Abstract
This study investigated the effect of different Bacillus spp. and Debaryomyces spp., which are screened from Melopsittacus undulates crop milk on growth performance, intestinal morphology, intestinal microflora and antioxidant abilities in broilers. By screening the Bacillus spp. and Debaryomyces spp. from parrot’s crop milk, the properties of these microbial were examined in vitro accordingly. In animal experiments, 480 1-day-old Ross 308 broilers were randomly divided into 6 treatments as control (corn-soybean meal), Bacillus velezensis with 5 × 106 cfu/kg feed (BV), Bacillus subtilis with 5 × 106 cfu/kg feed (BS), Debaryomyces hansenii with 5 × 106 cfu/kg feed (DH), B. velezensis + Saccharomyces cerevisiae with 5 × 106 cfu/kg feed (BV + SC), and B. velezensis + D. hansenii with 5 × 106 cfu/kg feed (BV + DH). The results showed that chickens in BV, BV + SC, and BV + DH groups showed significantly higher body weight and average weight gain than control group at 21 days of age. Biochemistry of triglyceride values in BV and BV + SC had significantly decreased than in control group. Intestinal lactic acid bacteria were significantly higher in BV and BS groups than in the control group in ileum. The villus height of jejunum in BV + SC and BV + DH was significantly higher than in the control group. Glutathione peroxidase activity was significantly higher in BV, BS, BV + SC groups than in the control group, but immune assays had no significant differences among the groups. Based on the results of animal growth performance, the number of lactic acids, intestinal morphology, antioxidant activity, and immune system, BV and BV + SC groups possess better feed additives for broiler chickens.
Bacillus spp. and Debaryomyces spp. affect on growth performance, intestinal morphology, intestinal microflora and antioxidant abilities in broiler chickens.
Broilers with feed additives of Bacillus velezensis, Debaryomyces hansenii, Saccharomyces cerevisiae have higher body weight and average weight gain
B. velezensis and B. velezensis + Saccharomyces cerevisiae groups possess better feed additives for broiler chickens.
HIGHLIGHTS
Introduction
There are about 80% of antibiotics in the world used in animals. As this phenomenon is likely to cause drug resistance (AI-Khalaifah Citation2018; Chang et al. Citation2020; Huang et al. Citation2021), more people pay attention to this issue (Lee et al. Citation2022). In 2006, the European Union (EU) began to prohibit the use of antibiotics in promoting animal growth. In 2017, the United States imposed restrictions on antibiotics, and in 2020, China began to ban the use of antibiotics in animal feed. Accordingly, the use of antibiotics has been reduced and replaced by beneficial feed additives like probiotics (Lutful-Kabir Citation2009). Probiotics are beneficial for improving feed intake, digestion and intestinal microflora, which could increase the health status and growth performance of chickens (Chang et al. Citation2019b; Chuang et al. Citation2021; Lee et al. Citation2022). Moreover, probiotics are regarded as natural sources to enhance broiler chickens’ antioxidant and immune capacity (Aluwong et al. Citation2013; Teng et al. Citation2017; Gong et al. Citation2018).
It is well-known that Bacillus spp. is resistant to gastric and intestinal juices because when Bacillus suffered from a poor environment, it would produce spores to protect itself, allowing Bacillus spp. to pass through gastric and intestinal juices to the host (Barbosa et al. Citation2005; Tsai et al. Citation2021; Lee et al. Citation2022). In addition, Bacillus spp. is capable of secreting a variety of enzymes that could improve feed digestion and nutrient absorption (Mingmongkolchai and Panbangred Citation2018).
Furthermore, Debaryomyces spp. have been reported to have potential probiotic properties (Kumura et al. Citation2004). It is approved by the European Food Safety Authority (EFSA) and included in their Qualified Presumption of Safety status list. Debaryomyces spp. DI 02 can be a potential probiotic candidate because it could safely pass through the gastrointestinal tract, adhere to Caco-2 cells, and enhance immune responses (Ochangco et al. Citation2016). The cell wall of Debaryomyces hansenii is composed of ß-glucan and mannan. These substances have the ability to enhance the immune system (Angulo et al. Citation2020). Thus, the strains of yeast D. hansenii are considered potential probiotic candidates for the poultry industry.
It is known that male and female parrots secrete the hormone prolactin, increasing the thickness of the inner wall of the crop, which will fall off to form the crop milk before the young bird hatches (Silver Citation1984). Crop milk is semi-solid, and its main component are proteins (60%). Therefore, crop milk is an important source necessary for the growth of young parrots. A previous study found that using crop milk to feed broilers, the body weight gain is higher than the control group at 7, 14, 21, 28 and 42 days (Pace et al. Citation1952). Therefore, it may have hypothesised that crop milk contains some probiotics which positively affected the growth of broilers.
However, fewer studies have screened probiotics from parrots’ crop milk. Hence, the purpose of this study is to screen potential Bacillus spp. and Debaryomyces spp. from parrots’ crop milk as a supplement in the broilers’ diet to investigate the growth performance, serum characteristics, intestinal microflora and antioxidant activity for broilers.” in the Introduction section.
Materials and methods
Bacillus spp. and Debaryomyces spp. culture conditions
All animals use protocols were followed in accordance with the Animal Care and Use Committee (IACUC: 107-042). The 22 strains of Bacillus spp. and the 4 strains of Debaryomyces spp. were screened from Melopsittacus undulates crop milk. Bacillus strains were cultured in LB broth (Neogen, Lansing, MI, USA) at 37 °C for 24 h, while Debaryomyces spp. was cultured in YM broth (Neogen, Lansing, MI, USA) at 28 °C for 48 h. Saccharomyces cerevisiae spp., a commercial probiotic, was used as a positive control. Comparison of sequencing by NCBI/Basic Local Alignment Search Tool (BLAST) after Bacillus strains and Debaryomyces spp. were identified by biochemistry, 16s rDNA and 18s rDNA gene sequencing (Table ).
Table 1. Bacillus spp. and Debaryomyces hansenii screening from Melopsittacus undulates crop milk of strain identification.
In vitro adherence ability
The adherence ability method was modified by Fuller (Citation1978). The fresh crop was taken from a chicken, and the contents of crop epithelium were cleaned with pH 7.2 sterilised phosphate-buffered saline (PBS). Then, the epithelial cells were scraped with sterilised slide glass and dissolved in PBS. The 100 μL of epithelium cells were mixed with 5 × 106 cfu/mL of Bacillus spp. and Debaryomyces spp. (100 μL), respectively. Then, they were separately cultured at 37 °C and 28 °C, shaken by a water tank for 30 min. After 30 min, the sample was placed into a microcentrifuge (Labnet, spectrafugeTM 24D Edison, NJ, USA) at 3000 × g for 5 min. The supernatant was discarded, and the pellet was resuspended in 100 μL sterilised PBS. Subsequently, the bacteria were observed with a microscope camera (OPTI, item EI600 HD camera) after a small amount of mixed solution was taken on the slide glass and stained with gram stain and methyl blue.
Gastric and intestinal fluid tolerances
The Bacillus and Debaryomyces spp. were incubated in the LB broth and YM broth for 1 day and 2 days, respectively. After cultivating, the bacterial liquid with concentration of 5 × 106 cfu/mL was centrifuged at 7500 × g for 10 min, and the supernatant was removed. The method for gastric and intestinal fluid tolerance was modified by Guo et al. (Citation2006). For gastric tolerance, samples were added with 1 mL PBS (pH 2.0, containing 0.3% pepsin (Merck, CAS NO. 9001-75-6, Darmstadt, Germany). For intestinal fluid tolerance, on the other hand, samples were added with 1 mL PBS (pH 8.0, containing 0.3% bile bovine (Sigma, CAS NO. 8008-63-7, ST. Louis, USA) and 1 mg/mL trypsin. Then, the samples were incubated at 37 °C. Surviving microorganisms were plated in LB and YM agars, and the count was expressed in colony-forming units (CFU) per millilitre.
Enzyme activity assay
The protease, cellulase and mannanase activities of Bacillus spp. (U/mL) methods were modified by previous study (Raut et al. Citation2012; Yopi et al. Citation2017; Abu-Gharbia et al. Citation2018). Protease activity was measured using substrate 0.3 mL of 1% (w/v) casein (dissolved in 20 mM Tris-HCl buffer, pH 7.5) as one unit of protease is defined as the amount of enzyme that hydrolyses casein (Sigma, CAS NO. 9005-46-3, ST. Louis, USA) to produce equivalent absorbance to 1 μmol of tyrosine/min with tyrosine (Alfa Aesar, CAS NO. 60-18-4, Massachusetts, USA) as standard. Cellulase activity was measured using substrate 5 mg/mL of CMC (Sigma, CAS NO. 9004-32-4, ST. Louis, USA) as one unit of cellulase activity is defined as the amount of enzyme that releases 1 μmol of d-glucose per minute under 37 °C pH 5.5. Mannanase activity was measured using substrate of 6 mg/mL Locust Bean Gum (Sigma, CAS NO. 9000-40-2, ST. Louis, USA) which was dissolved in 50 mM of sodium phosphate buffer, pH 5.5 as one unit of mannanase activity is defined as the amount of enzyme that liberates 1 μmol of d-mannose per minute under 37 °C pH 5.5. Meanwhile, the methods for xylanase, cellulase, mannanase, ß-glucanase and α-galactosidase activities of D. hansenii were modified by previous study (Vink et al. Citation2004; Carrasco et al. Citation2016; Yopi et al. Citation2017; Liu et al. Citation2018; Cao et al. Citation2019). Xylanase activity was measured using 250 μL of the substrate solution of 10 mg/mL xylan (Sigma, CAS NO. 9014-63-5, ST. Louis, USA) as one unit of xylanase activity is defined as the amount of enzyme capability in releasing 1 μmol of xylose per minute under 30 °C pH 5.0. One unit of ß-glucanase activity is defined as the amount of enzyme required to release one µmole of glucose-reducing-sugar equivalents per minute in the presence of ß-glucan (8 mg/mL) (Sigma, CAS NO. 9008-22-4, ST. Louis, USA) in sodium acetate buffer (100 mM), pH 5.0 at 50 °C. One activity unit (U) of α-galactosidase was defined as the amount of enzyme that releases one μmol of p-nitrophenol from 10 mM p-nitrophenyl-α-d-galactopyranoside (Sigma, CAS NO. 3767-28-0, ST. Louis, USA) per minute under 40 °C pH 4.0.
Animal experiments
A total of 480 1-day-old male and female polyculture Ross 308 broiler chickens were randomly assigned for 35 days to 6 groups, namely the control group (fed with corn-soybean meal), BV group (basal diets with 5 × 106 cfu/kg feed Bacillus velezensis), BS group (basal diet with 5 × 106 cfu/kg feed Bacillus subtilis), DH group (basal diet with 5 × 106 cfu/kg feed D. hansenii), BV + DH group (basal diet with 5 × 106 cfu/kg feed B. velezensis and D. hansenii), BV + SC group (basal diet with 5 × 106 cfu/kg feed B. velezensis and S. cerevisiae). All the bacteria added into the diet is in powder form. There were 20 chickens per pen and 4 replicates per treatment. During the test period, the feed and drinking were ad libitum. Diets were divided into starter and finisher (Table ). The average body weight (47.1 ± 0.60 g/bird) was initially similar among the groups. Both were formulated to meet the nutrients of broilers (NRC, 1994). The body weights of the broiler were measured at 21 and 35 days of age to calculate the broiler’s body weight, average weight gain, average feed consumption and feed conversion ratio (FCR).
Table 2. Ingredients and compositions of the experimental diets for broiler chickens.1
Serum characteristics
At 5th weeks of the experiment, blood samples were randomly collected from broilers in each treatment group. The samples were collected into a blood collection vessel (about 5 mL), placed at 4 °C for 3–4 h to precipitate the blood. Then, it was centrifuged at 3000 × g at 4 °C for 10 min to extract the serum for analysis. Each treatment 4 replicates (each replicate 2 birds, totally 8 birds for half male and female) was measured by an automatic biochemical analyzer (Hitachi, 7150 auto-analyzer, Tokyo, Japan). The enzymes were measured by different commercial kits are as follows, Superoxide dismutase (Cayman, No.706002, USA), Glutathione peroxidase (Cayman, No.703102, USA), Catalase (Cayman, No. 707002, USA), Interleukin-6 (Fine Test, ECH0046, China), Interleukin-10 (Fine Test, No. ECH0033, USA) and Tumour necrosis factor alpha (Cusabio, No.CSB-E11231Ch, China). All methods and protocols provided by the manufacturer were followed.
Intestinal morphology
At 5 weeks, a total of 30 broiler chickens were randomly taken from 6 treatments and fasted for 1 day. The broiler chickens were euthanized, and the middle of the jejunum and ileum were excised in three sections. They were soaked in neutral formalin for 3 days, and each sample was embedded by paraffin and stained with haematoxylin and eosin. Furthermore, the slice was observed with a light microscope. The samples were measured 60 spots of villus height and crypt depth by drawing a program analysis system (Capture 2.1).
Intestinal microbial population
At 5 weeks, a total of 24 broiler chickens were randomly taken from 6 treatments (each treatment has 4 broiler chickens) to analyse a total of lactic acid bacteria and coliform counts in the ileum and caecum. The broiler chickens were euthanized, 0.1 g of digesta were taken from the ileum and caecum, and mixed with 0.9 mL sterilised PBS for serial dilution. The number of lactic acid bacteria and coliform were calculated using Lactobacilli MRS agar and CHRO MagarTM ECC as the selected media.
Statistical analysis
Collected data were analysed for significance by ANOVA using the GLM procedure in the SAS software (SAS® 9.4, 2018, SAS Institute Inc., Cary, NC, USA). Differences between the treatment means were analysed using Duncan’s multiple range test, where p < .05 was considered significantly different.
Results
In vitro adhesion of probiotics to epithelial cells
The results of different probiotics adhering to crop epithelial cells are shown in Figure . Four different strains of probiotics were used in the adhering assay, namely B. velezensis, B. subtilis, S. cerevisiae and D. hansenii. Based on the pictures, the number of bacteria adhering to the crop epithelial cell was more than on the outside. Thus, all the probiotics had adhered to the crop epithelial ability.
Figure 1. Photo of broiler crop epithelial cell (A), crop epithelial cell (B1), Bacillus velezensis (B2), Bacillus velezensis adhering on the epithelial (C1), Bacillus subtilis (C2), Bacillus subtilis adhering on the epithelial (D1), Saccharomyces cerevisiae (D2), Saccharomyces cerevisiae adhering on the epithelial cell (E1), Debaryomyces hansenii (E2), Debaryomyces hansenii adhering on the epithelial cell.
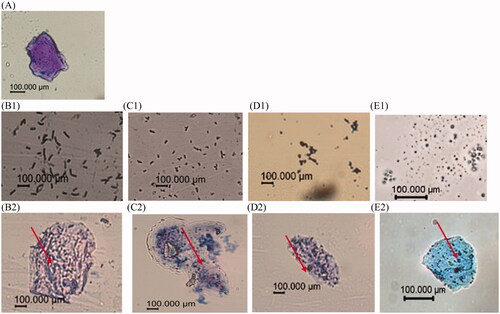
Stimulated gastric fluid tolerance assay
The gastrointestinal fluid tolerance of Bacillus spp. and Saccharomyces spp. are shown in Figure . (A) showed that 4 different D. hansenii screened from crop milk decreased within 1 log CFU/mL after 2 h and 4 h of pH 2.0 with 0.3% pepsin-stimulated gastric fluid; hence the ability of all D. hansenii to tolerate gastric juice. On the other hand, (B) showed that the number of 22 Bacillus strains decreased within 1 log cfu/mL after 3 h of pH 2.0 and 0.3% pepsin-stimulated juice, implying that the different Bacillus spp. screened from crop milk had the ability to tolerate stimulated juice.
Figure 2. Tolerance test of Bacillus strains and Saccharomyces strains. (A) Gastric juice (pH 2.0) tolerance of Debaryomyces hansenii strains and Saccharomyces cerevisiae strain at 0 h, 2 h and 4 h. (B) Gastric juice tolerance (pH 2.0) of Bacillus strains at 0 h and 3 h. (C) Bile salt (pH 8.0) tolerance of Debaryomyces hansenii strains and Saccharomyces cerevisiae strain at 0 h,2 h and 4 h (D) Bile salt (pH 8.0) tolerance of Bacillus strains at 0 h and 3 h. Each value represents the mean ± S.D. of three replicates (n = 3).
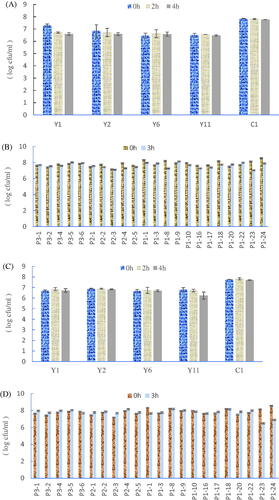
Stimulated small intestinal fluid tolerance assay
The small intestinal fluid tolerance of Bacillus spp. and Saccharomyces spp. are shown in Figure . Five different D. hansenii strains decreased within 1 log cfu/mL after 2 h and 4 h of pH 8.0, 0.3% bile salts (w/v) with 1 mg/mL trypsin-stimulated juice, thereby demonstrating all five strains’ ability to tolerate small intestinal fluid. Figure shows Bacillus P1-23. P1-24 strains decreased over 1 log cfu/mL after stimulating small intestinal fluid, indicating that Bacillus P1-23 and P1-24 could not tolerate such a stimulating environment. However, other strains of Bacillus spp. remained at least 7 log cfu/mL after the stimulation of the intestinal fluid environment.
Enzyme activity assay
Table shows the protease, cellulase and mannanase activities of the 22 Bacillus spp. The protease activities of P3-2 and P3-5 had the highest activity—25.9 U/mL and 25.5 U/mL, respectively. Meanwhile, the cellulase activities of P1-8 and P2-4 had the highest activity—respectively, 0.138 U/mL and 0.153 U/mL. In the mannanase analysis, P1-8 and P2-4 had the highest mannanase activities, with 0.388 U/mL and 0.445 U/mL, respectively.
Table 3.1. The protease, cellulase, mannanase activities of Bacillus spp.
Table 3.2. The xylanase, cellulase, mannanase, ß-glucanase, α-galactosidase activities of Debaryomyces hansenii (U/mL).
Table shows the activities of cellulase, xylanase, β-glucanase, mannanase and α-galactosidase of the strains of D. hansenii and Saccharomyces spp. In the cellulase test, five strains had similar 0.20 U/mL activity. The highest activity of xylanase, β-glucanase, mannanase and α-galactosidase were YD2, YD1, YD11 and C1 strains, respectively.
Animal performances
The results showed five different probiotic formulas (5 × 106 cfu/mL) supplemented with basal diets of broiler chicken in Table . At 1–21 days, in terms of the body weight of the chicken, BV, BV + SC, and BV + DH groups were significantly higher than the control group (p < .05). The body weight gain significantly increased in the BV + SC and BV + DH groups (p < .05). However, all treatments had no significant differences during 22–35 days and 1–35 days.
Table 4. The effects of Bacillus spp. and yeast supplemented in diets on growth performance of 1-35d broiler chickens.
Serum characteristics
The results of serum characteristics data are shown in Table . The analysis of GLU, BUN, CREA, UA, CHOL, HDL-C, LDL-C, SGOT and SGPT showed no significant differences among the groups. Meanwhile, the TG value was significantly decreased in the BV, BV + SC, and DH groups (p < .05). The CAT and SOD activities had no significant differences among the groups. However, the GPx activity significantly increased in BV, BS and BV + SC groups than in the control group (p < .05).
Table 5. The effect of Bacillus spp. and yeast supplemented in diets on serum characteristic of 35d broiler chickens.1
Intestinal morphology
Table shows the results of the five different probiotic formulas supplemented with basal diets of broiler chickens. In terms of the jejunum villus height, BV + SC and BV + DH significantly increased, and the BV group significantly decreased in crypt depth than the control group (p < .05). In terms of the ileum villus height, the BV + SC group had the highest villus height among the groups, and the BV + DH group had significantly lower crypt depth than the control group (p < .05). Meanwhile, the villus/crypt ratio had no significant differences among the groups in the jejunum and ileum.
Table 6. The effect of Bacillus spp. and yeast supplemented in diets on intestinal morphology of 35 d broiler chickens1.
Microbial population in ileum and caecum
Table shows the effect of feed supplement with Bacillus spp., D. hansenii and Saccharomyces spp. on the gut microflora. BV and BS groups increased significantly the lactic acid bacteria compared to the control group in the ileum, whereas BV, BS and DH groups had significantly higher lactic acid bacteria than the control group in the caecum (p < .05). However, coliform had no significant differences among the groups (p > .05).
Table 7. The effect of Bacillus spp. and yeast supplemented in diets on intestinal microflora concentration of 35d broiler chickens.
Discussion
The use of antibiotics for growth promotion has been prohibited by the European Union. Therefore, probiotics play an important role in replacing antibiotics. FAO/WHO (2002) defined probiotics as live microorganisms, which could benefit the host. Fuller (Citation1989) listed the basic characteristic of probiotics as (1) it should be a strain that could bring positive effects to the host; (2) it should be non-pathogenic and non-toxic; (3) it should survive in the low pH and bile salts environment. Accordingly, if probiotics pass through the gastrointestinal tract, they would have the ability to tolerate gastric juice and small intestinal fluid (Lee et al. Citation2022). In vitro test showed that 22 strains of Bacillus spp. and 4 different D. hansenii screened from M. undulates (Table ) could survive in gastric and intestinal fluids because the Bacillus spp. and Debaryomyces spp. counts only decreased within 1 log cfu/mL after culturing in the gastric- and intestinal-simulated environment except for P1-23 and P1-24 (Figure ). Gotcheva et al. (Citation2002) reported that yeast could pass through the gastrointestinal tract. Furthermore, Algburi et al. (Citation2016) showed B. subtilis KATMIRA1933 exposed to the acid environment (range from pH 2.0 to pH 3.0) and bile salts test for 4 h. In the study, the survival rates of B. subtilis KATMIRA1933 remain changeless. The results showed similar findings in our study.
Furthermore, probiotics should have the ability to adhere to intestinal epithelial cells because they can compete for the binding site with pathogens (Dunne et al. Citation2011; Teng et al. 2017; Lee et al. Citation2022). As mentioned previously, probiotics could inhibit the number of pathogens in the host’s intestine and maintain the intestinal microflora ecology balance (Dunne et al. Citation2011; Chang et al. Citation2019a; Chang et al. Citation2020). Both et al. (Citation2010) pointed out that the adhesion ability results depend on the number of adhered bacterial cells on the epithelial cell. According to the in vitro assay with different strains in our study, Bacillus P3-5, P2-4, D. hansenii Y1, and S. cerevisiae (control group for business) were selected to adhere to the epithelial cell assay. The results showed that 4 different strains have adhesion to the epithelial ability because the number of bacteria on the epithelial cells is more than on the outside (Figure ).
In the enzyme test, Bacillus P3-2 and P3-5 had higher protease activity among other groups − 25.9 U/mL and 25.5 U/mL, respectively. On the other hand, Bacillus spp. had the highest protease activity among other enzymes (Tables ). Chu (Citation2007) pointed out that strain Bacillus APP1 with the highest-yield alkaline proteases improves the high protein contents of feed digestion and enhances broiler’s absorption. Since protease can break protein’s peptide bonds and digest dietary proteins into small molecules, the host can easily absorb dietary nutrients. Additionally, Debaryomyces spp. could secrete enzymes, such as cellulase, xylanase, mannanase, ß-glucanase and α-galactosidase; 4 different Debaryomyces spp. had similar results. Park et al. (Citation2020) reported that multi-enzyme, including xylanase, ß-glucanase, protease, and amylase, could improve the host’s growth performance and digestibility.
Angulo et al. (Citation2020) reported that yeast D. hansenii has been considered an excellent probiotic in animals because D. hansenii could improve the growth of fish, enhance digestibility and maintain the intestinal population balance. Bai et al. (Citation2017) showed that diets supplemented with Bacillus spp. could increase the average daily gain, improve the feed conversion ratio (FCR) of broiler chickens by reducing the feed intake, and enhance antioxidant activities and immune responses. Above all, D. hansenii and Bacillus spp. are the potential probiotics nowadays, which are beneficial for the growth of broiler chickens. In the current study, BV, BV + SC, BV + DH supplemented in broiler chickens’ diets significantly increased the chickens’ body weight and weight gain at 21 days of age (Table ). Manafi et al. (Citation2018) showed that adding Bacillus spp. and Debaryomyces spp. in the feed could improve the broilers’ growth performance. Furthermore, Chen et al. (Citation2009) demonstrated that B. subtilis var. natto combined with S. cerevisiae had resulted in a higher broiler chickens body weight, body weight gain, and feed intake than the control group at 1–21 and 21–39 days.
The serum characteristic of 35d broiler chickens showed that BV, DH and BV + SC groups had a lower value than the control group (129, 155 and 118 vs. 198 mg/dL) (Table ). Rajput et al. (Citation2013) reported that Saccharomyces boulardii and B. subtilis decreased TG content in broilers. Ahmadi et al. (Citation2017) reported that probiotics could decrease serum triglycerides and VLDL-cholesterol because they can produce short fatty acids, which can suppress the synthesis of fatty acids and decrease the triglycerides secretin rate. The antioxidant assay and glutathione peroxidase (GPx) activity significantly increased in BV, BS, and BV + SC groups than the control group (134.7, 136.7 vs. 155.6 nmol/min/mL) (Table ). GPx is a cytosolic enzyme that catalyses hydrogen peroxide to water and oxygen. Rajput et al. (Citation2013) pointed out that B. subtilis can increase the serum antioxidant enzyme, which could support host scavenging free radicals and maintaining healthy states. Excessive oxidative stress will cause DNA hydroxylation, protein denaturation, and lipid oxidation on the host. Wang et al. (Citation2017) showed that probiotics might modulate the redox status of the host via metal ion chelating and antioxidant enzymatic system and stimulate the Nrf2-keap1-ARE pathway, which can stimulate phase II detoxifying antioxidant enzymes, such as haem oxygenase-1 (HO-1) and GPx. Thus, the supplementation of BV, BS, and BV + SC probiotics to the feed could help balance the oxidative stress for broilers.
In this study, BV + SC and BV + DH groups had higher villus height, and the BV group had lower crypt depth in the jejunum. The BV + SC group had higher villus height in the ileum, and BV + DH groups had lower crypt depth than the control group (Table ). The results indicated that BV + SC and BV + DH groups might influence the improvement of nutrient absorption because increasing villus height could expand the villus surface area, which affects the host’s nutrient absorptivity. Manafi et al. (Citation2018) pointed out that Bacillus spp. and yeast supplements could increase villus height and decrease crypt depth. Decreasing crypt depth can reduce energy consumption because animals can spend less energy to form crypt depth (Manafi et al. Citation2018).
BV and BS groups significantly increased the total lactic acid bacteria in the ileum. However, BV, BS, and DH groups increased significantly the total lactic acid bacteria in the caecum (Table ). Giang et al. (Citation2011) pointed out that Bacillus spp. and yeast can enhance the number of lactic acid bacteria counts in the intestinal. Phuoc and Jamikorn (Citation2016) also demonstrated that probiotics could enhance the organic acid concentration in the intestine, which is expected to reduce pH. Furthermore, a lower pH value could inhibit the pathogens, allowing probiotics to enhance intestinal health.
Conclusions
The Bacillus spp. and Debaryomyces spp. from M. undulates’s crop milk were screened, whose properties were examined in vitro accordingly. The in vitro test shows that the best B. velezensis, B. subtilis, and D. hansenii supplements in the broiler feed additive were selected. In terms of the broilers’ growth performance, BV, BV + SC, and BV + DH groups significantly increased the broilers’ body weight and body weight gain at 21 days of age. BV and BS treatments can increase the lactic acid bacteria in the ileum and caecum. In intestinal morphology, BV + SC group significantly increased the villus height in the jejunum and ileum. The BV group significantly decreased the crypt depth in the jejunum, whereas the BV + DH group significantly decreased in the ileum. BV, BS, and BV + SC groups significantly enhanced the GPx activity than the control group. According to the results, BV and BV + SC treatments possess potential the probiotic feed additives for broilers.
Author contributions
Conceptualisation, C. L. Liu and T. T. Lee; materials analysis, C. L. Liu and Y. R. Shih; methodology, C. L. Liu and P. C. Tang; supervision, T. T. Lee; investigation, C. L. Liu and T. T. Lee; writing—original draft preparation, C. L. Liu and T. T. Lee; writing—review and editing, C. L. Liu, L. J. Lin and T. T. Lee.
Disclosure statement
No potential conflict of interest was reported by the authors.
Additional information
Funding
References
- Abu-Gharbia MA, El-Sawy NM, Nasr AM, Zedan LA. 2018. Isolation optimization and characterization of cellulases and hemicellulases from Bacillus cereus LAZ 518 isolated from cow dung using corn cobs as lignocellulosic waste. J Pharm Appl Chem. 4(2):67–79.
- Ahmadi S, Jamilian M, Karamali M, Ebrahimi MT, Hafari P. 2017. Probiotic supplementation and the effects on weight loss, glycaemia and lipid profiles in women with polycystic ovary syndrome: a randomized, double-blind, placebo-controlled trial. Hum Reprod. 20:254–261.
- Algburi A, Volski A, Cugini C, Walsh EM, Chistyakov VA, Mazanko MS, Bren AB, Dicks LMT, Chikindas ML. 2016. Safety properties and probiotic potential of Bacillus subtilis KATMIRA1933 and Bacillus amyloliquefaciens B-1895. AiM. 06(06):432–452.
- Al-Khalaifah HS. 2018. Benefits of probiotics and/or prebiotics for antibiotic-reduced poultry: A literature review. Poult Sci. 97:3807–3815.
- Aluwong T, Kawu M, Raji M, Dzenda T, Govwang F, Sinkalu V, Ayo J. 2013. Effect of yeast probiotic on growth, antioxidant enzyme activities and malondialdehyde concentration of broiler chicken. Antioxidants. 2(4):326–339.
- Angulo M, Becerril MR, Cordova NM, Ramirez D, Angulo C. 2020. Probiotic and nutritional effects of Debaryomyces hansenii on animals: A literature review. Appl Microbiol Biotechnol. 104(18):7689–7699.
- Bai K, Huang Q, Zhang J, He J, Zhang L, Wang T. 2017. Supplemental effects of probiotic Bacillus subtilis fmbJ on growth performance, antioxidant capacity, and meat quality of broiler chickens. Poult Sci. 96(1):74–82.
- Barbosa TM, Serra CR, La-Ragione RM, Woodward MJ, Henriques AO. 2005. Screening for Bacillus isolates in the broiler gastrointestinal tract. Appl Environ Microbiol. 71(2):968–978.
- Both E, Gyorgy E, Kibedi-Szabo CZ, Tamas E, Abraham B, Miklossy I, Lanyi S. 2010. Acid and bile tolerance, adhesion to epithelial cells of probiotic microorganisms. UPB Sci Bull. 72:38–44.
- Cao MA, Cerdan ME, Sisio MG, Becerra M. 2019. Optimization of Saccharomyces cerevisiae α galactosidase production and application in the degradation of raffinose family oligosaccharides. Microb Cell Factories. 18:1–9.
- Carrasco M, Villarreal P, Barahona S, Alcaino J, Cifuentes V, Baeza M. 2016. Screening and characterization of amylase and cellulase activities in psychrotolerant yeasts. BMC Microbiol. 16(1):1–9.
- Chang CH, Teng PY, Lee TT, Yu B. 2019a. Effects of multi-strain probiotics combined with Gardeniae fructus on intestinal bacterial microbiota in broilers. Jpn Poult Sci. 56(1):32–43.
- Chang CH, Teng PY, Lee TT, Yu B. 2019b. The effects of the supplementation of multi-strain probiotics on intestinal microbiota, metabolites and inflammation of young SPF chickens challenged with Salmonella enterica subsp. enterica. Anim Sci J. 90(6):737–746.
- Chang CH, Teng PY, Lee TT, Yu B. 2020. Effects of multi-strain probiotic supplementation on intestinal microbiota, tight junctions, and inflammation in young broiler chickens challenged with Salmonella enterica subsp. enterica. Asian-Aust. J Anim Sci. 33(11):1797–1808.
- Chen KL, Kho WL, You SH, Yeh RH, Tang SW, Hsieh CW. 2009. Effects of Bacillus subtilis var. natto and Saccharomyces cerevisiae mixed fermented feed on the enhanced growth performance of broilers. Poult Sci. 91:978–985.
- Chu WH. 2007. Optimization of extracellular alkaline protease production from species of Bacillus. J Microbiol Biotechnol. 34:241–245.
- Chuang WY, Hsieh YC, Lin LJ, Chang SC, Lee TT. 2021. Effects of Saccharomyces cerevisiae and phytase co-fermentation of wheat bran on growth, antioxidation, immunity and intestinal morphology in broilers . Anim Biosci. 34(7):1157–1168.
- Dunne C, Mahony LO, Murphy L, Thornton G, Morrissey D, Halloran SO, Feeney M, Flynn S, Fitzgerald G, Daly C, et al. 2011. In vitro selection criteria for probiotic bacteria of human origin: correlation with in vivo findings. Am J Clin Nutr. 73:386–392.
- Fuller R. 1978. Epithelial attachment and other factors controlling the colonization of the intestine of the gnotobiotic chicken by Lactobacilli. J Appl Microbiol. 34:389–395.
- Fuller R. 1989. Probiotics in man and animals. J Appl Bacteriol. 66(5):365–378.
- Giang HH, Viet TQ, Ogle B, Lindberg JE. 2011. Effects of supplementation of probiotics on the performance, nutrient digestibility and faecal microflora in growing-finishing Pigs. Asian Australas J Anim Sci. 24(5):655–661.
- Gong L, Wang B, Mei X, Xu H, Qin Y, Li W, Zhou Y. 2018. Effects of three probiotic Bacillus on growth performance, digestive enzyme activities, antioxidative capacity, serum immunity, and biochemical parameters in broilers. Anim Sci J. 89(11):1561–1571.
- Gotcheva V, Hristozova E, Hristozova T, Guo M, Roshkova Z, Angelov A. 2002. Assessment of potential probiotic properties of lactic acid bacteria and yeast strains. Food Biotechnol. 16(3):211–225.
- Guo X, Li D, Lu W, Piao X, Chen X. 2006. Screening of Bacillus strains as potential probiotics and subsequent confirmation of the in vivo effectiveness of Bacillus subtilis MA139 in pigs. Antonie Van Leeuwenhoek. 90(2):139–146.
- Huang CM, Chuang WY, Lin WC, Lin LJ, Chang SC, Lee TT. 2021. Production performances and antioxidant activities of laying hens fed Aspergillus oryzae and phytase co-fermented wheat bran. Anim Biosci. 34(3):371–384.
- Kumura H, Tanoue Y, Tsukahara M, Tanaka T, Shimazaki K. 2004. Screening of dairy yeast strains for probiotic applications. J Dairy Sci. 87(12):4050–4056.
- Lee TT, Chou CH, Wang CL, Lu HY, Yang WY. 2022. Bacillus amyloliquefaciens and Saccharomyces cerevisiae feed supplements improve growth performance and gut mucosal architecture with modulations on cecal microbiota in red-feathered native chickens. Anim Biosci. (in press) doi: 10.5713/ab.21.0318
- Liu J, Basit A, Miao T, Zheng F, Yu H, Wang Y, Jiang W, Cao Y. 2018. Secretory expression of β-mannanase in Saccharomyces cerevisiae and its high efficiency for hydrolysis of mannans to mannooligosaccharides. Appl Microbiol Biotechnol. 102(23):10027–10041.
- Lutful-Kabir SM. 2009. The role of probiotics in the poultry industry. IJMS. 10(8):3531–3546.
- Manafi M, Hedayati M, Mirzaie S. 2018. Probiotic Bacillus species and Saccharomyces boulardii improve performance, gut histology and immunity in broiler chickens. S Afr J Anim Sci. 48(2):379–388.
- Mingmongkolchai S, Panbangred W. 2018. Bacillus probiotics: an alternative to antibiotics for livestock production-review article. J Appl Microbiol. 124(6):1334–1346.
- Ochangco HS, Gamero A, Smith IM, Christensen JE, Jespersen L, Arneborg N. 2016. In vitro investigation of Debaryomyces hansenii strains for potential probiotic properties. World J Microbiol Biotechnol. 32:1–13.
- Pace DM, Landolt PA, Mussehl FE. 1952. The effect of pigeon crop-milk on growth in chickens. Growth. 16(4):279–285.
- Park S, Li W, Pierre BS, Wang Q, Woyengo TA. 2020. Growth performance, nutrient digestibility and fecal microbial composition of weaned pigs fed multi-enzyme supplemented diets. J Anim Sci. 98:1–31.
- Phuoc TL, Jamikorn U. 2016. Effects of probiotic supplement (Bacillus subtilis and Lactobacillus acidophilus) on feed efficiency, growth performance, and microbial population of weaning rabbits. Asian-Australas J Anim Sci. 30(2):198–205.
- Rajput IR, Li YL, Xu X, Huang Y, Zhi WC, Yu DY, Li W. 2013. Supplementary effects of Saccharomyces boulardii and Bacillus subtilis B10 on digestive enzyme activities, antioxidation capacity and blood homeostasis in broiler. Int J Agric Biol. 15:231–237.
- Raut S, Sen SK, Kabir NA, Satpathy S, Raut S. 2012. Isolation and characterization of protease producing bacteria from upper respiratory tract of wild chicken. Bioinformation. 8(7):326–330.
- Silver R. 1984. Prolactin and parenting in the pigeon family. J Exp Zool. 232(3):617–625.
- Teng PY, Chung CH, Chao YP, Chiang CJ, Chang SC, Yu B, Lee TT. 2017. Administration of Bacillus amyloliquefaciens and Saccharomyces cerevisiae as direct-fed microbials improves intestinal microflora and morphology in broiler chickens. J Poult Sci. 54: 134–141.
- Tsai CF, Lin LJ, Wang CH, Tsai CS, Chang SC, Lee TT. 2021. Assessment of intestinal immunity and permeability of broilers on partial replacement diets of two-stage fermented soybean meal by Bacillus velezensis and Lactobacillus brevis ATCC 367. Animals. 11(8):2336.
- Vink E, Rodriguez-Suarez RJ, Gérard-Vincent M, Ribas JC, de Nobel H, van den Ende H, Durán A, Klis FM, Bussey H. 2004. An in vitro assay for (1-6)-beta-D-glucan synthesis in Saccharomyces cerevisiae. Yeast. 21(13):1121–1131.
- Wang Y, Wu Y, Wang Y, Xu H, Mei X, Yu D, Wang Y, Li W. 2017. Antioxidant properties of probiotic bacteria. Nutrients. 9(5):521–536.
- Yopi Y, Djohan AC, Rahmani N, Jannah AM. 2017. Isolation and characterization of mannanase, xylanase, and cellulase from marine bacteria Bacillus sp. J Asian Nat Prod Res. 15:15–20.