogen recognition receptors, are part of the innate immune system (Aderem and Ulevitch Citation2000). These receptors recognise the molecular patterns of pathogens called microbe-associated molecular patterns, initiate a chain reaction, and stimulate the immune system (Aderem and Ulevitch Citation2000). Chicken TLR2b4, and 21 are involved in recognising peptidoglycan, lipopolysaccharides, and unmethylated CpG oligonucleotides, respectively (Hoshino et al. Citation1999; Fukui et al. Citation2001; Keestra et al. Citation2010). Yitbarek et al. (Citation2013) showed the greater expression of TLR4, and TLR2b in the bird's spleen and bursa of Fabricius fed with yeast culture-supplementation diets (Yitbarek et al. Citation2013). They also demonstrated that the dietary supplementation with a yeast culture in chickens significantly increased the TLR21 transcription in their intestine.
However, in the current study, the lower concentration of the YCWP did not restrain the harmful bacteria (Coliform bacteria). But the higher inclusion levels (0.3, 0.45, and 0.6 g/kg of YCWP) resulted in the lower harmful bacteria and finally led to a reduction of TLR4 transcription and inflammation. The linkage between TLR4 and LPS could activate the cascade of the signalling pathway in the inflammation, subsequently increasing the anti-inflammatory cytokine expressions, such as IL-10, which are involved in the immune response and the intrinsic immune system (Chen et al. Citation2010). The IL-10 is an anti-inflammatory cytokine that acts as a potential factor in decreasing the inflammation and host pathology when diseases occur (Redpath et al. Citation2014). Dependent protozoan parasites activate IL-10 to diminish the pathogen-damaging host inflammatory responses. Arendt et al. also showed that the Eimeria challenge in broiler chickens increased the IL-10 gene expression (Arendt et al. Citation2019). Some studies have demonstrated that IL-10 acts as a macrophage-inactivating regulatory cytokine, suppressing the secretion of the pro-inflammatory cytokine and inhibiting the expression of the major histocompatibility class II (MHC II) and co-stimulatory molecules (O'Garra and Vieira Citation2007). In healthy chickens, the gene expression of IL-10 mRNA has been shown to be greater in the duodenum than in the caecum (Haritova and Stanilova Citation2012). Yitbarek et al. (Citation2013) also observed the up-regulation of the IL-10 gene only in the ileum of the birds receiving a yeast culture supplemented diet, which can be considered as a local inhibition of the Th1 pathway (Yitbarek et al. Citation2013). He et al. (Citation2012) have also suggested that the regulation of the macrophage activity by Th1/2 cytokines is crucial to maintain a balanced immunity, providing adequate Th1-mediated protective immunity and preventing the detrimental effects of severe inflammation (He et al. Citation2012). Therefore, these results indicated that dietary YCWP supplementation at the level of 0.6 g/kg considerably reduced the chronic inflammation in the broilers’ GIT; meanwhile, this led to the successful regulation of the metabolism and specifically, mitochondrial biogenesis. In other words, the inclusion of the YCWP in the broiler diets could modulate the local innate immunity in the broiler chickens. Besides, feeding YCWP-supplemented diets for a long period might have decreased the bacterial population, TLR4 responses, and inflammation.
Conclusions
The results demonstrated that dietary YCWP supplementation could positively affect the broilers’ gut, epithelial cell differentiation and turnover, and immunomodulation. Furthermore, the YCWP improved intestinal villi development, which could be due to the PPARγ gene expression and the increasing population of the beneficial bacteria, specifically Lactobacillus. Supplementation of YCWP regulated pro and anti-inflammatory cytokines via increment of IL-10 and reduction of TLR4 expression systemic pathways. Therefore, YCWP supplementation could have an immune stimulatory effect via the local and systemic responses involving TLRs and cytokines, with implications for enhancing the resistance to the pathogenic microorganisms. Furthermore, because the jejunum is a key organ in controlling the entry of bacteria and other pathogens entering into the GIT, the strong responses of the cytokines observed in the current study warrant further investigation into the role of YCWP in limiting the pathogens' invasion.
Ethical approval
The authors confirm that THEY were followed the animal welfare guide,as adopted by FASS (2010). All animal care and experimentalprocedures were approved by the animal policy and welfare committee of the Isfahan University of Technology.
Acknowledgements
We appreciate Pathway Intermediates Company, Seoul, South Korea, and the Isfahan University of Technology for supporting this article. We also appreciate Ali Sadeghi Sefidmazgi (Isfahan University of Technology, Isfahan, Iran) for his support in statistical analysis
Disclosure statement
No potential conflict of interest was reported by the author(s).
Additional information
Funding
References
- Abe T, Mujahid A, Sato K, Akiba Y, Toyomizu M. 2006. Possible role of avian uncoupling protein in down-regulating mitochondrial superoxide production in skeletal muscle of fasted chickens. FEBS Lett. 580(20):4815–4822.
- Aderem A, Ulevitch RJ. 2000. Toll-like receptors in the induction of the innate immune response. Nature. 406(6797):782–787.
- Adhikari PA, Kim WK. 2017. Overview of prebiotics and probiotics: focus on performance, gut health and immunity–a review. Ann Anim Sci. 17(4):949–966.
- Akhlaghi A, Zamiri MJ, Jafari Ahangari Y, Atashi H, Ansari Pirsaraei Z, Deldar H, Eghbalian AN, Akhlaghi AA, Navidshad B, Yussefi Kelarikolaei K, et al. 2013. Oral exposure of broiler breeder hens to extra thyroxine modulates early adaptive immune responses in progeny chicks. Poult Sci. 92(4):1040–1049.
- Andersson U, Scarpulla RC. 2001. Pgc-1-related coactivator, a novel, serum-inducible coactivator of nuclear respiratory factor 1-dependent transcription in mammalian cells. Mol Cell Biol. 21(11):3738–3749.
- Arendt M, Elissa J, Schmidt N, Michael E, Potter N, Cook M, Knoll LJ. 2019. Investigating the role of interleukin 10 on Eimeria intestinal pathogenesis in broiler chickens. Vet Immunol Immunopathol. 218:109934.
- Arevalo-Turrubiarte M, Gonzalez-Davalos L, Yabuta A, Garza JD, Davalos JL, Mora O, Shimada A. 2012. Effect of 2, 4-thiazolidinedione on limousin cattle growth and on muscle and adipose tissue metabolism. PPAR Res. 2012:891841.
- Arslan F, De Kleijn DP, Pasterkamp G. 2011. Innate immune signaling in cardiac ischemia. Nat Rev Cardiol. 8(5):292–300.
- Asgari F, Falak R, Teimourian S, Pourakbari B, Ebrahimnezhad S, Shekarabi M. 2018. Effects of oral probiotic feeding on toll-like receptor gene expression of the chicken’s cecal tonsil. Rep Biochem Mol Biol. 6(2):151–157.
- Auwerx J. 2002. Nuclear receptors. I. PPAR gamma in the gastrointestinal tract: gain or pain? Am J Physiol Gastrointest Liver Physiol. 282(4):G581–G585.
- Baghi M, Rostamian Delavar M, Yadegari E, Peymani M, Pozo D, Nasr-Esfahani MH, Ghaedi K. 2020. Modified level of miR-376a is associated with Parkinson's disease. J Cell Mol Med. 24(4):2622–2634.
- Baurhoo B, Ferket PR, Zhao X. 2009. Effects of diets containing different concentrations of mannanoligosaccharide or antibiotics on growth performance, intestinal development, cecal and litter microbial populations, and carcass parameters of broilers. Poult Sci. 88(11):2262–2272.
- Berger J, Moller DE. 2002. The mechanisms of action of PPARs. Annu Rev Med. 53:409–435.
- Brand MD, Affourtit C, Esteves TC, Green K, Lambert AJ, Miwa S, Pakay JL, Parker N. and 2004. Mitochondrial superoxide: production, biological effects, and activation of uncoupling proteins. Free Radic Biol Med. 37(6):755–767.
- Brand MD, Pamplona R, Portero-Otín M, Requena JR, Roebuck SJ, Buckingham JA, Clapham JC, Cadenas S. and 2002. Oxidative damage and phospholipid fatty acyl composition in skeletal muscle mitochondria from mice underexpressing or overexpressing uncoupling protein 3. Biochem J. 368(Pt 2):597–603.
- Chee SH, Iji PA, Choct M, Mikkelsen LL, Kocher A. 2010. Characterisation and response of intestinal microflora and mucins to manno-oligosaccharide and antibiotic supplementation in broiler chickens. Br Poult Sci. 51(3):368–380.
- Chen Y, Wermeling F, Sundqvist J, Jonsson AB, Tryggvason K, Pikkarainen T, and Karlsson MCI. 2010. A regulatory role for macrophage class A scavenger receptors in TLR4-mediated LPS responses. Eur J Immunol. 40(5):1451–1460.
- Clark RB. 2002. The role of PPARs in inflammation and immunity. J Leukoc Biol. 71(3):388–400.
- Clavijo V, Flórez MJV. 2018. The gastrointestinal microbiome and its association with the control of pathogens in broiler chicken production: a review. Poult Sci. 97(3):1006–10021.
- Desvergne B, Wahli W. 1999. Peroxisome proliferator-activated receptors: nuclear control of metabolism. Endocr Rev. 20(5):649–688.
- Dubuquoy L, Jansson EA, Deeb S, Rakotobe S, Karoui M, Colombel J-F, Auwerx J, Pettersson S, Desreumaux P. and 2003. Impaired expression of peroxisome proliferator-activated receptor gamma in ulcerative colitis. Gastroenterology. 124(5):1265–1276.
- Dubuquoy L, Rousseaux C, Thuru X, Peyrin‐Biroulet L, Romano O, Chavatte P, Chamaillard M, Desreumaux P. 2006. PPARgamma as a new therapeutic target in inflammatory bowel diseases. Gut. 55(9):1341–1349.
- Duszka K, Picard A, Ellero-Simatos S, Chen J, Defernez M, Paramalingam E, Pigram A, Vanoaica L, Canlet C, Parini P, et al. 2016. Intestinal PPARγ signalling is required for sympathetic nervous system activation in response to caloric restriction. Sci Rep. 6(1):36937.
- Ekim B, Calik A, Ceylan A, Saçakl P. 2020. Effects of Paenibacillus xylanexedens on growth performance, intestinal histomorphology, intestinal microflora, and immune response in broiler chickens challenged with Escherichia coli K88. Poult Sci. 99(1):214–223.
- El-Shall NA, Awad AM, El-Hack MEA, Naiel MEA, Othman SL, Allam AA, Sedeik ME. 2019. The simultaneous administration of a probiotic or prebiotic with live Salmonella vaccine improves growth performance and reduces fecal shedding of the bacterium in Salmonella-challenged broilers. Animals. 10(1):70.
- Escher P, Braissant O, Basu-Modak S, Michalik L, Wahli W, Desvergne B. 2001. Rat PPARs: quantitative analysis in adult rat tissues and regulation in fasting and refeeding. Endocrinology. 142(10):4195–4202.
- FASS, McGlone, J. 2010. Guide for the care and use of agricultural animals in agricultural research and teaching. 3rded. In: Federation of Animal Science Societies ISBN: 978-1-884706-11-0.
- Fernandez F, Hinton M, Gils BV. 2002. Dietary mannan-oligosaccharides and their effect on chicken caecal microflora in relation to Salmonella Enteritidis colonization. Avian Pathol. 31(1):49–58.
- Fukui A, Inoue N, Matsumoto M, Nomura M, Yamada K, Matsuda Y, Toyoshima K, Seya T. and 2001. Molecular cloning and functional characterization of chicken toll-like receptors A single chicken toll covers multiple molecular patterns. J Biol Chem. 276(50):47143–47149.
- Gadde U, Kim WH, Oh ST, Lillehoj HS. 2017. Alternatives to antibiotics for maximizing growth performance and feed efficiency in poultry: a review. Anim Health Res Rev. 18(1):26–45.
- Grasman KA. 2002. Assessing immunological function in toxicological studies of avian wildlife. Integr Comp Biol. 42(1):34–42.
- Haritova AM, Stanilova SA. 2012. Enhanced expression of IL-10 in contrast to IL-12B mRNA in poultry with experimental coccidiosis. Exp Parasitol. 132(3):378–382.
- Harmon GS, Dumlao DS, Ng DT, Barrett KE, Dennis EA, Dong H, Glass CK. 2010. Pharmacological correction of a defect in PPAR-gamma signaling ameliorates disease severity in Cftr-deficient mice. Nat Med. 16(3):313–318.
- He H, Genovese KJ, Swaggerty CL, MacKinnon KM, Kogut MH. 2012. Co-stimulation with TLR3 and TLR21 ligands synergistically up-regulates Th1-cytokine IFN-γ and regulatory cytokine IL-10 expression in chicken monocytes. Dev Comp Immunol. 36(4):756–760.
- Heni AC, Schmid J, Rasche A, Corman VM, Drosten C, Sommer S. 2020. Pathogen-associated selection on innate immunity genes (TLR4, TLR7) in a neotropical rodent in landscapes differing in anthropogenic disturbance. Heredity. 125(4):184–199.
- Hoshino K, Takeuchi O, Kawai T, Sanjo H, Ogawa T, Takeda Y, and, et al. 1999. Cutting edge: Toll-like receptor 4 (TLR4)-deficient mice are hyporesponsive to lipopolysaccharide: evidence for TLR4 as the Lps gene product. J Immunol. 162:3749–3752.
- Huang Q, Wei Y, Lv Y, Wang Y, Hu T. 2015. Effect of dietary inulin supplements on growth performance and intestinal immunological parameters of broiler chickens. Livest Sci. 180:172–176.
- Janani C, Kumari BDR. 2015. PPAR gamma gene-a review. Diabetes Metab Syndr. 9(1):46–50.
- Janardhana V, Broadway MM, Bruce MP, Lowenthal JW, Geier MS, Hughes RJ, Bean AGD. 2009. Prebiotics modulate immune responses in the gut-associated lymphoid tissue of chickens. J Nutr. 139(7):1404–1409.
- Keestra AM, de Zoete MR, Bouwman LI, van Putten JPM. 2010. Chicken TLR21 is an innate CpG DNA receptor distinct from mammalian TLR9. J Immunol. 185(1):460–467.
- Khan S, Roberts J, Wu SB. 2017. Reference gene selection for gene expression study in shell gland and spleen of laying hens challenged with infectious bronchitis virus. Sci Rep. 7(1):12.
- Kim GB, Seo YM, Kim CH, Paik IK. 2011. Effect of dietary prebiotic supplementation on the performance, intestinal microflora, and immune response of broilers. Poult Sci. 90(1):75–82.
- Kim S, Jang MJ, Kim SY, Yang Y, Pavlidis HO, Ricke CS. 2019. Potential for prebiotics as feed additives to limit foodborne Campylobacter establishment in the poultry gastrointestinal tract. Front Microbiol. 10(91):91.
- Kliewer SA, Sundseth SS, Jones SA, Brown PJ, Wisely GB, Koble CS, Devchand P, Wahli W, Willson TM, Lenhard JM, and, et al. 1997. Fatty acids and eicosanoids regulate gene expression through direct interactions with peroxisome proliferator-activated receptors alpha and gamma. Proc Natl Acad Sci USA. 94(9):4318–4323.
- Krishnaswami A, Ravi-Kumar S, Lewis JM. 2010. Thiazolidinediones: a 2010 perspective. Perm J. 14(3):64–72.
- Ledesma A, de Lacoba MG, Rial E. 2002. The mitochondrial uncoupling proteins. Genome Biol. 3(12):3015–3019.
- Lee R, Jung JS, Yeo J, Kwon HM, Park J. 2021. Analysis of immune response of chicken primary cells by infection with Korean IBV strain. Res Sq. :1–11.
- Leonardini A, Laviola L, Perrini S, Natalicchio A, Giorgino F. 2009. Cross-talk between PPARgamma and insulin signaling and modulation of insulin sensitivity. PPAR Res. 2009: 818945–818957.
- Li S, Li J, Liu Y, Li C, Zhang R, Bao J. 2020. Effects of intermittent mild cold stimulation on mRNA expression of immunoglobulins, cytokines, and toll-like receptors in the small intestine of broilers. Animals. 10(9):1492.
- Luo B, Shi H, Wang L, Shi Y, Wang C, Yang J, Wan Y, Niu J. and 2014. Rat lung response to PM2. 5 exposure under different cold stresses. IJERPH. 11(12):12915–12926.
- Mansen A, Guardiola-Diaz H, Rafter J, Branting C, Gustafsson JA. 1996. Expression of the peroxisome proliferator-activated receptor (PPAR) in the mouse colonic mucosa. Biochem Biophys Res Commun. 222(3):844–851.
- Mazzei JC, Zhou H, Brayfield BP, Hontecillas R, Bassaganya-Riera J, Schmelz EM. 2011. Suppression of intestinal inflammation and inflammation-driven colon cancer in mice by dietary sphingomyelin: importance of peroxisome proliferator-activated receptor γ expression. J Nutr Biochem. 22(12):1160–1171.
- Micciche AC, Foley SL, Pavlidis HO, McIntyre DR, Ricke SCA. 2018. review of prebiotics against Salmonella in poultry: current and future potential for microbiome research applications. Front Vet Sci. 5(191):191.
- Mujahid A, Sato K, Akiba Y, Toyomizu M. 2006. Acute heat stress stimulates mitochondrial superoxide production in broiler skeletal muscle, possibly via downregulation of uncoupling protein content. Poult Sci. 85(7):1259–1265.
- Napper S, Dadgar S, Arsenault RJ, Trost B, Scruten E, Kusalik A, Shand P. 2015. Induction of tissue- and stressor-specific kinomic responses in chickens exposed to hot and cold stresses. Poult Sci. 94(6):1333–1345.
- Nisoli E, Clementi E, Paolucci C, Cozzi V, Tonello C, Sciorati C, Bracale R, Valerio A, Francolini M, Moncada S, and, et al. 2003. Mitochondrial biogenesis in mammals: the role of endogenous nitric oxide. Science. 299(5608):896–899.
- O'Garra A, Vieira P. 2007. T(H)1 cells control themselves by producing interleukin-10. Nat Rev Immunol. 7(6):425–428.
- Ott CP, Omara II, Persia ME, Dalloul RA. 2018. The impact of β-glucans on performance and response of broiler chickens during a coccidiosis challenge. Poult Sci. 97(8):2713–2721.
- Pan D, Yu Z. 2014. Intestinal microbiome of poultry and its interaction with host and diet. Gut Microbes. 5(1):108–119.
- Paul A, Dangi SS, Gupta M, Singh J, Thakur N, Naskar S, Nanda PK, Mohanty N, Das AK, Bandopadhayay S, and, et al. 2015. Expression of TLR genes in Black Bengal goat (Capra hircus) during different seasons. Small Rumin Res. 124:17–23.
- Pourabedin M, Xu Z, Baurhoo B, Chevaux E, Zhao X. 2014. Effects of mannan oligosaccharide and virginiamycin on the cecal microbial community and intestinal morphology of chickens raised under suboptimal conditions. Can J Microbiol. 60(5):255–266.
- Pourabedin M, Zhao X. 2015. Prebiotics and gut microbiota in chickens. FEMS Microbiol Lett. 362(15): 122.
- Prakatur I, Miskulin M, Pavic M, Marjanovic K, Blazicevic V, Miskulin I, Domacinovic M. 2019. Intestinal morphology in broiler chickens supplemented with propolis and bee pollen. Animals. 9(6):301.
- Redpath SA, Fonseca NM, Perona‐Wright G. 2014. Protection and pathology during parasite infection: IL-10 strikes the balance. Parasite Immunol. 36(6):233–252.
- Ricke SC. 2018. Focus: nutrition and food science: impact of prebiotics on poultry production and food safety. Yale J Biol Med. 91(2): 151.
- Ricke SC, Lee SI, Kim SA, Park SH, Shi Z. 2020. Prebiotics and the poultry gastrointestinal tract microbiome. Poult Sci. 99(2):670–677.
- Ritzi MM, Abdelrahman W, Van-Heerden K, Mohnl M, Barrett NW, Dalloul RA. 2016. Combination of probiotics and coccidiosis vaccine enhances protection against an Eimeria challenge. Vet Res. 47(1):111.
- Rius-Pérez S, Torres-Cuevas I, Millán I, Ortega AL, Pérez S. 2020. PGC-1α, inflammation, and oxidative stress: an integrative view in metabolism. Oxid Med Cell Longev. 2020:1452696.
- Rosen ED, Sarraf P, Troy AE, Bradwin G, Moore K, Milstone DS, Spiegelman BM, Mortensen RM. 1999. PPAR gamma is required for the differentiation of adipose tissue in vivo and in vitro. Mol Cell. 4(4):611–617.
- Sakamoto K, Hirose H, Onizuka A, Hayashi M, Futamura N, Kawamura Y, Ezaki T. 2000. Quantitative study of changes in intestinal morphology and mucus gel on total parenteral nutrition in rats. J Surg Res. 94(2):99–106.
- SAS (Statistical Analysis System). 2009. SAS/STAT® 9.4. User's guide. Cary, North Carolina: SAS Institute Inc.
- Sato K, Fukao K, Seki Y, Akiba Y. 2004. Expression of the chicken peroxisome proliferator-activated receptor-gamma gene is influenced by aging, nutrition, and agonist administration. Poult Sci. 83(8):1342–1347.
- Schwab M, Reynders V, Loitsch S, Steinhilber D, Stein J, Schröder O. 2007. Involvement of different nuclear hormone receptors in butyrate-mediated inhibition of inducible NF kappa B signalling. Mol Immunol. 44(15):3625–3632.
- Seidavi A, Dadashbeiki M, Alimohammadi-Saraei M-H, van den Hoven R, Payan-Carreira R, Laudadio V, Tufarelli V. 2017. Effects of dietary inclusion level of a mixture of probiotic cultures and enzymes on broiler chickens immunity response. Environ Sci Pollut Res Int. 24(5):4637–4644.
- Sellaoui S, Alloui N, Mehenaoui S, Djaaba S. 2012. Evaluation of immune status of the chicken using morphometry and histology of the bursa of fabricius. J Vet Adv. 2:440–443.
- Sims MD, Dawson KA, Newman KE, Spring P, Hoogell D. 2004. Effects of dietary mannan oligosaccharide, bacitracin methylene disalicylate, or both on the live performance and intestinal microbiology of turkeys. Poult Sci. 83(7):1148–1154.
- Suresh G, Das RK, Kaur Brar S, Rouissi T, Avalos Ramirez A, Chorfi Y, Godbout S. 2018. Alternatives to antibiotics in poultry feed: molecular perspectives. Crit Rev Microbiol. 44(3):318–335.
- Takizawa H, Fritsch K, Kovtonyuk LV, Saito Y, Yakkala C, Jacobs K, Ahuja AK, Lopes M, Hausmann A, Hardt W-D, et al. 2020. Pathogen-induced TLR4-TRIF innate immune signaling in hematopoietic stem cells promotes proliferation but reduces competitive fitness. Cell Stem Cell. 27(1):177.
- Tarabees R, Gafar KM, EL-Sayed MS, Shehata AA, Ahmed M. 2019. Effects of dietary supplementation of probiotic mix and prebiotic on growth performance, cecal microbiota composition, and protection against Escherichia coli O78 in broiler chickens. Probiotics Antimicrob Proteins. 11(3):981–989.
- Teng PY, Kim WK. 2018. Roles of prebiotics in intestinal ecosystem of broilers. Front Vet Sci. 5(245):245.
- Ueda M, Watanabe K, Sato K, Akiba Y, Toyomizu M. 2005. Possible role for avPGC-1alpha in the control of expression of fiber type, along with avUCP and avANT mRNAs in the skeletal muscles of cold-exposed chickens. FEBS Lett. 579(1):11–17.
- Vermeulen AN, Schaap DC, Schetters TPM. 2001. Control of coccidiosis in chickens by vaccination. Vet Parasitol. 100(1–2):13–20.
- Voltan S, Martines D, Elli M, Brun P, Longo S, Porzionato A, Macchi V, D'Incà R, Scarpa M, Palù G, and, et al. 2008. Lactobacillus crispatus M247-derived H2O2 acts as a signal transducing molecule activating peroxisome proliferator activated receptor-gamma in the intestinal mucosa. Gastroenterology. 135(4):1216–1227.
- Wachtershauser A, Loitsch SM, Stein J. 2000. PPAR-gamma is selectively upregulated in Caco-2 cells by butyrate. Biochem Biophys Res Commun. 272(2):380–385.
- Wang X, Farnell YZ, Peebles ED, Kiess AS, Wamsley KGS, Zhai W. 2016. Effects of prebiotics, probiotics, and their combination on growth performance, small intestine morphology, and resident Lactobacillus of male broilers. Poult Sci. 95(6):1332–1340.
- Wang G, McConn BR, Liu D, Cline MA, Gilbert ER. 2017. The effects of dietary macronutrient composition on lipid metabolism-associated factor gene expression in the adipose tissue of chickens are influenced by fasting and refeeding. BMC Obes. 4:14.
- Wu Z, Puigserver P, Andersson U, Zhang C, Adelmant G, Mootha V, Troy A, Cinti S, Lowell B, Scarpulla RC, and, et al. 1999. Mechanisms controlling mitochondrial biogenesis and respiration through the thermogenic coactivator PGC-1. Cell. 98(1):115–124.
- Yang RB, Mark MR, Gray A, Huang A, Xie MH, Zhang M, Goddard A, Wood WI, Gurney AL, Godowski PJ, et al. 1998. Toll-like receptor-2 mediates lipopolysaccharide-induced cellular signalling. Nature. 395(6699):284–288.
- Yang Y, Iji PA, Kocher A, Mikkelsen LL, Choct M. 2008. Effects of mannanoligosaccharide and fructooligosaccharide on the response of broilers to pathogenic Escherichia coli challenge. Br Poult Sci. 49(5):550–559.
- Yitbarek A, Rodriguez-Lecompte JC, Echeverry HM, Munyaka P, Barjesteh N, Sharif S, Camelo-Jaimes G. 2013. Performance, histomorphology, and toll-like receptor, chemokine, and cytokine profile locally and systemically in broiler chickens fed diets supplemented with yeast-derived macromolecules. Poult Sci. 92(9):2299–2310.
- Zhang WF, Li DF, Lu WQ, Yi GF. 2003. Effects of isomalto-oligosaccharides on broiler performance and intestinal microflora. Poult Sci. 82(4):657–663.
- Zheng X, Boyer L, Jin M, Mertens J, Kim Y, Ma L, Ma L, Hamm M, Gage FH, Hunter T, and, et al. 2016. Fred H Gage, and Tony Hunter. Metabolic reprogramming during neuronal differentiation from aerobic glycolysis to neuronal oxidative phosphorylation. Elife. 5:e13374.
- Zoumpopoulou G, Kazou M, Alexandraki V, Angelopoulou A, Papadimitriou K, Pot B, Tsakalidou E. 2018. Probiotics and prebiotics: an overview on recent trends. In: Probiotics and prebiotics in animal health and food safety. Springer. P. 1–34.