Abstract
Self-propagation of aberrant protein folds is the defining characteristic of prions. Knowing the structural basis of self-propagation is essential to understanding prions and their related diseases. Prion rods are amyloid fibrils, but not all amyloids are prions. Prions have been remarkably intractable to structural studies, so many investigators have preferred to work with peptide fragments, particularly in the case of the mammalian prion protein PrP. We compared the structures of a number of fragments of PrP by X-ray fiber diffraction, and found that although all of the peptides adopted amyloid conformations, only the larger fragments adopted conformations that modeled the complexity of self-propagating prions, and even these fragments did not always adopt the PrP structure. It appears that the relatively complex structure of the prion form of PrP is not accessible to short model peptides, and that self-propagation may be tied to a level of structural complexity unobtainable in simple model systems. The larger fragments of PrP, however, are useful to illustrate the phenomenon of deformed templating (heterogeneous seeding), which has important biological consequences.
Abbreviations
PrP | = | prion protein |
PrP 27–30 | = | proteinase K digested brain-derived PrP |
The ability of some proteins to fold into alternative, β-rich conformations has been known for over 80 yCitation1 Since that time, it has been determined that such β-rich conformations are the defining structural features of amyloid fibrils. The identification of prion rods as amyloid fibrilsCitation2 is more recent, but still well-established. Nevertheless, not all amyloids are prions, indeed, most are not. We must inevitably ask: what are the structural features of prions that distinguish them from other amyloids? What is the structural basis of the protein conformation self-replication that defines prion activity? The answers to these questions have at least 2 important ramifications: conceptually, they will help us to understand the pathological function of prions, and experimentally, they will help us to determine the value of various molecular model systems for prions. The latter is of considerable importance in itself, because of the intractability of most prions to physical and chemical studies.
Because of this intractability, most fiber diffraction studies, all crystallographic studies, and many other structural studies of amyloids have been of small amyloidogenic fragments of biologically important amyloids.Citation3,4 These fragments have the cross-β structure common to all amyloids, β-strands running perpendicular to the fibril axis and forming long β-sheets that extend parallel to the fibril axis. However, the β-sheets are arranged in generic stacked-sheet architectures, stacked parallel to one another. These simple arrangements are quite distinct from those of the structurally more complex architectures found in biologically active amyloids. For example, the fungal prion HET-s and putatively the mammalian prion PrP have β-solenoidal structuresCitation5,6,7 (), and even the relatively small Alzheimer's-associated Aβ peptide has a U-shaped 2-β-strand architecture.Citation8,9 These are cross-β structures, and they are amyloids, but they are qualitatively more complex than simple stacked sheets. Generic stacked-sheet amyloid structures do not appear to share the self-propagating character of structurally more complex prions.
FIGURE 1. Structural models of amyloids, constructed using UCSF-Chimera.Citation31 (A) Four-rung β-solenoidal model core (residues 89–174) of PrP.Citation32 (B) Two-rung β-solenoidal structureCitation6 of HET-s (PDB ID: 2KJ3). (C) Generic antiparallel stacked β-sheet model. (D) Generic parallel stacked-sheet model.
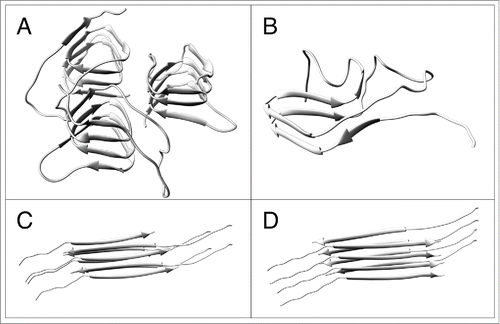
We have recently examinedCitation10 the structures of amyloids formed by a series of fragments of PrP, ranging from the 21-amino-acid fragment PrP21,Citation11 known to form amyloid fibrilsCitation12 and to be toxic but not self-propagating in cell culture,Citation13 to the 106-amino-acid fragment PrPSc106, which has been shown to support prion propagation in transgenic mice.Citation14 We compared these structures to that of PrP 27–30Citation5 (). PrP itself consists of approximately 200 amino acids; PrP 27–30 has about 65 residues removed from the N-terminus, but retains infectivityCitation15 and appears to be structurally very similar to the full-length prion protein PrPSc. We found that the structural similarity between the fragments and PrP 27–30 depends heavily on the size of the fragment. Despite its experimentally demonstrated toxicity, PrP21 has a generic stacked-sheet structure. A 55-residue fragment, PrP55 (mouse PrP(89–143)P101L), may have stacked-sheet or β-solenoidal conformation, depending on conditions of amyloid formation and fiber diffraction specimen preparation, particularly humidity, but even in the β-solenoidal form, the solenoid appears to consist of only 2 rungs of β-strands, not 4 as in PrP 27–30. The diffraction patterns from a larger fragment, PrP89, were under some but not all circumstances consistent with the β-solenoidal structure of PrP 27–30, while the patterns from PrPSc106 were strongly reminiscent of those of PrP 27–30, including meridional maxima at 9.6, 6.4, and 4.8 Å, corresponding to the second, third, and fourth orders of a 19.2-Å repeat, the size of a 4-stranded β-sheet.
We concluded that the relatively complex structure of the prion form of PrP 27–30 is not accessible to short model peptides, although these models can have some value in understanding the simple generic amyloid stacked-sheet structure. Self-propagation, the definitive property of prions, appears to be tied to a level of structural complexity unobtainable in simple model systems, so there may not be simple solutions to the structural understanding of prions.
Amyloids may be considered in an ascending hierarchy of complexity, beginning with the simplest of stacked-sheet structures formed by peptides as short as 6 amino-acid residues, many of which have been studied by crystallographyCitation3 and fiber diffraction.Citation4 Under suitable solution conditions, many, perhaps most, poly-amino acids can form such amyloids. Studies have often been made of small peptide fragments of larger amyloidogenic proteins, but caution should be exercised when considering the amyloid structures of these fragments; it has often been suggested that the amyloid state is accessible to most or all polypeptides under suitable conditions, so there is no reason to assume that the amyloid structure of a fragment is characteristic of the amyloid structure of the complete protein. For example, the β-strands in the structures of short peptide amyloids may be parallel or antiparallel (), although the strands in naturally occurring amyloids have invariably been found to be parallel. The peptide PrP21 formed a 2-strand repeat generic stacked-sheet amyloid, probably with antiparallel strands, under our conditions, although a previous study under nominally identical solution conditions by solid state NMRCitation11 found a single-strand-repeat, parallel in-register stacked sheet. It is certainly possible that different amyloid structures (parallel and antiparallel) could form even under very similar conditions. Regardless of the structural details, there is no evidence that any generic stacked-sheet amyloid structure of this type is self-propagating, as is required for a prion.
Larger polypeptides or proteins can also form generic stacked-sheet amyloids. Infectious recombinant PrPCitation16 forms such structures.Citation5 In most cases recombinant PrP has not been found to be infectious at all, and when it is infectious, it is not clear whether the infectivity is due to a small fraction of molecules, undetected in diffraction experiments but similar in structure to naturally occurring prions, or to a distinctly structured PrP amyloid, which acts as a catalyst for the formation of the fully replication-competent infectious prion structure. This route of infection represents a pathway parallel to the natural etiology and would most likely be caused by a process of deformed templating (heterogeneous seeding).Citation17,18,19
Regardless of the mechanism of infectivity, it is clear that the generic stacked-sheet structure has very little infectivity if any, and is very different, both structurally and functionally, from the β-solenoidal structure. HET-s exhibits a similar polymorphism:Citation20 the wild-type protein at neutral pH and many mutants form β-solenoids, but other mutants and the wild-type protein at acidic pH form non-infectiousCitation21 stacked sheets.
There is, however, a more complex stacked-sheet form, which might be seen as intermediate in structure and complexity between the generic stacked sheet form and the β-solenoidal form. Under low-humidity conditions, PrP55 adopts a different conformation, in which a β-solenoid appears to have collapsed into a stacked-sheet structure. The same effect has been seen for HET-s,Citation22 and may be the basis of a type of structural polymorphism in the yeast prion Sup35.Citation23
The complexity of the PrP β-solenoid is such that even another β-solenoidal structure may not be an adequate model for the full prion structure. PrP55 does under some conditions appear to acquire a 2-rung β-solenoidal structure, and it is infectious in transgenic mice expressing PrP(P101L) at a level similar to that of wild-type PrP.Citation24,25 Nevertheless, it is too small to form the full 4-rung β-solenoid, and in this sense, can only be a partial model for PrPSc. PrPSc106 forms a 4-rung β-solenoid, and is also infectious,Citation14 and of the fragments examined, it may be the best model for PrPSc. However, both PrP55 and PrPSc106 exhibit high levels of disorder, and PrP55 does not consistently form β-solenoidal structures, so these fragment models offer limited advantages if any over PrPSc and PrP 27–30 as objects of structural studies.
In a further extension of complexity, there is considerable evidence that prion structure and activity is affected not only by the amyloid structure of the folded protein, but by other parts of the protein, and in particular by the loops at the ends of and often connecting the β-strands. While there is no structure available in sufficient detail to illustrate this point for PrP, it is evident in the cases of Aβ and HET-s. Mutations in a region without β-structure, the connecting loop between the 2 rungs of the β-solenoidal structure of HET-s (), can have a large impact on fibrillization kinetics, stability, and structure of the fibril,Citation20 as well as infectivity.Citation26 In Aβ, residues 21–23 in the boundary between the first β-strand and the loop connecting it to the second strand are often altered in families exhibiting early-onset Alzheimer's disease.Citation27
Perhaps the greatest value for prion studies of at least the larger fragments of PrP lies in their use not as structural models for the active prion itself, but as templates that illustrate the phenomenon of heterogeneous seeding or deformed templating.Citation17,18,19,28 The infectivity of PrP55, for example, may well come from heterogeneous seeding, that is, seeding between distinct amyloid architectures. With two rungs of a β-solenoid, PrP55 could easily contain a structured segment that can act as a seed, in distinct contrast to PrP21 and other short, non-infectious fragments. Such seed structures would usually need to contain elements of the PrPSc structure, and to that extent could represent partial structural models, although they do not contain all of the important molecular interactions found in PrPSc. Even more significantly, however, the phenomenon of seeding itself has important biological consequences.
The complexity of prion structures can allow a variety of amyloid structures to form, and some of these structures can give rise to prion strains, having different phenotypes. For example, behavioral variation has been observed among PrP strains.Citation29 The molecular basis of differences among strains is now widely considered to be structural variation. Although self-propagation of molecular conformation in prions usually faithfully replicates the original prion structure, prions may occasionally act as seeds to form different structures. This is the process of deformed templating, and has been demonstrated in HET-sCitation19,28 and PrP.Citation17 Amyloids with distinct architectures can interact with one another, without requiring in vivo cofactors. Deformed templating provides a mechanism for a preferred prion structure to be reached from different initial nucleating agents, although the process may require several passages through animals.Citation17,30
In summary, small fragments of prion proteins have at best limited value as structural models, but infectious fragments, which have at least until now only included fragments of considerable size such as PrP55, may well have value in heterogeneous seeding or deformed templating studies of prion strains, strain adaptation, and interspecies prion transmission.
Disclosure of Potential Conflicts of Interest
No potential conflicts of interest were disclosed.
Funding
This work was supported by U.S. National Institutes of Health (NIH) grants AG002132, AG021601, AG010770, and F31-AG040947. The SSRL is a national user facility operated by Stanford University on behalf of the DOE. The SSRL Structural Molecular Biology Program is supported by the DOE and NIH. The APS is supported by the DOE. BioCAT is supported by NIH grant 9 P41 GM103622.
REFERENCES
- Astbury WT, Dickinson S, Bailey K. The X-ray interpretation of denaturation and the structure of the seed globulins. Biochem J 1935; 29:2351-60; PMID:16745914; http://dx.doi.org/10.1042/bj0292351
- Prusiner SB, McKinley MP, Bowman KA, Bolton DC, Bendheim PE, Groth DF, Glenner GG. Scrapie prions aggregate to form amyloid-like birefringent rods. Cell 1983; 35:349-58; PMID:6418385; http://dx.doi.org/10.1016/0092-8674(83)90168-X
- Sawaya MR, Sambashivan S, Nelson R, Ivanova MI, Sievers SA, Apostol MI, Thompson MJ, Balbirnie M, Wiltzius JJW, McFarlane HT, Madsen AØ, Riekel C, Eisenberg D. Atomic structures of amyloid cross-β spines reveal varied steric zippers. Nature 2007; 447:453-7; PMID:17468747; http://dx.doi.org/10.1038/nature05695
- Jahn TR, Makin OS, Morris KL, Marshall KE, Tian P, Sikorski P, Serpell LC. The common architecture of cross-β amyloid. J Mol Biol 2010; 395:717-27; PMID:19781557; http://dx.doi.org/10.1016/j.jmb.2009.09.039
- Wille H, Bian W, McDonald M, Kendall A, Colby DW, Bloch L, Ollesch J, Borovinskiy, AL, Cohen FE, Prusiner SB, Stubbs G. Natural and synthetic prion structure from X-ray fiber diffraction. Proc Natl Acad Sci USA 2009; 106:16990-5; PMID:19805070; http://dx.doi.org/10.1073/pnas.0909006106
- Van Melckebeke H, Wasmer C, Lange A, Ab E, Loquet A, Böckmann A, Meier BH. Atomic-resolution three-dimensional structure of HET-s(218−289) amyloid fibrils by solid-state NMR spectroscopy. J Am Chem Soc 2010; 132:13765-75; PMID:20828131; http://dx.doi.org/10.1021/ja104213j
- Wan W, Wille H, Stöhr J, Baxa U, Prusiner SB, Stubbs G. Degradation of fungal prion HET-s(218-289) induces formation of a generic amyloid fold. Biophys J 2012; 102:2339-44; PMID:22677387; http://dx.doi.org/10.1016/j.bpj.2012.04.011
- McDonald M, Box H, Bian W, Kendall A, Tycko R, Stubbs G. Fiber diffraction data indicate a hollow core for the Alzheimer's Aβ 3-fold symmetric fibril. J Mol Biol 2012; 423:454-61; PMID:22903058; http://dx.doi.org/10.1016/j.jmb.2012.08.004
- Lu J, Qiang W, Yau W, Schwieters CD, Meredith SC, Tycko R. Molecular structure of β-amyloid fibrils in Alzheimer's disease brain tissue. Cell 2013; 154:1257-68; PMID:24034249; http://dx.doi.org/10.1016/j.cell.2013.08.035
- Wan W, Wille H, Stöhr J, Kendall A, Bian W, McDonald M, Tiggelaar S, Watts JC, Prusiner SB, Stubbs G. Structural studies of truncated forms of the prion protein PrP. Biophys J 2015; 108(6):1548-54; PMID:25809267; http://dx.doi.org/10.1016/j.bpj.2015.01.008
- Walsh P, Simonetti K, Sharpe S. Core structure of amyloid fibrils formed by residues 106–126 of the human prion protein. Structure 2009; 17:417-26; PMID:19278656; http://dx.doi.org/10.1016/j.str.2008.12.018
- Tagliavini F, Prelli F, Verga L, Giaccone G, Sarma R, Gorevic P, Ghetti B, Passerini F, Ghibaudi E, Forloni G, Salmona M, Bugiani O, Frangione B. Synthetic peptides homologous to prion protein residues 106–147 form amyloid-like fibrils in vitro. Proc Natl Acad Sci USA 1993; 90:9678-82; PMID:8105481; http://dx.doi.org/10.1073/pnas.90.20.9678
- Forloni G, Angeretti N, Chiesa R, Monzani E, Salmona M, Bugiani O, Tagliavini F. Neurotoxicity of a prion protein fragment. Nature 1993; 362:543-6; PMID:8464494; http://dx.doi.org/10.1038/362543a0
- Supattapone S, Bosque P, Muramoto T, Wille H, Aagaard C, Peretz D, Nguyen HO, Heinrich C, Torchia M, Safar J, Cohen FE, DeArmond SJ, Prusiner SB, Scott M. Prion protein of 106 residues creates an artificial transmission barrier for prion replication in transgenic mice. Cell 1999; 96:869-78; PMID:10102274; http://dx.doi.org/10.1016/S0092-8674(00)80596-6
- McKinley MP, Meyer RK, Kenaga L, Rahbar F, Cotter R, Serban A, Prusiner SB. Scrapie prion rod formation in vitro requires both detergent extraction and limited proteolysis. J Virol 1991; 65:1340-51; PMID:1704926
- Legname G, Baskakov IV, Nguyen H-OB, Riesner D, Cohen FE, DeArmond SJ, Prusiner SB. Synthetic mammalian prions. Science 2004; 305:673-6; PMID:15286374; http://dx.doi.org/10.1126/science.1100195
- Makarava N, Kovacs GG, Savtchenko R, Alexeeva I, Budka H, Rohwer RG, Baskakov IV. Stabilization of a prion strain of synthetic origin requires multiple serial passages. J Biol Chem 2012; 287:30205-14; PMID:22807452; http://dx.doi.org/10.1074/jbc.M112.392985
- Makarava N, Kovacs GG, Savtchenko R, Alexeeva I, Ostapchenko, VG, Budka H, Rohwer RG, Baskakov IV. A new mechanism for transmissible prion diseases. J Neurosci 2012; 32:7345-55; PMID:22623680; http://dx.doi.org/10.1523/JNEUROSCI.6351-11.2012
- Wan W, Bian W, McDonald M, Kijac A, Wemmer DE, Stubbs G. Heterogeneous seeding of a prion structure by a generic amyloid form of the fungal prion-forming domain HET-s(218–289). J Biol Chem 2013; 288:29604-12; PMID:23986444; http://dx.doi.org/10.1074/jbc.M113.505511
- Wan W, Stubbs G. Fungal prion HET-s as a model for structural complexity and self-propagation in prions. Proc Natl Acad Sci USA 2014; 111:5201-6; PMID: 24706820; http://dx.doi.org/10.1073/pnas.1322933111
- Sabaté R, Baxa U, Benkemoun L, Sánchez de Groot N, Coulary-Salin B, Maddelein M, Malato L, Ventura S, Steven AC, Saupe SJ. Prion and non-prion amyloids of the HET-s prion forming domain. J Mol Biol 2007; 370:768-83; http://dx.doi.org/10.1016/j.jmb.2007.05.014
- Wan W, Stubbs G. Fiber diffraction of the prion-forming domain HET-s(218–289) shows dehydration-induced deformation of a complex amyloid structure. Biochemistry 2014; 53:2366-70; PMID:24670041; http://dx.doi.org/10.1021/bi5002807
- McDonald M, Kendall A, Tanaka M, Weissman JS, Stubbs G. Enclosed chambers for humidity control and sample containment in fiber diffraction. J Appl Cryst 2008; 41:206-9; http://dx.doi.org/10.1107/S0021889807060803
- Kaneko K, Ball HL, Wille H, Zhang H, Groth D, Torchia M, Tremblay P, Safar J, Prusiner SB, DeArmond SJ, Baldwin MA, Cohen FE. A synthetic peptide initiates Gerstmann-Sträussler-Scheinker (GSS) disease in transgenic mice. J Mol Biol 2000; 295:997-1007; PMID:10656806; http://dx.doi.org/10.1006/jmbi.1999.3386
- Tremblay P, Ball HL, Kaneko K, Groth D, Hegde RS, Cohen FE, DeArmond SJ, Prusiner SB, Safar JG. Mutant PrPSc conformers induced by a synthetic peptide and several prion strains. J Virol 2004; 78:2088-99; PMID:14747574; http://dx.doi.org/10.1128/JVI.78.4.2088-2099.2004
- Ritter C, Maddelein ML, Siemer AB, Lührs T, Ernst M, Meier BH, Saupe SJ, Riek R. Correlation of structural elements and infectivity of the HET-s prion. Nature 2005; 435:844-8; PMID:15944710; http://dx.doi.org/10.1038/nature03793
- Schledorn M, Meier B, Böckmann A. Alternative salt bridge formation in Aβ - a hallmark of early-onset Alzheimer disease? Front Mol Biosci 2015; 2:14; PMID:25988181
- Wan W, Stubbs G. Heterogeneous seeding of HET-s(218-289) and the mutability of prion structures. Prion 2014; 8:1-5; PMID:25077317; http://dx.doi.org/10.4161/pri.28126
- Prusiner SB. Prions. In: Knipe DM, Howley PM, Griffin DE, eds., Fields Virology. Philadelphia, PA: Lippincott Williams & Wilkins; 2007; 3059-92.
- Ghaemmaghami S, Colby DW, Nguyen HO, Hayashi S, Oehler A, DeArmond SJ, Prusiner SB. Convergent replication of mouse synthetic prion strains. Am J Pathol 2013; 182:866-74; PMID:23438476; http://dx.doi.org/10.1016/j.ajpath.2012.11.038
- Pettersen EF, Goddard TD, Huang CC, Couch GS, Greenblatt DM, Meng EC, Ferrin TE. UCSF Chimera - a visualization system for exploratory research and analysis. J Comput Chem 2004; 25:1605-12; PMID:15264254; http://dx.doi.org/10.1002/jcc.20084
- Govaerts C, Wille H, Prusiner SB, Cohen FE. Evidence for assembly of prions with left-handed β-helices into trimers. Proc Natl Acad Sci USA 2004; 101:8342-7; PMID:15155909; http://dx.doi.org/10.1073/pnas.0402254101